39 Surgical site infection and antimicrobial prophylaxis
Epidemiology
In England, there are over nine million operations and interventions undertaken each year. Over 50% of these are performed as day cases and many patients are admitted on the day of surgery (Health and Social Care Information Centre, 2009). Healthcare-associated infections (HCAIs), including surgical site infections, complicate around 7% of all hospital admissions (HIS/ICNA, 2007). Surgical site infections are of major clinical importance because they account for 14–16% of all healthcare-associated infections (HPS, 2009; Public Accounts Committee, 2009) and are associated with considerable morbidity and mortality. One-third of peri-operative deaths are related to surgical site infections (Astagneau et al., 2001). It has been estimated that surgical site infections double the length of hospital stay (Coello et al., 2005). While surgical site infections can be common in some procedures, the incidence can be minimised by the care provided before and after the operation, together with the skill of the surgeon (HPS, 2009).
Surveillance
Monitoring the incidence of surgical site infections is hampered by the lack of agreed measuring systems. In particular, to monitor the rates of surgical site infection within an organisation, or to benchmark between organisations, there needs to be a standard approach to diagnosis. Criteria for such a definition have been developed by the Centres for Disease Control and Prevention (CDC) (Mangram et al., 1999) and these are presented in Table 39.1. More detailed surgical site infection scoring systems have been developed but these are time consuming to use.
Table 39.1 Criteria for defining surgical site infection (Mangram et al., 1999)
Type | Level | Signs and symptoms |
---|---|---|
Superficial incisional | Skin and subcutaneous tissue | Localised (Celsian) signs such as redness, pain, heat or swelling at the site of the incision or by the presence of pus within 30 days |
Deep incisional | Fascial and muscle layers | Presence of pus or an abscess, fever with tenderness of the wound, or a separation of the edges of the incision exposing the deeper tissues within 30 days (or 1 year if an implant used) |
Organ or space infection | Any part of the anatomy other than the incision that is opened or manipulated during the surgical procedure, for example, joint or peritoneum | Loss of function of a joint, abscess in an organ, localised peritonitis or collection. Ultrasound or CT scans confirm infection. Within 30 days (or 1 year if implant is used) |
Mandatory surveillance for surgical site infections in orthopaedic surgery in the UK was introduced in 2003. In addition, Scotland monitors most other common procedures (http://www.hps.scot.nhs.uk) while in Wales caesarian section is also monitored (http://www.wales.nhs.uk). England has a voluntary reporting system for a broader range of operations (http://www.hpa.org.uk). All report their findings annually. Many surgical site infections, for example, those involving prosthetic joints, often develop late (>28 days post-operation), so post-discharge surveillance schemes are essential. Patients need to be aware how a surgical site infection may present after discharge from hospital. Surveillance of surgical site infections and feedback to the surgical team has been shown to reduce rates of infection (Gastmeier et al., 2005).
Risk factors
Surgical site infections can be categorised into three groups: superficial incisional, deep incisional and organ or space (Fig. 39.1) Whether a wound infection occurs after surgery depends on a complex interaction between the following:
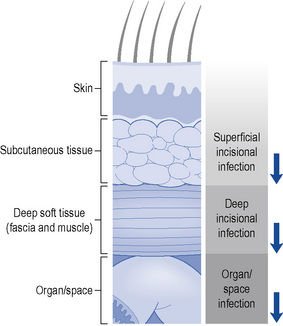
Fig. 39.1 Schematic representation of the anatomical classification of surgical site infection
(Horan et al., 1992). Reproduced with permission from the University of Chicago Press.
A system to stratify operative wounds by the expected level of bacterial contamination (Table 39.2) was developed to help predict likely infection rates (Mangram et al., 1999). A number of other factors have also been found to affect the incidence of surgical site infection and are discussed below.
Prosthetic implants
Medical implants have a detrimental effect on host defences such that a lower bacterial count is needed to initiate infection. Hence, there is a greater risk of infection during implant surgery. Bacteria growing on an abiotic surface, such as a prosthetic hip implant or heart valve, together with a protective layer of microbial polymers are known as a biofilm (Donlan and Costerton, 2002). Antimicrobials are frequently ineffective against micro-organisms growing in biofilms, making treatment of implant infections problematic and their prevention even more important.
Duration of surgery
The longer the operation, the greater is the risk of wound infection. This, in turn, may be influenced by the experience (Fig. 39.2) speed and skill of the surgeon and is additional to the classification of the operation by risk of infection, for example, clean, contaminated, dirty or infected.
Patient related factors
Table 39.3 American Society of Anesthesiology (ASA) classification of physical status (Mangram et al., 1999)
ASA score | Physical status |
---|---|
1 | A normal healthy patient |
2 | A patient with mild systemic disease |
3 | A patient with a severe systemic disease that limits activity but is not incapacitating |
4 | A patient with an incapacitating systemic disease that is a constant threat to life |
5 | A moribund patient that is not expected to survive 24 h with or without operation |
For each surgical procedure, a score of 0–3 is allocated to represent the number of risk factors present. Patients with a score of 0 are at the lowest risk of developing a surgical site infection, while those with a score of 3 have the greatest risk (Table 39.4). Use of this risk index allows comparison of similar patient groups in terms of surgical site infection risk over time. The risk index is a significantly better predictor of surgical-wound risk than the traditional wound classification system and performs well across a broad range of operative procedures.
Table 39.4 Risk index based on presence of co-morbidity and duration of operation (Culver et al., 1991)
Risk index | Infection rate (%) |
---|---|
0 | 1.5 |
1 | 2.9 |
2 | 6.8 |
3 | 13.0 |
Other factors
There are a number of other risk factors that may increase the risk of a surgical site infection (Table 39.5) for an individual patient but the impact has not been quantified to the extent of those risk factors discussed above.
Table 39.5 Patient and operative risk factors for surgical site infection
Patient risk factors | Operative risk factors |
---|---|
Advanced age | Tissue ischaemia |
Malnutrition | Lack of haemostasis |
Obesity | Tissue damage, for example, crushing by surgical instruments |
Concurrent infection | Presence of necrotic tissue |
Diabetes mellitus | Presence of foreign bodies including surgical materials |
Liver impairment | |
Renal impairment | |
Immune deficiency states | |
Prolonged preoperative stay | |
Blood transfusion | |
Smoking |
Smoking
Smoking increases the risk of developing a wound infection (Myles et al., 2002). The mechanism is not known but tobacco use may delay wound healing via the vasoconstricting effects of nicotine and thus increase the risk of infection (Myles et al., 2002).
Diabetes mellitus
Long-term diabetes does not appear to have any impact on the risk of developing a surgical site infection. However, peri-operative fluctuations in blood glucose for up to 48 h have been shown to double the infection risk in cardiac patients (Latham et al., 2001).
Age
Increasing age is associated with an increased risk of surgical site infection. However, there is debate whether age serves simply as a marker for underlying disease or whether the decline in immune function with age is the significant factor. A study of 72,000 patients in the USA, which adjusted for hospital type, procedure, duration, wound class and physical status of the patient, showed a 1.1% increase in surgical site infection per year of age from the age of 18 to 65 years, but a 1.2% decrease in individuals over 65 years (Kaye et al., 2005). In contrast, the findings of the English surgical site infection surveillance scheme (Fig. 39.3) indicated that the chance of getting a surgical site infection were 37% higher for a 65-year-old person compared to a 45-year-old person (HPA, 2008).
Pathogenesis
Development of a surgical site infection depends on survival of the contaminating micro-organism in a wound site at the end of a surgical procedure; the pathogenicity and number of these micro-organisms; and the host’s immune response. Most micro-organisms are from the host (endogenous), but are occasionally introduced via surgical instruments, the environment or contaminated implants (exogenous). The likely invading micro-organism varies according to the type of surgery (Table 39.6). Data for England from 2003 to 2007 has shown that the predominant organism was Staphylococcus aureus, which accounted for 38% of all surgical site infections (Fig. 39.4); 64% of these were caused by a meticillin-resistant strain (MRSA). The proportion of surgical site infections caused by S. aureus was highest in hip hemiarthroplasty (57%), followed by limb amputation (54%) and open reduction of long bone fracture (52%). Enterobacteriaceae (coliforms) caused the second largest group of infections, accounting for 21% of all surgical site infections. These were the prominent causes of surgical site infections in three categories: large bowel surgery (33%), coronary artery bypass graft (32%) and small bowel surgery (30%).
Table 39.6 Likely pathogens in post-operative wound infections
Category of surgery | Most likely pathogen(s) |
---|---|
Clean | |
Cardiac/vascular/orthopaedic Breast | Coagulase-negative staphylococci, S. aureus, Gram-negative bacilliS. aureus |
Clean-contaminated | |
Burns | S. aureus, Pseudomonas aeruginosa |
Head and neck Gastro-intestinal tract | S. aureus, Streptococcus spp., anaerobes (from oral cavity)Coliforms, anaerobes (Bacteroides fragilis) |
Urogenital tract | Coliforms, Enterococcus spp. |
Dirty | |
Ruptured viscera | Coliforms, anaerobes (B. fragilis) |
Traumatic wound | S. aureusStreptococcus pyogenes, Clostridium spp. |