CHAPTER 61 Surgery for Extratemporal Lobe Epilepsy
One of the most significant developments in the treatment of epilepsy has been the recognition of specific surgically remediable syndromes of epilepsy.1 Foremost among these conditions has been the syndrome of mesial temporal lobe epilepsy, characterized by distinct patterns of semiology, electroencephalographic signature, imaging correlates, and histopathology.2 The hallmark of this syndrome is hippocampal sclerosis, which underlies a hyperexcitable, recurrent, and pharmacologically resistant pattern of electrical activity. From the surgeon’s standpoint, the significance of this syndrome is the feasibility of a uniform surgical approach to the disease.
Although mesial temporal lobe epilepsy has become better defined as a clinicopathologic entity that can be treated with a standard surgical approach,2,3 such is not the case for extratemporal lobe epilepsy syndromes. The semiology of extratemporal neocortical epilepsy is less well characterized, even when the seizure focus is localized to a single lobe (frontal, temporal, or parietal). Extratemporal lobe epilepsies also tend to spread rapidly, thus making localization based on their clinical characteristics difficult. In some cases, especially in patients with frontal lobe epilepsy, seizures cross to the contralateral side rapidly, which makes it difficult to even lateralize the site of seizure onset.
The presence of a lesion on preoperative imaging studies has a significant impact on the surgical prognosis. Seizure-free outcomes after lesional extratemporal epilepsy surgery are significantly better than those after nonlesional epilepsy surgery.4 MRI scanners with higher magnet strength that can provide brain imaging with higher resolution offer promise for identifying anatomic abnormalities in more patients,5,6 an especially promising area because many surgical specimens from “nonlesional” epilepsy surgery are found to have abnormalities on subsequent pathologic analysis.7 New MRI and other imaging technologies that are being developed for the identification of epileptic foci are discussed later in the chapter in the section on patient evaluation.
Technologic advances have provided modern alternatives to resective surgery for medically intractable epilepsy, but none has supplanted surgical resection in efficacy. These alternatives include stimulation methods, such as vagal nerve stimulation or deep brain stimulation (DBS). The rate of significant seizure control with vagal nerve stimulation is approximately 30% to 50%.8 DBS for severe intractable epilepsy is still largely experimental, and even more recent protocols using stimulation of the subthalamic nucleus (STN) or anterior thalamus have achieved only a modest rate of success.9–11 Technologic advances have also made resective surgery safer. Advances in neurosurgical techniques and neuroanesthesia have made operative mortality a rare occurrence. Advances in functional imaging, stimulation brain mapping, and intraoperative image guidance help minimize the chance for neurological deficit. These considerations require that the surgical team have a good understanding of the different extratemporal seizure syndromes and the surgical risks and seizure control rates for each so that discussion among the surgical team members and with the patient can lead to an optimal decision.
General Epilepsy Surgery Outcomes
The earliest epilepsy surgeries performed in the late 1800s and early 1900s were targeted toward neocortical seizure foci.12 Because of the limited utility of imaging and neurophysiologic modalities available to clinicians at that time, localization of the seizure focus was based primarily on anatomic features such as external signs of head trauma and underlying cortical encephalomalacia. Over time, improved imaging and electrophysiologic evaluation allowed delineation of medial temporal lobe epilepsy syndrome,13,14 which is characterized by hippocampal sclerosis.15,16 The work of several surgeons in developing and analyzing surgical approaches to this syndrome17–21 has led to standardized approaches to epilepsy surgery for these patients. With these standardized approaches and distinct imaging characteristics of hippocampal sclerosis, many epilepsy surgery centers have proposed protocols that allow medial temporal lobe epilepsy surgery to be performed with a defined evaluation.22 In addition, epilepsy surgery for medial temporal lobe epilepsy with hippocampal sclerosis is associated with a high seizure-free outcome rate on the order of 53% to 84%, a rate that has not been matched by extratemporal neocortical resections.23–26 Probably for these and other reasons discussed later, modern series evaluating epilepsy surgery show that extratemporal lobe epilepsy surgery is much less common than temporal lobe epilepsy surgery.
The most recent multicenter source of information regarding current practice patterns of major epilepsy centers comes from a seven-center prospective observational study of resective epilepsy surgery. In this study, which included 355 patients at 1-year follow-up and 339 patients at 2-year follow-up, only 12% of the epilepsy surgery patients underwent extratemporal surgery.27,28 In a review of 708 epilepsy surgeries at a major epilepsy surgery center in Germany, 429 of which were therapeutic, 35% of the therapeutic surgeries were extratemporal and consisted of frontal resection in 14%, parietal resection in 2%, occipital resection in 3%, multilobar resection in less than 1%, callosotomy in 8%, and hemispherectomy in 8%.29 Based on these and other studies, it is clear that temporal lobe epilepsy surgery, especially medial temporal lobectomy, is much more common than extratemporal lobe epilepsy surgery. There are several probable reasons for this discrepancy, including a higher incidence of medial temporal lobe epilepsy that is intractable, better seizure-free rates with medial temporal lobe resection than with extratemporal resection, and frequently, more diffuse or obscure extratemporal seizure foci necessitating a more tailored approach for extratemporal resection and requiring additional evaluation for determination of the exact seizure location, often with surgically implanted electrodes.
Outcomes of extratemporal lobe epilepsy surgery are based mostly on retrospective case series reviews. Only one study has provided class I evidence for epilepsy surgery; however, this study looked only at medial temporal lobe resections but demonstrated a clear benefit of early surgery over maximal medical therapy for medically intractable epilepsy.25 The multicenter epilepsy surgery study just mentioned was a prospective case series that grouped neocortical temporal with general extratemporal cases.27,28 This study found that patients undergoing neocortical resection had a lower seizure-free rate than did patients undergoing medial temporal lobe resection at 1-year follow-up (56% for neocortical versus 77% for medial temporal resection) and 2-year follow-up (50% for neocortical versus 68% for medial temporal resection). These differences were statistically significant only at the 1-year follow-up. Interestingly, the seizure relapse rate for patients who were initially seizure free after surgery was lower with neocortical resection than with medial temporal lobe resection in both the 1-year (4% for neocortical versus 24% for medial temporal resection) and 2-year studies (19% for neocortical versus 25% for medial temporal resection), again only statistically significant at 1 year. A recent review of the adult and pediatric epilepsy surgery literature reported 1-year or greater freedom from seizures in 53% to 84% of patients undergoing medial temporal lobe resection, in 66% to 100% of patients with dual pathology, including medial temporal sclerosis and temporal neocortical involvement, and in 36% to 76% of patients undergoing neocortical resection.23
The American Academy of Neurology in association with the American Epilepsy Society and the American Association of Neurological Surgeons published a position paper that reviewed all the outcome studies for epilepsy surgery before the paper’s publication in 2003.30 The Quality Standards Subcommittee identified 33 studies reporting seizure-free outcomes after epilepsy surgery, but just 1 class I study was included (the one discussed earlier) and 32 class IV studies. Only 8 of these studies described outcomes after neocortical resection, including both temporal and extratemporal locations, and all were class IV studies. This group was able to conclude that the benefits of medial temporal lobe resection surgery for medically intractable disabling complex partial seizures are greater than the benefits with continued maximal medical therapy and that the risks related to surgery are at least comparable to the risks associated with antiepileptic drugs. However, they were not able to draw similar conclusions from the neocortical resection studies because of the low number of studies, lack of class I evidence, and great variability among the different neocortical epilepsies and their surgeries based on the lobe involved. This position paper simply states that further studies are needed to determine the benefits of surgery for treating neocortical epilepsies.
Many studies have supported the overall benefit of successful epilepsy surgery in several areas of patients’ daily lives. These studies have not typically differentiated among patients according to the site of the resection, but it is likely that these quality-of-life studies are applicable to all patients who have successfully undergone epilepsy surgery, including surgery on the extratemporal lobe. The Multicenter Study of Epilepsy Surgery looked at some quality-of-life outcomes in their study patients. They found that quality of life was improved by 3 months after surgery regardless of seizure outcome but that scores at 1 and 2 years were statistically significantly lower in patients who were not seizure free than in those who were seizure free.27 Others have found improved scores on quality-of-life measures after epilepsy surgery.31 A study of pediatric epilepsy surgery also found that quality of life was improved to a greater degree in children rendered seizure free after surgery than in those who continued to have seizures.32 Both anxiety and depression decreased significantly by 3 months after surgery, more in the seizure-free patients than in those who continued to have seizures, and scores remained improved at 1 and 2 years’ follow-up.33 Full-scale IQ has also shown improvements in long-term follow-up after temporal, parietotemporal, and frontal epilepsy surgery.34 At 2 years after surgery, 75.5% of patients said that they would definitely undergo surgery again, 79.1% thought that they had a very strong or strong overall positive impact from the surgery, but in only 7% was employment status improved.35 Other studies have shown no improvement in employment status for epilepsy surgery patients in comparison to epilepsy patients without surgical treatment despite a greater decline in the former group in both monthly seizure frequency and antiepileptic medication intake.31 In a review of epilepsy surgery outcome papers, two studies evaluated multiple outcome factors in both temporal and extratemporal epilepsy surgery. Both studies found improvements in seizure outcome and decreased antiepileptic drug use, but only one of these studies found an improvement in social outcomes, and the one study that evaluated quality of life found no difference between surgical patients and controls.24 This paper reported that in studies in which temporal lobe and extratemporal lobe resections were analyzed together, an average of 20% of patients were able to discontinue their antiepileptic drugs and an average of 41% were able to achieve monotherapy. Adverse outcomes associated with epilepsy surgery are relatively infrequent, with a series of 429 therapeutic epilepsy surgeries reporting no mortality, transient morbidity in 3%, and permanent morbidity in 2.3%.29 Taken together, these results indicate a positive impact of epilepsy surgery on patients’ lives that outweighs the risks.
Lobar Distribution of Extratemporal Lobe Epilepsy
Several case series have looked at epilepsy surgery in general or extratemporal epilepsy surgery in particular. These studies give an idea of the relative incidence of surgically remediable epilepsy in each of the lobes and the frequency of types of extratemporal epilepsy surgery. In adult epilepsy surgery series, extratemporal surgery represents 13% to 37% of operations.36–38 Of the extratemporal resections, 60% to 84% were frontal, 4% to 20% were parietal, 3% to 20% were occipital, and 0% to 46% were multilobar.36,38 Series combining adult and pediatric patients have reported extratemporal surgery in 12% to 44%.27–29,36,38–41 Of these, 33% to 64% were frontal, 7% to 14% were parietal, 2% to 23% were occipital, 0% to 37% were multilobar, 0% to 34% involved corpus callosotomy, and 0% to 22% involved hemispherectomy.29,36,38–44 The percentage of extratemporal epilepsy surgery cases in pediatric series varies from 32% to 83%.36,38,45–52 The pediatric extratemporal epilepsy surgeries included frontal resection in 18% to 73%, parietal in 0% to 18%, occipital in 0% to 9%, multilobar in 0% to 72%, corpus callosotomy in 0% to 50%, and hemispherectomy in 0% to 52%.36,38,45–52 Extratemporal resections, including corpus callosotomy and hemispherectomy, are more common in the pediatric population.
Parietal lobe epilepsy and occipital lobe epilepsy are rare in surgical series, a finding reported by several authors.53,54 This very low incidence may be due to the relative resistance of these regions of the brain to the development of seizures, difficulty identifying these seizures because of the ambiguity of symptoms referable to seizures from these areas (especially in parietal lobe epilepsy), and the potential risk for permanent postoperative neurological deficit. The data also illustrate one of the characteristics of extratemporal lobe epilepsy that make it less amenable than temporal lobe epilepsy to surgical therapy—a higher incidence of widespread pathology involving more than one lobe.
Frontal Lobe Epilepsy
The frontal lobe is the largest lobe of the brain, and it encompasses several distinct anatomic-functional units, including the primary motor region; supplementary motor areas; language areas in the dominant frontal operculum; the frontal eye fields; part of the cingulate gyrus; a component of the limbic system; the orbitofrontal and ventromedial regions, which play a major role in the regulation of emotions; and the dorsolateral frontal region, which has major cognitive function, especially in executive functions and working memory. The regions responsible for clearly observable motor functions, the primary and supplementary motor areas, were the earliest to be classified anatomically55,56 and the earliest targets for surgical treatment of epilepsy.57–60 The clinical syndromes of primary and secondary motor cortex seizures are fairly well agreed on. Characterization of primary motor cortex seizures has remained essentially unaltered since the investigations of Penfield and Jasper12: focal clonic jerks without loss of consciousness if generalization does not occur. Supplementary motor cortex seizure morphology has been described by Ajmone-Marsan and Ralston61 and later investigators and has been characterized by more complex motor semiology, including combined movements of the extremities and head version.
Seizures in other regions of the frontal lobe have shown significant variability, which has resulted in difficulty characterizing classic frontal lobe epilepsy syndromes.62 Based on characterization of seizures in patients via depth electrode recording, Bancaud and Talairach63 proposed that frontal lobe epilepsies be classified into (1) inferior frontal gyrus seizures in either the dominant or nondominant hemisphere with speech arrest, tonic or tonic-clonic contractions at the ipsilateral angle of the mouth, swallowing, salivation, gustatory hallucinations, vegetative signs, respiratory deficits, and possibly simple motor manifestations; (2) medial intermediate frontal seizures originating in the mesial frontal lobe, anterior to the supplementary motor cortex, superior to the cingulate gyrus, and posterior to the polar region, with frontal-type absence or complex motor seizures; (3) dorsolateral intermediate frontal seizures with contralateral deviation of the eyes followed by aversion of the head; (4) anterior cingulate gyrus seizures with intense fright, expressions of fear, and aggressive verbalizations and acts; (5) frontopolar seizures with dissociation from the environment, fixed eyes, immobility, flexion and turning of the head, falling, and tonic-clonic generalization; (6) orbitofrontal seizures with either olfactory illusions and hallucinations or vegetative symptoms, including cardiovascular, respiratory, or digestive system involvement; and (7) operculoinsular suprasylvian seizures with a variety of symptoms, including somatomotor involvement of the face and upper and lower limbs, disorders of verbal expression, contralateral oculocephalic deviation, dissociation from the environment, and postictal speech deficits. These syndromes are not universally accepted, with some authors expressing skepticism concerning the feasibility of anatomic localization by seizure semiology.64
Localization of a single resectable focus in patients with frontal lobe epilepsy is typically difficult because of the propensity for extensive epileptogenic zones with multiple pathways that allow rapid ictal spread within the frontal lobe and to other lobes and the contralateral side.65 Even a determination of lateralization can be difficult.66 In the absence of a distinct focal structural lesion, localization of frontal lobe epilepsy foci almost always requires intracranial ictal recording with either subdural electrodes65 or depth electrodes.63 The findings from these evaluations, combined with the variety of anatomic imaging modalities available, are the basis on which surgical resection plans are made.
Because of the difficulty in localization of the seizure focus, it is not surprising that reports of a reduction in seizure episodes after surgery for frontal lobe epilepsy are fewer than for temporal lobe seizures. An additional factor may be increased restraint in performing cortical resection because of the relative involvement of critical functions in the frontal lobe.67,68 Generous resections of the frontal lobe for epilepsy have been described, including total frontal lobectomy on the nondominant side and resection of the lateral sensorimotor cortex to nearly 3 cm above the sylvian fissure.41,69,70 Any transient deficit from loss of a frontal eye field or the supplementary motor cortex is likely to resolve eventually. Special care is taken, however, to preserve speech areas in the frontal operculum of the dominant hemisphere, a region in which incurred deficits are likely to remain permanent. Care is exercised in resecting primary motor cortex, especially in the hand region or in the dominant hemisphere face region, because fine motor movement could be permanently affected.12,57,71 Functional MRI (fMRI) can be helpful in delineating these motor areas preoperatively.72 A recently developed MRI technique, diffusion-tensor imaging (DTI), enables delineation of the subcortical motor pathways.73
Frontal lobe epilepsy surgery is somewhat successful in achieving seizure-free outcomes. A systematic review of epilepsy surgery studies showed a seizure-free rate of 27% with frontal lobe surgery in all age groups.24 In clinical series that looked at adult and pediatric patients together, the range of seizure-free outcomes for frontal lobe resections with an average of 1.8 to 16 years’ follow-up was 23% to 64%.36,40,42,43,74–78 For adults only, at an average follow-up of 1 to 5 years the seizure-free rate after frontal lobe epilepsy surgery was 25% to 50%.36,40,79 In the pediatric frontal lobe epilepsy surgery population, after an average of 2 to 3.6 years the seizure-free rate varied widely from 9% to 75%.36,40,49,51,52 There is some variability in postoperative seizure control in this population, and it is not as good as with temporal lobe surgery, but with seizure-free rates possibly being 50% or better, these surgeries should still be considered a possible option. Lower seizure-free rates in series with longer average follow-up periods may indicate long-term relapse of seizures after frontal lobe epilepsy surgery (Fig. 61-1) (Case Study 61-1).
Case Study 61-1
A 29-year-old woman underwent surgical evaluation for complex partial seizures dating back to 8 years of age, when Sturge-Weber syndrome was diagnosed. Her seizures were characterized by unresponsiveness, a blank stare, eyelid flutter, and rubbing her fingers together or rubbing her arms. These seizures would typically last 10 to 40 seconds; she had 15 to 25 seizures per month with occasional generalization. Her seizures had proved resistant to multiple pharmacologic agents. Her interictal EEG showed generalized frontotemporal slowing greater on the right than on the left, frontally predominant polyspike and wave discharges on the right, and isolated right temporal spike discharges. During EEG video telemetry, the patient’s seizures were characterized by wide-eyed staring, lifting of both arms above her head, rubbing her face, and moving the pillow and covers. EEG recorded during these episodes suggested frontal lobe seizures with the possibility of right frontal onset, but because of rapid propagation to the left frontal lobe, the possibility of bilateral or left frontal onset could not be ruled out. MRI and an angiogram during intracarotid sodium amobarbital testing showed a right frontal venous angioma (Fig. 61-E1A). The intracarotid sodium amobarbital test indicated that the left hemisphere was dominant for language. MEG results were consistent with widespread right anterior frontal and orbitofrontal activity, but with some activity in the left frontal region (Fig. 61-E1B).
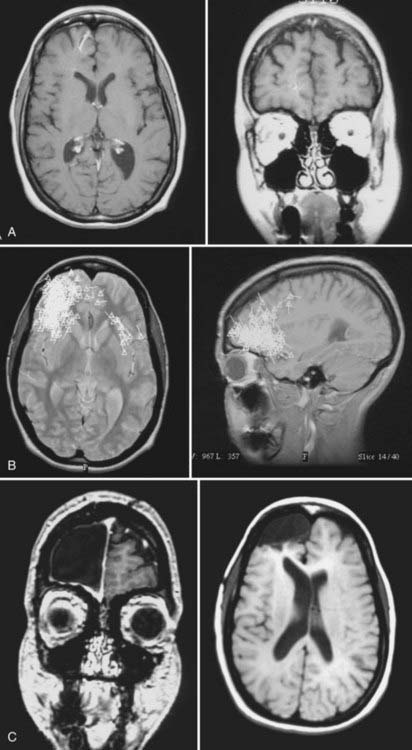
FIGURE 61-E1 Case Study 61-1. A, Preoperative magnetic resonance imaging (MRI) shows a right frontal venous angioma in the region consistent with electroencephalographic localization of the seizure focus. B, A magnetoencephalogram superimposed on MRI shows epileptiform spikes localized to the frontopolar region, consistent with the area of the venous angioma seen on MRI in A. C, Postoperative MRI shows resection of the frontopolar region, including the venous angioma seen in A.
Based on the MEG findings, subdural grid and strip electrodes were surgically placed over the right frontal region and extended over the mesial and lateral frontal and the orbitofrontal regions. Ictal recordings from these electrodes showed onset in the anterior right frontal lobe. Electrocorticography at the time of resective surgery showed spiking activity at the anterior, mesial, and lateral extents of the craniotomy in the right frontal lobe. The tissue surrounding the venous angioma was resected en bloc and consisted mostly of the middle frontal gyrus with some additional cortical tissue at the frontal pole (Fig. 61-E1C). The resection included the anterior mesial frontal region. Somatosensory evoked potential mapping indicated a margin of safety between the posterior border of the resection and the rolandic cortex. Postresection electrocorticography showed no residual spiking activity, so the resection was carried no further. The pathology reading for the resected tissue was cortical dysplasia. Postoperatively, the patient was seizure free and remained so at 5-year follow-up.
Parietal Lobe Epilepsy
Parietal lobe epilepsy is relatively rare in series of epilepsy surgeries, perhaps because of an innate resistance to seizures in the region; the difficulty of localizing these seizures, which may be accompanied by symptoms referable to other lobes; and the reluctance of surgeons to resect tissue in this area. Seizures originating in the parietal area have not received as much investigation as frontal lobe or temporal lobe seizure syndromes. Parietal lobe seizures are typically characterized by somatosensory auras,80,81 pain, paresthesias, vertigo, head and eye deviation, complex visual hallucinations, sensations of body movements, and actual complex movements of the extremities.82–84 Except for the primary sensory cortex, there is a paucity of reported clinical and electrophysiologic correlates of seizure activity.82 Combined with a tendency for seizures to spread quickly to other regions of the brain,81 this paucity makes characterization and localization of the seizure focus based on semiology and scalp EEG difficult. Similar to frontal lobe epilepsy, demonstration of focal lesions on MRI or other imaging modalities is paramount in determining the appropriate surgical resection site.
Tailored resections of the parietal lobe do not usually include the primary somatosensory cortex because of the importance of this cortex in the production of skilled movement, as described by Penfield and Erickson.85 Similar to the primary motor cortex, it has been suggested that the primary sensory cortex can be resected nearly 3 cm above the sylvian fissure in the nondominant and dominant hemispheres without significant deficit as long as the tongue, thumb, and lip areas are identified and preserved.70 Any resection of the parietal lobe in the dominant hemisphere should remain above the intraparietal sulcus to prevent damage to the receptive language center.70 In general, resections in the parietal dominant hemisphere should take into consideration the high probability of language deficits in the vicinity of the sylvian fissure and more superiorly in regions such as the angular gyrus. As a general rule, resections in the dominant parietal lobe should be carried out only after electrical stimulation mapping. Preoperative fMRI can be useful in providing a general idea of language representation in the region but cannot be relied on with respect to final surgical decision making. With significant resection of the parietal lobe, a contralateral inferior quadrantanopia should be expected.70 The nondominant parietal lobe mediates important visuospatial functions. Large resections in this lobe cannot be undertaken without severe impairment in spatial cognition. We have performed focal resections in the nondominant parietal lobe when invasive monitoring showed a fairly circumscribed epileptogenic region (Case Study 61-2) but have resorted to multiple subpial transections when a large nondominant parietal territory was involved (see Case Study 61-4).
Case Study 61-2
An 18-year-old, right-handed man with seizures since the age of 6 was admitted for presurgical evaluation. His seizures were characterized by an aura of impending doom. Initially, his seizures consisted of 20 seconds of staring, but later the semiology changed to staring and dystonic posturing in a sitting position with outstretched arms that lasted 20 seconds, occasionally followed by left face and arm twitching with drooling. Postictally after the longer seizures, he exhibited left-hand weakness and dysarthric speech. On average, he had more than one seizure per day. The patient was prescribed several different antiepileptic medications and had a vagal nerve stimulator placed without alleviating the seizures. Video EEG monitoring showed rare right temporal spike and wave complexes with seizure activity originating in the right temporal region. MRI was unremarkable, but PET showed right inferior parietal hypometabolism (Fig. 61-E2A). MEG showed dipoles in the right posterior temporal and inferior parietal regions (Fig. 61-E2B). These findings suggested possible temporal and parietal lobe involvement; accordingly, subdural electrodes were surgically implanted to cover the frontotemporoparietal region, with strips covering the basal temporal lobe, anterior frontal lobe, posterior parietal region, and superior frontoparietal junction. These subdural electrodes localized the region of seizure onset to the right temporoparietal cortex, including the supramarginal gyrus and posterior superior temporal gyrus. The region of ictal onset corresponded well to the region of interictal abnormalities, but was smaller. For resective surgery, intraoperative evoked potentials and electrical stimulation mapping were used to define the rolandic cortex. Intraoperative electrocorticography confirmed localization of the epileptogenic region to the temporoparietal junction and was similar to the interictal localization obtained by long-term recordings with the subdural grid. Resection included the area surrounding the posterior edge of the sylvian fissure on the parietal and temporal sides. Subpial transections were performed at the anterior border of the resection cavity, where interictal abnormalities were observed close to the somatosensory cortex. Postresection electrocorticography showed slowing but no residual spikes. Pathologic evaluation of the resected tissue revealed no histologic abnormalities. The patient has remained seizure free for more than 1 year, although longer follow-up is required to assess the success of surgery.
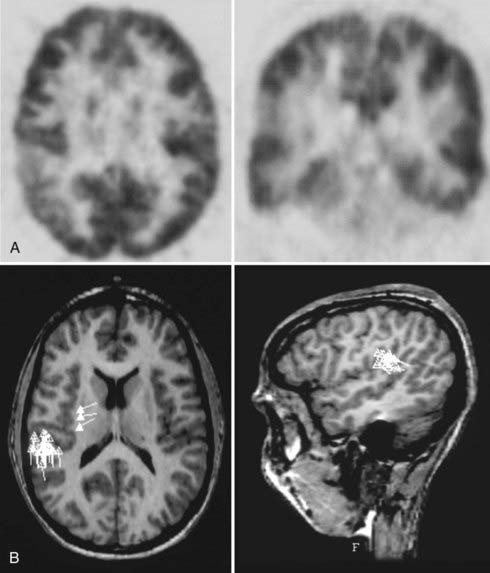
FIGURE 61-E2 Case Study 61-2. A, Positron emission tomography (PET) shows a region of hypometabolism in the right lateral parietal lobe. B, A magnetoencephalogram superimposed on magnetic resonance imaging shows spiking activity focused in the right parietal lobe, consistent with the findings of PET in A.
Seizure control outcomes after parietal resection are reportedly slightly better than outcomes after frontal lobe resection. A systematic review of epilepsy surgery studies showed a seizure-free rate of 46% with parietal lobe surgery in all age groups.24 Combined adult and pediatric series with an average follow-up of 2 to 20 years show seizure-free rates of 46% to 100% after parietal lobe resection.36,42,43,53,81,86,87 Adult series of parietal lobe epilepsy surgery with an average follow-up of 2 to 2.5 years report 90% to 100% seizure-free rates.36,53 Pediatric series of parietal lobe epilepsy surgery with an average 2 to 3.6 years’ follow-up have reported 33% to 100% seizure-free rates.36,49 Lower seizure-free rates in series with longer average follow-up periods after parietal lobe epilepsy surgery, as with frontal lobe surgery, may indicate long-term relapse over time (Fig. 61-2).
Occipital Lobe Epilepsy
Occipital lobe epilepsy is also rare. Clinically, occipital lobe epilepsy is characterized most often by visual auras.54,88,89 These visual auras are elementary in nature and are described as lights, spots, or simple shapes that can be flashing or moving. Formed visual hallucinations are more suggestive of temporal lobe epilepsy, either primary or from spread of an occipital lobe seizure. Another clinical feature of occipital lobe epilepsy is episodic blindness,88–91 which can involve half the visual field or the entire visual field. Other signs observed with occipital seizures include blinking and tonic or clonic eye deviation.88,89 Occipital lobe seizures can spread rapidly to the temporal lobe, thus making localization of their onset difficult and occasionally leading to relatively ineffective temporal lobectomy.89
Resective surgery for occipital lobe epilepsy is almost certainly going to lead to some degree of visual deficit,70 and this procedure must be considered carefully by the patient and the surgical team. Impairment of sight could be more disabling than the seizures. It would be unfortunate for a patient to undergo surgical intervention for seizure control in anticipation of regaining a driver’s license simply to have the license denied because of a dense hemianopia. Many of these patients have preoperative visual field deficits, especially if there is a mass underlying the seizure disorder, and there is a risk in not controlling the seizures because permanent blindness has been described after recurrent, uncontrolled occipital seizures.92 Given the frequent spread of occipital lobe seizures to the temporal lobe, resections are sometimes designed to include some portion of the temporal lobe.44,88,89
Postoperative seizure control outcomes for occipital lobe epilepsy patients have varied significantly among series. A systematic review of epilepsy surgery studies showed a seizure-free rate of 46% with occipital lobe surgery in patients of all ages.24 In several series of adult and pediatric patients combined at an average follow-up of 2 to 17 years, seizure-free rates were 20% to 88%.36,42,43,81,87,88,93 Adult series alone showed a seizure-free rate of 46% to 100% after an average 2- to 6-year follow-up.36,54 Pediatric occipital lobe epilepsy patients had a seizure-free rate of 0% with an average follow-up of 3.6 years.49 Similar to frontal lobe resective surgery for epilepsy, seizure control outcomes for occipital lobe epilepsy surgery may suffer because of a more conservative approach to the extent of cortical resection. Interestingly, occipital lobe epilepsy surgery series do not show lower seizure-free rates in series with longer average follow-up periods (Fig. 61-3) (Case Study 61-3).
Case Study 61-3
A 38-year-old man who has had seizures since the age of 13 initially had generalized tonic-clonic seizures that would occur at night, but 5 years before surgery, he began having seizures during the day in which he would become disoriented, experience vertigo and partial loss of awareness, and turn his head and eyes to the right. The generalized tonic-clonic seizures began with lifting of his right arm and lasted for 0.5 to 2 minutes. He typically had 5 to 10 focal seizures per day and 1 to 2 generalized seizures per month. On video EEG monitoring, the seizures appeared to begin in the left occipital region but almost instantly spread to the left parasagittal area. On MEG, he had dipoles not only in the mesial part of the left occipital lobe but also in bilateral central parietal areas (Fig. 61-E3A). MRI and SPECT were unremarkable, and PET showed no regions of focal hypometabolism. Neuropsychological testing indicated involvement of the nondominant hemisphere. Intracranial depth electrodes were stereotactically placed in the right occipital, right parietal, right parahippocampal gyrus, right supplementary motor, left occipital, left posterior parietal, left parietal, left parahippocampal gyrus, and left supplementary motor areas (Fig 61-E3B). Evaluation of seizures with these electrodes indicated a left frontal onset of the seizures. The depth electrodes were removed, and at a later time, frontal subdural grid and strip electrodes were placed through bilateral frontal craniotomies with extensive sampling of the left mesial frontal region. A left mesial frontal focus was delineated, and this region was resected with electrocorticographic guidance and somatosensory evoked potential and stimulation mapping of the motor cortex. The pathology was consistent with a cavernous hemangioma, a lesion that was not seen on any of the preoperative imaging studies. The patient is currently nearly seizure free 3 years after surgery.
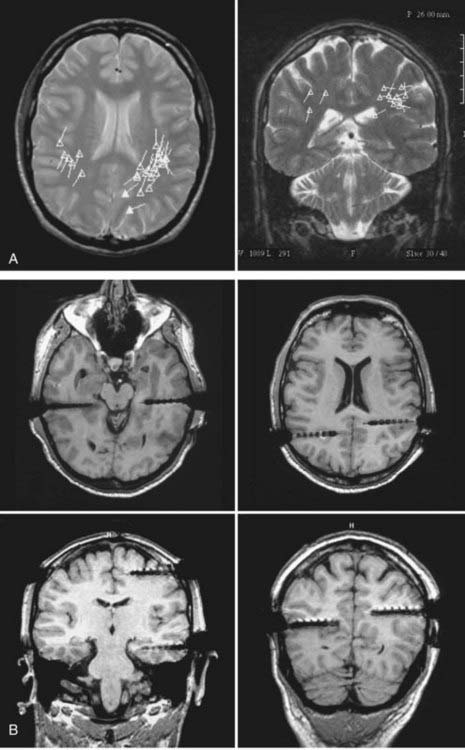
FIGURE 61-E3 Case Study 61-3. A, A magnetoencephalogram superimposed on magnetic resonance imaging (MRI) shows bilateral parietal spikes, more on the left than the right. B, MRI shows placement of depth electrodes (MRI artifact exaggerates the size of the electrodes, which have a diameter of 1.3 mm).
Multilobar Resection
Extratemporal lobe epilepsy may involve more than one lobe, particularly in pediatric patients,50,94 and in such cases multilobar resection involving two or three lobes may be necessary. The more common multilobar resection patterns are frontal-temporal,44 frontal-parietal, and temporal-occipital.50 These resections are designed around preserving regions of important function, such as motor-sensory cortex and language areas. The range of seizure-free rates in combined adult and pediatric patients undergoing multilobar resection was 15% to 60% with an average of 2 to 11 years’ follow-up.36,38,69,94 Adult series of multilobar resection showed a 25% to 50% seizure-free rate after 1 to 5 years.38,79 Pediatric multilobar resection patients with an average of 2 to 5 years’ follow-up had a seizure-free rate of 22% to 100%.36,38,46,51 The higher rates of success in children may be due to more aggressive removal of tissue than in adults because the greater plasticity in an undeveloped brain allows better functional recovery (Fig. 61-4).
Hemispherectomy/Hemispherotomy
When the epileptogenic region is extensive enough to involve the cortex of one cerebral hemisphere entirely or almost entirely, removal or disconnection of the whole hemisphere may be in order, especially for pediatric patients.95–97 The original procedure to accomplish this was anatomic hemispherectomy, in which the entire hemisphere neocortex was resected.98 Functional hemispherectomy and hemispherotomy procedures were developed in an attempt to leave some of the brain tissue intact for structural purposes to alleviate the issues of cerebral shift, hemosiderosis, and large subdural hygromas. These procedures were designed to remove only the amount of tissue necessary to completely disconnect the hemisphere’s neocortex from other parts of the brain. Several variants of the procedure have been described.99–103 Hemispherectomy and hemispherotomy are more commonly reserved for pediatric patients, who have the best opportunity for regaining neurological function over time. In the series by Eriksson and coworkers,36 in which pediatric and adult epilepsy surgeries were compared, eight hemispherectomies were performed in the pediatric group and none in the adult group. The procedure has been used in adult patients with hemispheric atrophy and fixed unilateral motor deficit. Given the inevitable minimum neurological deficit of decreased fine motor control and the higher risk for surgical complications than with other epilepsy surgeries (16% in one series29), these procedures should be considered only for severe epilepsy syndromes with full comprehension of the risks by the family and be performed only by a surgeon who is experienced in this specific type of surgery. Outcomes after hemispherectomy have been reported only in pediatric epilepsy series and range from 40% to 83% seizure-free rates after an average 1- to 5-year follow-up.36,48,51,104–107 Outcomes are somewhat dependent on the underlying pathology105 and the method used for disconnection of the hemisphere.108 Patients with hemispheric cortical dysplasia and Rasmussen’s encephalitis had the best postoperative seizure-free rates, 81% at 1 year and 60% to 62% at 5 years, followed by patients with infarct or ischemia, who had seizure-free rates of 75% at 1 year and 71% at 5 years, and hemimegalencephaly patients, who had the worst seizure-free rates, 57% at 1 year and 33% at 5 years.105 Based on the technique used for hemispherectomy, the highest reoperation rate for recurrent seizures occurred after functional hemispherectomy, the longest hospital stay and highest rate of shunt requirement were associated with anatomic hemispherectomy, and the least blood loss and lowest complication rate were associated with the lateral hemispherotomy technique.108
Other Surgical Techniques
Disconnection Procedures
Multiple Subpial Transection
Multiple subpial transection (MST) involves inserting a specially designed instrument through the pia at one side of a gyrus and transecting the cortical ribbon of that gyrus subpially to the other side of the gyrus.109,110 Subpial transections are traditionally performed approximately every 5 to 10 mm along the length of each gyrus within a region of epileptogenicity. These transections are thought to divide the fibers connecting adjacent regions of the cortex while leaving the projection fibers in and out of the region intact. MST has shown some efficacy in eliminating or reducing seizures without compromising function of the cortical region. Although one series of pediatric epilepsy patients reported that all 20 had transient hemiparesis after MST of the primary motor cortex,111 permanent motor deficit after MST of the primary sensory-motor cortex is not typically reported.111,112 A very small series of MST in the language cortex suggests that MST in the posterior language cortex leads to language dysfunction that will improve over time but that MST in the frontoparietal language areas does not lead to language deficits.113 In contrast, a meta-analysis of MST showed a 22% incidence of new neurological deficits after MST,114 so the possibility of neurological deficits should be considered carefully and discussed with the patient and family. Subpial transections are typically used in regions of cortex with critical functions (Case Study 61-4) and can be performed in combination with tissue resection techniques.109,110
Case Study 61-4
A 36-year-old man had his first seizure at the age of 32. The seizures were typically characterized by visual hallucinations of the number 103 followed by loss of awareness and cheering and clapping behavior and then postictal confusion and sleepiness. He was having approximately two to three seizures per week, only a third of which began with a visual aura. The seizures had proved resistant to pharmacotherapy with four different medications in various combinations. Interictal scalp EEG showed right parietal spike and slow wave activity, and video EEG monitoring captured three complex partial seizures that localized to the right central parietal region and one rapidly secondarily generalized seizure in which his head turned to the left before generalization. MRI findings were normal, but PET showed mild right temporal hypometabolism. MEG showed clusters of spike activity over the posterior temporal and parietal regions of the right hemisphere (Fig. 61-E4). The patient had subdural grid electrodes surgically implanted through a right temporal/parietal/occipital craniotomy. Activity recorded from these subdural electrodes showed diffuse onset of the seizures from the right parietal and temporal regions. After extraoperative monitoring, the patient returned to the operating room for surgical treatment of the epilepsy. Stimulation motor mapping and electrocorticography were performed. A biopsy specimen taken from a region well behind the rolandic cortex showed no abnormality on pathologic evaluation. Multiple subpial transection (MST) guided by electrocorticography and the information obtained from the subdural grids before the operation was carried out during surgery. MST was used because the seizure focus overlapped regions of rolandic cortex and extensive regions of the nondominant parietal lobe.
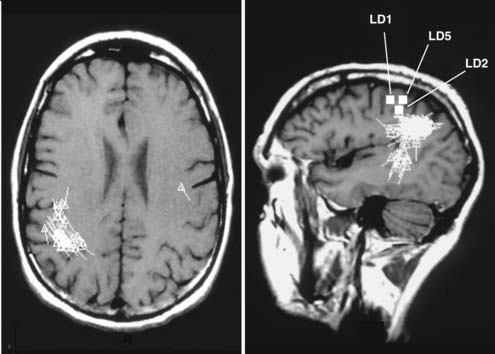
FIGURE 61-E4 Case Study 61-4. A magnetoencephalogram superimposed on magnetic resonance imaging shows spikes primarily localized to the right lateral parietal and posterior temporal lobes but with some spikes in the left parietal lobe. LD1, LD2, and LD5 denote dipoles related to the somatosensory representation of the left-hand digits.
The most comprehensive analysis of outcomes after MST is a meta-analysis of six epilepsy surgery programs with a total of 211 patients.114 This study classified patients with excellent outcome as those with a greater than 95% reduction in seizures. In patients who underwent MST in addition to resection, 87% with generalized seizures, 68% with complex partial seizures, and 68% with simple partial seizures had excellent outcomes. In patients undergoing MST alone, 71% of generalized, 62% of complex partial, and 63% of simple partial epilepsy patients had excellent outcomes. However, only 16% of patients became seizure free after MST in another systematic review of adult and pediatric epilepsy surgery.24 Case series of outcomes after MST, with or without cortical resection, vary in their reported success rates, with 0% to 50% being seizure free, 30% to 62% having a significant reduction in seizures,107,110,111,115–120 and one report of relapse of seizures between 2 and 5 years after surgery in 18.5% of patients.120
Corpus Callosum Section (Corpus Callosotomy)
In corpus callosotomy, a portion of the corpus callosum, usually the anterior two thirds, is divided in an attempt to eliminate most connections from one cerebral hemisphere to the other, in this manner curtailing sudden generalization by preventing spread of seizures from one side of the brain to the other.121 Corpus callosotomy is indicated in patients with drop attacks and generalized epilepsy in which the seizures spread throughout the brain so quickly that they cause complete flaccid paralysis and falling, which poses considerable risk for serious injury. These patients are severely affected by the resulting repeated head trauma. Corpus callosotomy rarely eliminates seizures (6% to 19% of patients)122–125 but is primarily designed to change the character of the seizures to eliminate drop attacks. A systematic review of the epilepsy surgery literature showed that only 35% of corpus callosotomy patients became free of their most disabling seizures.24 However, 33% to 92% of patients had significant improvement in their seizures after corpus callosotomy,107,121–139 depending on the extent of the callosotomy (partial versus total section)138–140 and type of seizures.122
Corpus callosotomy can lead to disconnection syndromes that may be disabling.121,141 The reported incidence of disabling disconnection syndromes varies from series to series, with one reporting 4 of 20 having a disconnection syndrome,134 one reporting only transient disconnection syndrome in 57% of patients,135 one reporting no severe neuropsychological deficits in 15 patients,133 and one reporting neuropsychological benefits in all 25 patients in the series after corpus callosotomy.142 Because of concern about surgical morbidity and disconnection syndromes, many epilepsy surgery programs consider vagal nerve stimulator implantation the first-line surgical therapy for severe generalized seizures before considering corpus callosum section.126
Stimulation Procedures
Implantable stimulation devices have added to the surgical options for the treatment of medically intractable epilepsy. Stimulation devices are especially important in the treatment of extratemporal lobe epilepsy because the foci are more likely to be located in areas not amenable to surgical resection. The vagal nerve stimulator has been approved by the Food and Drug Administration (FDA) for the treatment of medically intractable epilepsy and has been commercially available for years. This device is 30% to 50% effective in providing at least a 50% reduction in seizures in patients with medically intractable seizures who are not surgical candidates.8,143–145
The only permanently implanted brain stimulation device approved by the FDA for human use is the Medtronic, Inc., DBS device. This device is approved for the treatment of movement disorder, including Parkinson’s disease, and is widely used in that capacity. It is thought to exert its effects by disrupting or inhibiting local neuronal activity in the target nucleus, the STN in patients with Parkinson’s disease. Various regions of the brain have been investigated as potential targets for the treatment of medically intractable epilepsy with DBS. Because of the extensive experience with DBS electrodes implanted in the STN for Parkinson’s disease, this seemed an obvious potential target for evaluation by two groups of investigators. Each of these two studies had two medically intractable epilepsy patients implanted with bilateral STN-DBS electrodes.146,147 In one study with an 18-month follow-up for one patient and 6-month follow-up for the other, they found an 86.7% and 88.6% reduction in seizure frequency, respectively.146 In the other study with 26- and 32-month follow-up, the two patients had reductions in seizure frequency of 44% and 29%, respectively.147 This gives an average seizure reduction rate for bilateral STN-DBS of 62%, with no patients being seizure free, two patients having greater than a 50% reduction in their seizures, and two patients having less than a 50% reduction in their seizures.
Another popular and fairly obvious target for DBS to control epilepsy is the medial temporal lobe for medial temporal lobe epilepsy. One group first tested short-term stimulation in 10 patients with bilateral hippocampal depth or unilateral basotemporal strip electrodes as part of their evaluation before temporal lobectomy surgery. Over a period of 16 days of stimulation, 7 patients had no clinical seizures and decreased interictal EEG spikes, whereas 3 had no antiepileptic effects.148 Based on these results, they implanted unilateral hippocampal DBS stimulators in 9 patients with complex partial intractable epilepsy. With at least 18 months of follow-up, 5 patients were reportedly seizure free and 4 had continued seizures.149 In another study of 10 medial temporal lobe epilepsy patients who underwent bilateral hippocampal DBS with an average follow-up of 31 months, 1 patient was seizure free, 6 patients had greater than a 50% reduction in their seizures, and 3 had less than a 50% reduction in seizures.150
Thalamic targets have also shown promise for DBS treatment of epilepsy. In one study of bilateral anterior thalamic nuclei DBS with a 36-month follow-up, there was a mean reduction in seizure frequency of 75.6%, with all four patients having a greater than 50% reduction in seizures.151 In another study with six bilateral anterior thalamic DBS patients, five had greater than a 50% reduction in seizures and one had less than a 50% reduction.152 Thus, for DBS stimulation of the anterior thalamus, no patients were seizure free, nine patients had greater than a 50% reduction in seizures, and one patient had less than a 50% reduction. A new study of DBS of the anterior thalamus has recently been completed, but detailed results have not yet been published.11
In a study that included 2 patients with bilateral centromedian thalamic nuclei DBS, neither had any reduction in seizure frequency.152 Another study of 13 patients with Lennox-Gastaut syndrome involving medically intractable generalized tonic-clonic and atypical absence seizures treated by bilateral centromedian thalamic nuclei DBS showed an average reduction in seizures of 80% at 18 months of follow-up.153 Taken together, these studies show that for centromedian thalamus DBS there were 2 seizure-free patients, 10 patients with greater than a 50% reduction in seizures, and 3 patients with less than a 50% reduction.
Other potential DBS targets attempted for the treatment of medically intractable epilepsy include the cerebellum and caudate nucleus. Cerebellar stimulation has received mixed reviews; one study showed no reduction in seizure frequency in 12 epilepsy patients with cerebellar stimulation,154 whereas in another study of 27 patients with cerebellar stimulators, 12 were seizure free, 11 had a significant reduction in seizures, and 4 had no change or their condition worsened.155 In a study that assessed patients with cerebellar dentate nucleus stimulation, thalamic centromedian stimulation, and cortical epileptic focus stimulation together, 26 of 54 were seizure free, 23 of 54 had a significant reduction in their seizure frequency, and 5 had no reduction in seizures.156 Stimulation of the caudate nuclei in 57 intractable epilepsy patients was effective in decreasing epileptiform electrical activity.157
Techniques are being developed to use seizure-detecting algorithms for predicting seizures and preventing their propagation with triggered brain stimulation.158,159 A new brain stimulation device undergoing human trials for FDA approval is the Responsive Neurostimulator (RNS) from Neuropace, Inc.160 Much like the DBS device on the market, the RNS has electrodes that can be implanted either as depth electrodes in deep regions of the brain or as subdural electrodes against the cortical surface. The RNS is unique in that it records electrical activity from the brain and can be programmed with seizure detection algorithms that trigger brain stimulation at electrical signs of seizure onset. This responsive stimulation is intended to block progression of the seizure. Although many patients are involved in the FDA trial of the device, the results from only a few patients have been published at this point.161 In this paper, preliminary results from 8 patients with the RNS system after an average follow-up of 9.2 months were presented. The electrode sites varied, with 6 patients receiving hippocampus electrodes and 2 receiving neocortical temporal subdural electrodes. Although none of the patients were completely seizure free, 7 of the 8 patients had greater than a 45% reduction in seizure frequency. A more recent presentation of results from 50 patients with implanted RNS systems reported a 50% or greater reduction in total disabling seizures in 25% of patients at 3 months, in 48% at 8 months, and in 54% at 36 months.162
The new technology of transcranial magnetic stimulation obviates the need for surgical implantation of a stimulation device. By placing a specially designed magnetic coil near the head and generating a magnetic field, a corresponding current can be generated in the underlying neocortex. Attempts at seizure control with transcranial magnetic stimulation have used repetitive stimuli. Repetitive-stimulus transcranial magnetic stimulation (rTMS) is thought to be inhibitory at low frequencies (≤1 Hz or less) and stimulatory at high frequencies (>1 Hz). The low-frequency stimulation rate most commonly used in attempts to control seizures is 1 Hz. Two randomized, controlled studies of 1-Hz rTMS for control of medically refractory epilepsy came to different conclusions. In one study of 21 patients, rTMS significantly decreased the number of seizures for at least 2 years when compared with placebo,163 but another study of 24 patients found a minimal effect in reducing seizures, and the effect quickly disappeared.164 Another group found that low-frequency rTMS of the seizure focus at 0.9 Hz reduced the frequency of seizures an average of 19%.165 Low-frequency rTMS at 0.5 Hz has reportedly reduced seizure frequency by 14% to 51%,166–168 with one case report showing nearly complete elimination of epilepsia partialis continua.169 At 0.3-Hz rTMS, seizures were reduced by 22.8%.170 Although one group was able to inhibit epilepsia partialis continua with a combination of low-frequency (1 Hz) and high-frequency (20 to 100 Hz) rTMS,171,172 high-frequency rTMS does not seem to hold promise for the treatment of epilepsy, with one case report showing no effect on poststimulus epileptic activity173 and another case report of high-frequency rTMS triggering a seizure.174 There is also a case report on the use of another magnetic technology, the ion magnetic inductor, to successfully reduce seizure frequency.175 The seizure control results from rTMS show that it is not as effective as surgically implanted electrical stimulation devices; however, rTMS is an extracranial technology and thereby reduces the risks associated with brain surgery. Although seizures triggered by rTMS have been reported,176,177 the risks to epilepsy patients during treatment with rTMS are minimal, with seizures occurring in 0% to 3.6% and the most common side effect being headaches, which occurred in 9.6%.178,179 These various stimulation techniques under development would provide more options for patients with extratemporal lobe epilepsy who are not candidates for surgical resection.
Pathologic Substrates
The pathologic substrates underlying extratemporal lobe epilepsy syndromes are important in planning surgery and also influence the seizure control outcomes of patients. It is clear that the presence of a defined lesion with a pathologic substrate signifies a better prognosis and that the so-called nonlesional extratemporal epilepsy presents a more difficult challenge in epilepsy surgery. Focal abnormalities, such as tumors, vascular lesions, and some cortical abnormalities, may have relatively distinct borders that can be differentiated more easily from normal brain tissue intraoperatively. Such differentiation facilitates identification of the epileptogenic substrate and complete removal of the entire abnormality. Analysis of several extratemporal lobe epilepsy surgery series showed various categories of epileptogenic pathology.*
Neoplastic Lesions
Neoplasms frequently underlie epileptic foci. Based on frequency of occurrence in extratemporal epilepsy surgery series, these tumors, in order of their frequency, were dysembryoplastic neuroepithelial tumors, 2% to 36%; gangliogliomas, 2% to 35%; astrocytomas, 1% to 31%; oligodendrogliomas, 2% to 20%; glioneural hamartia, 14%; hamartomas, 1% to 13%; oligoastrocytomas, 2% to 12%; meningiomas, 6%; and ependymomas, 2%.† Some of the extratemporal lobe epilepsy series simply listed neoplastic causes in 2% to 70%.41,45,48,75,81,87,89 In series that combined temporal lobe epilepsy and extratemporal epilepsy surgery, with the majority being temporal lobe surgery, the frequencies were slightly different, with gangliogliomas in 1% to 38%, astrocytomas in 2% to 31%, oligodendrogliomas in 1% to 15%, dysembryoplastic neuroepithelial tumors in 1% to 11%, hamartomas in 6%, xanthoastrocytomas in 3%, ependymomas in 3%, gangliocytomas in 2%, oligoastrocytomas in 1%, epidermoids in less than 1%, generically listed tumors in 2% to 33%, and gliomas in 5% to 7%.36,39,46,69,182,183 Extratemporal series with adult patients only53,77 differ in the underlying pathologies from extratemporal series with pediatric patients only38,45,48,49,51,52 in that certain pathologies were found only in pediatric patients, such as gangliocytoma and hamartoma; some were more frequent in pediatric patients, such as dysembryoplastic neuroepithelial tumors; several were about equal in both groups, including astrocytoma, ganglioglioma, and oligodendroglioma; and only oligoastrocytoma was seen solely in adults.
Epileptogenic tumors may involve the limbic system184 but are commonly seen in the temporal lobe and extratemporal locations. In general, epileptogenic tumors occur in young people, are slow growing, and involve the cortical gray matter. These tumors can be addressed best with an extended lesionectomy in an effort to remove the tumor and its margins in the surrounding brain. As long as the entire lesion with margins is removed, the seizure-free outcome after resection of neoplasms is likely to be good. Surgical outcome depends on the extent of resection. Partial resections of neoplasms usually yield a poor seizure outcome, and the best results are achieved with initial complete resection of the lesion and surrounding margins.183 There are differing opinions in the literature regarding the benefit of resection of additional contiguous tissue based on electrocorticography.185,186 Reported seizure-free outcomes for extratemporal lobe epilepsy surgery vary by pathology, with gangliocytomas associated with a 100% seizure-free rate, oligoastrocytomas, 100%; dysembryoplastic neuroepithelial tumors, 75% to 100%; gangliogliomas, 67% to 100%; oligodendrogliomas, 50% to 100%; hamartomas, 0% to 100%; and astrocytomas, 0% to 100%.49,51–53,77,81,86,88
Vascular Lesions
The two vascular lesions classically associated with seizures are arteriovenous malformations (AVMs) and cavernous hemangiomas. Detection of cavernous hemangiomas as seizure foci has been improving since the development and refinement of MRI in the preoperative evaluation for epilepsy surgery. Venous angiomas have also been found in patients with epilepsy, but they are not always the cause of the seizures. Some associated underlying developmental abnormality may be the culprit, as illustrated in Case Study 61-1. The other vascular abnormality listed in some surgical epilepsy series is seen in connection with Sturge-Weber syndrome, a pathology more commonly found in pediatric patients. The vascular lesions reported in extratemporal lobe epilepsy series are Sturge-Weber syndrome in 1% to 30% of patients, AVMs in 2% to 10%, cavernous hemangiomas in 2% to 10%, and venous angiomas in 1% to 10%, with nonspecific vascular lesions being reported in 2% to 9%.* The combined temporal and extratemporal series report no venous angiomas and have lower frequencies of vascular lesions, with Sturge-Weber syndrome in 1% to 6%, cavernous hemangiomas in 4% to 5%, AVMs in 1% to 2%, and generically reported vascular lesions in 8%.36,39,69,182 In comparing adult-only extratemporal series53,77 with pediatric-only extratemporal series,38,45,48,49,51,52 AVMs, cavernous hemangiomas, and venous angiomas were found only in the adult series, whereas Sturge-Weber syndrome was found predominantly in the pediatric series.
Seizure-free outcomes of extratemporal epilepsy surgery vary with the type of vascular lesion resected. Patients with cavernous hemangiomas and venous angiomas have a reported seizure-free rate of 100%, those with AVMs have a 67% to 100% seizure-free rate, and the rate in patients with Sturge-Weber syndrome is 0% to 100%.45,51,53,77,81,86,88 Seizure outcomes after removal of cavernous hemangiomas appear to be comparable to those after resection of neoplasms. A paper looking only at cavernous hemangiomas found seizure-free outcome rates of 88% for frontal lobe, 78% for parietal lobe, 100% for occipital lobe, and 75% for multilobar resections.187 We believe that removal of the margins of this lesion is important in achieving good seizure outcomes. This contrasts with a similar paper looking only at AVMs, which reported a lower seizure-free rate of 66%.188
Gliotic Lesions
Extratemporal lobe series report many types of epileptogenic lesions that would be gliotic in nature, including gliosis in 6% to 65%, scar or posttraumatic lesions in 2% to 40%, encephalomalacia in 2% to 25%, perinatal infarct or trauma in 15% to 19%, infarct in 1% to 18%, atrophy in 14%, necrosis in 10%, abnormal cysts including porencephalic cysts in 2% to 10%, gliomesodermal scar in 8%, postinfectious lesions in 2% to 7%, meningocerebral cicatrix in 5%, foreign body in 2%, and old hematoma in 2%.* Combined temporal and extratemporal series report only gliosis in 6% to 45%, infarct in 21%, perinatal infarct or trauma in 8%, and scar or posttraumatic lesions in 4% to 5%, but they do include hippocampal sclerosis in 5% to 23%, which is exclusively a temporal lobe pathology.36,39,46,69,182 The pediatric-only series reported postinfarct cases, whereas the adult-only series did not; the adult-only series reported scar or posttraumatic cases only, whereas the pediatric series did not; but both groups reported many gliosis cases that may be from these causes but were not distinguished in the papers.38,45,48,49,51–53,77 Surgery on these pathologies has mixed results, with seizure-free rates of 57% to 100% for infarct, 38% to 100% for scar or posttraumatic causes, 0% to 100% for gliosis, 0% to 71% for postinfectious causes, 58% to 67% for perinatal infarct or trauma, 0% for cysts including porencephalic cysts, and 0% for meningocerebral cicatrix.45,49,51–53,77,86,88
Developmental Lesions
Extratemporal lobe epilepsy surgery series report the following rates of developmental lesions: cortical dysplasia in 2% to 83%, neuronal migration disorders in 1% to 56%; cytoarchitectural abnormalities in 28%; macrogyria in 20%; cortical malformations in 3% to 17%,; tuberous sclerosis in 2% to 17%; hemimegencephaly in 7% to 10%; microgyria in 5% to 10%; heterotopia in 2% to 10%; ulegyric change in 6%; and ossification in 2% to 3%.† Many of these pathologies may overlap, given that they are dependent on the pathologist’s interpretation and nomenclature for the abnormal developmental process.7 The combined temporal and extratemporal series mention only cortical dysplasia (5% to 56%), neuronal migration disorder (15%), microgyria (5% to 14%), cortical malformation (6%), and tuberous sclerosis (1% to 6%) as developmental pathologies.36,39,46,69,94,182 Cytoarchitectural abnormalities and microgyria were part of the adult-only series; cortical dysplasia, hemimegencephaly, macrogyria, neuronal migration disorders, and ulegyric changes were part of the pediatric-only series; and heterotopia and tuberous sclerosis were seen in both.38,45,48,51,53,77
This group of pathologies does not demonstrate as high seizure-free rates as the other categories, and this can be attributed to the diffuse nature of developmental pathologies, which do not have distinct borders and often have more widespread subtle abnormalities in neuronal migration and neuronal activity throughout the brain.189 Seventy-five percent to 100% of patients with microgyria, 68% to 100% of patients with extratemporal cortical dysplasia, and 0% to 100% of those with tuberous sclerosis are seizure free postoperatively, all pathologies that can be more focal in manifestation, but only 50% of patients with macrogyria, 43% of patients with cytoarchitectural abnormalities, 0% to 43% of patients with hemimegencephaly, and 0% of those with nonspecific heterotopias become seizure free after epilepsy surgery in these surgical series.45,51,53,77,86,88 These findings agree with reports of patients having better seizure control after surgery for focal cortical lesions than for developmental abnormalities such as cortical dysplasia.50 In one epilepsy surgery series looking only at outcomes after resection of focal cortical dysplasia, there was a high seizure-free rate of 72%, whereas multilobar patients with more widespread disease had worse outcomes.190 However, another paper summarizing studies of surgery for malformations of cortical development reported a 2-year seizure-free rate of only 38% for extratemporal focal cortical dysplasia and just 34% for all extratemporal malformations of cortical development.191 The extent of resection for cortical dysplasia influences postoperative outcomes, with 80% of patients undergoing complete resection of the lesion being seizure free but only 20% with incomplete lesion resection being seizure free.192 A small series of pediatric epilepsy surgeries for tuberous sclerosis demonstrated only a 33% seizure-free rate.193
Inflammatory and Infectious Pathologies
The extratemporal epilepsy surgery series listed 2% to 17% with Rasmussen’s encephalitis, 2% to 6% with other encephalitides, 1% to 5% with inflammation, one case of tuberculoma for a 1% incidence, and one case of cysticercosis for a 1% incidence.41,43,45,50,54,75,86–88,94 The combined temporal and extratemporal series only reported encephalitis pathology in 1% to 17%.36,182 In exclusively adult and exclusively pediatric extratemporal lobe epilepsy surgery series, inflammatory pathologies were reported just in the pediatric-only series and consisted of cases of inflammation and Rasmussen’s encephalitis.45 Seizure-free outcomes from inflammatory and infectious causes were poor overall, with 0% to 55% with Rasmussen’s encephalitis being seizure free, 0% to 33% with encephalitis being seizure free, but complete seizure freedom for the patient with the tuberculoma.45,86,88
Normal Tissue
In general, surgical resections that yield tissue with no histopathologic abnormalities are a minority in epilepsy surgery. It may be that surgeons are less inclined to operate on normal-appearing substrates. Cryptogenic epilepsy surgical cases and cases producing normal resected tissue account for 2% to 43% of patients in extratemporal lobe epilepsy surgery series* and 1% to 15% of patients in combined temporal and extratemporal lobe epilepsy surgery series.39,46,69,94,182 In these series, arachnoid cysts, which we consider “normal” anomalies, were reported as causes in 1% to 5% of epilepsy patients.69,86 Cryptogenic and normal-tissue cases were seen in both the adult-only and the pediatric-only case series.38,45,77 Seizure-free rates for cryptogenic patients and those with no abnormal substrate were 25% to 100%,45,77,86,88 thus indicating that lack of cortical abnormality in an extratemporal epilepsy patient with good electrocorticographic localization of the focus should not deter the surgeon from attempting surgical intervention.
Evaluative Techniques for Extratemporal Lobe Epilepsy
Preoperative Evaluation
Video Electroencephalographic Monitoring
Modern imaging modalities often provide pivotal information for preoperative evaluation of patients for epilepsy surgery. EEG monitoring has therefore relinquished its role as the primary source of information on localization of the seizure focus in some cases. Despite the presence of a distinct lesion, it is nevertheless important to establish the relationship between a lesion seen on imaging and seizure onset. In some cases, the seizure focus is found to be distant from the lesion, and in rare cases, presumed epilepsy patients with space-occupying lesions were found to have nonepileptic seizures (i.e., pseudoseizures). At epilepsy centers, as many as 20% to 30% of patients admitted with a diagnosis of medically refractory seizures may actually have psychogenic nonepileptic seizures.194 EEG monitoring is especially important in patients with multiple structural lesions such as tuberous sclerosis or familial multiple cavernous angiomas, each of which could be the causative substrate underlying the epileptic syndrome. In some epilepsy patients, no focal abnormality is found on imaging studies and EEG monitoring remains the primary modality for localization of the epileptic focus. In many of these patients, invasive monitoring is necessary for precise localization. Newer techniques allowing three-dimensional localization of interictal spikes make interictal scalp EEG potentially more useful for localization of the seizure focus than is the case currently.195,196
Interictal EEG is a good tool for differentiating an epileptic patient from a nonepileptic patient in that 50% of epilepsy patients will have interictal abnormalities on one EEG, which increases to 92% on four EEGs, as opposed to a 0.4% incidence of spikes in the normal population.197 It has been argued that routine interictal EEG monitoring is frequently inadequate for accurate localization of the epileptogenic focus.198 However, interictal spike frequency has been a predictor of postoperative seizure-free outcome in patients with temporal lobe epilepsy.199 A study of interictal EEG for extratemporal epilepsy showed 100% concordance with subsequent surgical evaluation, including ictal and invasive recordings to localize the seizure focus to a specific lobe of origin.44 Another study comparing ictal and interictal EEG showed that interictal EEG localized the seizure onset to the appropriate lobe 67% of the time, similar to the ictal EEG rate of 68%200 and similar to rates in two other studies that showed ictal EEG localizing the seizure focus in 62% and 68% of patients.201,202 These findings aside, most epilepsy surgery centers rely heavily on video EEG to capture ictal EEG activity for localization of the epileptic focus.
Invasive Electrode Monitoring
Invasive monitoring with surgically implanted electrodes is frequently necessary for more accurate localization of the epileptic focus than can be provided by scalp EEG, especially with extratemporal lobe epilepsy.202 Depth electrodes have several contacts along their length and are surgically placed in specific locations within the brain parenchyma under frame-based or frameless stereotactic guidance. The use of multiplanar planning software has increased the accuracy of implantation of depth electrodes.203 Depth electrodes allow investigation of mesial, basal, and deep structures. They are especially helpful in the evaluation of mesial temporal lobe epilepsy syndromes or when patterns of spread from an extratemporal focus may involve the mesial temporal lobe rapidly.204 Examples would be distinguishing mesial temporal lobe epilepsy from orbitofrontal epilepsy or occipital lobe epilepsy because orbitofrontal and occipital seizures often spread rapidly to the temporal lobe.91,205 The use of depth electrodes in patients with suspected extratemporal lobe epilepsy is supported by a study showing that 25% of these patients will be found to have mesial temporal foci rather than extratemporal foci.206 Depth electrodes have a remarkably low complication rate, with a 2.4% infection rate, a 0.6% hemorrhage rate, no neurological deficits, and no deaths in one series in which there was an 85% rate of determining a surgically amenable seizure focus.207 In addition, new technologies combining microdialysis catheters with depth electodes208 may make cerebral microdialysis clinically significant for the surgical evaluation of intractable epilepsy patients in the future.209
Subdural grid and strip electrodes are surgically placed in direct apposition to the cortex, either through bur holes or by craniotomy. Grid electrodes are especially useful in extratemporal lobe epilepsy because they provide sampling of cortical activity over the cerebral convexities and make recording from more extensive territories possible.65 The advantage of subdural grids is that they enable detailed sampling of a cortical region in terms of seizure activity, along with functional mapping via electrical stimulation.210 This coverage can be essential in defining the anatomic extent of the more diffuse epileptic foci that are seen frequently in extratemporal lobe epilepsy. For the best of both worlds, some surgical centers have been using both subdural and depth electrodes together to evaluate patients with intractable epilepsy.211,212 Subdural electrodes alone appear to be preferable for the evaluation of frontal or multilobar seizure onset, whereas combined subdural and depth electrodes seem to be better for the evaluation of patients with dual pathology consisting of epileptic foci in both a neocortical region and the mesial temporal lobe.204 Frameless stereotactic systems facilitate combined placement of subdural and depth electrodes in the same patient by eliminating the need for a stereotactic frame, which may obstruct the approach for the craniotomy.213 The risk from limited subdural electrode studies is small, with one paper reporting a 0.85% rate of serious and minor complications in 350 patients,214 but the risk can be significant when using larger grids, where the complication rate was reported to be as high as 26.3%, mostly without permanent deficits but with a 0.5% mortality rate.215
Imaging
The most important imaging modality in preoperative planning for extratemporal epilepsy surgery is MRI.216 The advances in anatomic definition provided by modern MRI have contributed immensely to the identification of epileptic foci. The use of 3-T MRI and surface coils has made identification of a lesion underlying an epileptic focus 2.57 times more likely,6,217 and new quantitative techniques have made lesion characterization more accurate in terms of the underlying histopathology.218 Localized, well-circumscribed lesions on MRI, whether contrast-enhancing or signal abnormalities on T2-weighted or fluid-attenuated inversion recovery (FLAIR) images, delimit the target of resection, are associated with a higher occurrence of EEG focality,219 and signify a greater possibility for postoperative seizure control. FLAIR imaging can be particularly useful and should be part of the routine epilepsy MRI protocol.220,221 More diffuse lesions have a lower chance for postoperative seizure control,50 and the absence of a lesion on MRI denotes a poorer prognosis for seizure control with surgery (44% seizure free in one study).4,222,223 Three-dimensional reconstructions of CT and magnetic resonance angiographic images can now be fused with three-dimensional reconstructions of MRI in the frameless stereotactic system for more accurate representation of vascular structures in relation to cortical anatomy. Careful three-dimensional reconstructions of the cortical surface can reveal surface abnormalities associated with developmental lesions. Imaging of the brain with invasive monitors in place can also be fused with the preoperative MRI to provide exact localization and verification of electrode placement.224
Other imaging modalities have proved especially useful in the physiologic localization of epileptogenic regions in extratemporal lobe epilepsy. The first of these is fluorodeoxyglucose F 18 PET, which identifies areas of cortex with abnormal metabolic activity. Interictally epileptogenic zones tend to be hypometabolic relative to normal cortex.225,226 PET has proved most useful in the evaluation of mesial temporal lobe epilepsy,227 but it can also help delineate extratemporal epileptic foci and verify the likely epileptogenicity of structural lesions seen on MRI.228 Another imaging modality for delineation of epileptic foci is SPECT. In SPECT scanning, a trace amount of radioisotope is injected intravenously, and areas of increased blood flow are revealed. When used in the ictal state and compared with the interictal state, it may show a significant seizure focus in which enhanced neuronal activity results in increased blood flow.229,230 This technique has proved especially useful in tandem with coregistration to MRI (subtraction ictal SPECT coregistered to MRI [SISCOM]).231–233 Hypoperfusion on interictal SPECT can verify the epileptogenicity of certain foci identified on MRI, such as areas of cortical dysplasia.234 However, the disadvantage of ictal SPECT is that because of its inability to differentiate which region of activation occurred first, it can point to activated propagation pathways that do not need to be surgically removed to alleviate seizures.227 Although ictal SPECT has proved useful in the localization of extratemporal epileptic foci,228,235 interictal SPECT has not.236
Magnetic Source Imaging: Magnetoencephalography
Magnetic source imaging registered to MRI237 has proved to be a particularly effective tool for epilepsy surgery, especially extratemporal epilepsy (see Case Studies 61-1 to 61-4). MEG uses superconducting quantum interference device magnetometers to detect the magnetic fields associated with intraneuronal electrical currents. The magnetic field, which can indicate the epileptic focus, is not attenuated or distorted by the overlying skull and scalp as in scalp EEG, thus increasing the accuracy of localization without resorting to invasive EEG monitoring. MEG dipoles are registered to MRI by using fiducials, which allows structural localization in three dimensions. These images have proved useful in the localization of extratemporal lobe epileptic foci (see Case Study 61-1).237–239 Magnetic source imaging is most useful for neocortical epilepsy240 and correlates with intracranial recordings in the majority of cases.241 MEG can be helpful in identifying a seizure focus when MRI appears normal, and clustering of the MEG signal in the subsequently resected region can predict effective postoperative seizure control.242 In difficult cases requiring implantation of depth electrodes for evaluation of seizures, magnetic source imaging can be used to focus placement of the intracranial electrodes in a particular region suspected of epileptogenesis (see Case Study 61-3).
Another MRI modality showing promise in the localization of extratemporal seizure foci is MRS,243,244 which depicts the relative distributions of various chemicals in selected areas of the brain. MRS has been explored extensively for mesial temporal lobe epilepsy but has also been used in studies of extratemporal lobe epilepsy.245 Extratemporal epileptic foci have shown increased pH, decreased phosphomonoesters,246 decreased N-acetylaspartate (NAA), and decreased NAA-to-creatine and NAA-to-choline ratios.243,247–249 MRS is another imaging modality that can be used to localize extratemporal seizure foci in patients with negative MRI findings,249 and with further development it may enjoy wider use by clinicians.
DTI, a technique used to evaluate white matter tract projections in the brain, has been investigated as a potential imaging modality for determining seizure foci in traditional MRI-negative epilepsy syndromes. DTI has shown changes in the brain that indicate neocortical seizure foci5 but does not yet have the reliability to be used clinically for determination of seizure foci except in patients with tuberous sclerosis, in whom DTI was more accurate than structural MRI in localizing the epileptogenic cortex.250
fMRI is a maturing imaging technology that provides information on specific activation of cortical processing zones during motor, sensory, and cognitive functions. This modality is used as a preoperative tool to define the relationship of cortical functional anatomy and the epileptogenic zone.72 fMRI is useful in the delineation of functional cortical anatomy for frontal lobe epilepsy resections that include motor and speech areas.251 Although the clinical utility of fMRI activation outside the rolandic cortex is controversial,252 it provides a noninvasive preliminary to intraoperative mapping that can avoid craniotomy in patients in whom the deficits would be unacceptable. It is useful in determining hemispheric language dominance and it correlates well with results obtained by the Wada test.253,254 In addition to its role in defining functional cortical zones, fMRI is developing as a tool to localize interictal epileptiform discharges. Simultaneous EEG recording and event-related fMRI allow the linking of epileptiform activity with local hemodynamic changes in the brain.255–260 On EEG-fMRI, a blood oxygen level–dependent (BOLD) effect corresponded to spiking in the temporal lobe in the majority of temporal lobe epilepsy patients, but there was also activation in other brain regions that was thought to represent activation of networks associated with the epileptic activity generated at the focus in the medial temporal lobe.261 In addition, novel approaches involving quantitative methodologies in fMRI hold promise for localizing epileptiform neural activity in the future.262
Neuropsychological Testing
Careful neuropsychological testing can provide information concerning the impact of the epileptic focus on neurological function in the region of the focus.263 Thus, it can be judiciously used as a confirmative tool in the presurgical evaluation. Preoperative neuropsychological testing is important to describe the current cognitive and behavioral functioning in the context of the seizure disorder and underlying neuropathology, to start interventions for cognitive and behavioral difficulties if present, and for postoperative prognosis, both for seizure-free outcome and for neuropsychological deficits.263–265 This testing can be especially important in patients with frontal lobe epilepsy because specific abnormalities can become evident on testing, even though routine neurological examination appears normal. Frontal lobe epilepsy patients with either preoperative or postoperative deficits have difficulty with mental flexibility, planning, and fluency (verbal fluency from the left frontal lobe and nonverbal fluency from the right frontal lobe). Parietal lobe deficits are uncommon except in patients with extensive parietal lesions. Distortion in copying a complex figure representing distortion in conceptualization of spatial representation can be associated with parietal lobe lesions, right more than left, and somatosensory tests sometimes show differences in these patients. Occipital lobe lesions may be manifested as visual field deficits, and if significant, they may interfere with cognitive visual tests. Lateral occipital defects have profound cognitive effects on visual cognitive tasks only if they are bilateral, although unilateral occipitotemporal lesions may be accompanied by material-specific neurocognitive deficits, depending on the hemisphere involved. Some epilepsy surgery centers use neuropsychological testing to aid in localization and lateralization,266 although neuropsychological findings alone are not reliable for the lateralization of seizure foci.267
The other important preoperative neuropsychological test commonly used is the intracarotid amobarbital procedure, or the Wada test.268,269 The patient’s language and memory functions are tested during injection of a barbiturate into one cerebral hemisphere to block function on that side, and the test is sometimes repeated with injection on the other side. A review of 1421 Wada tests suggested that the test has high reliability and validity for language lateralization but does not have as high reliability or validity for memory lateralization.270 The memory component of the test is used to determine the safety of resection of medial temporal lobe tissue for the treatment of medial temporal lobe epilepsy. For extratemporal epilepsy, the memory part of the Wada test is less important. Establishing the side of language dominance is crucial, however, in planning frontal lobe and parietal lobe epilepsy surgery. Developments in fMRI hold promise in using this noninvasive modality to determine language hemispheric dominance,271 thereby potentially obviating the need for the more invasive amobarbital procedure, which requires angiography and administration of amobarbital.
Intraoperative Evaluation
Intraoperative Mapping
It is often necessary to evaluate localization of functions such as language and movement in patients who are undergoing extratemporal lobe resection for seizures. Preoperative fMRI and other preoperative imaging modalities can be useful in providing a general impression of these functional regions in relation to the proposed site of resection.272 fMRI is still under development, however, and thus most surgeons perform the “gold standard” intraoperative electrical stimulation mapping for proposed resections near regions of motor, sensory, or language cortex. In patients for whom EEG monitoring with grid electrodes is indicated for localizing the seizure focus, extraoperative mapping is performed on the ward.
For intraoperative language18 or sensory273 mapping, the patient needs to be fully conscious and able to cooperate. For motor mapping, patient cooperation is not required, and the procedure can be carried out under general, albeit light and nonparalytic anesthesia. In fact, there is some evidence that near a tumor, cortical stimulation motor mapping is inaccurate.274 Evoked potential sensorimotor mapping can be performed with the patient under general anesthesia. To perform evoked potential sensorimotor mapping, the median nerve at the hand is stimulated with a small electrical current, and somatosensory evoked potentials are recorded from the cortical surface over the suspected rolandic cortex. Phase reversal of the N20 median nerve somatosensory evoked potential from one strip electrode lead to the next defines the central sulcus, with the precentral motor area being anterior and the postcentral sensory area being posterior. This process indirectly identifies the motor cortex and does not provide the detailed map offered by electrical stimulation mapping.
When patient cooperation is required, the operation is performed under local anesthesia, whereas intravenous sedation is used during periods of the procedure when the patient’s cooperation is not required.275 Awake craniotomy can be performed fairly routinely with few complications.276 Agents such as propofol have greatly improved the ability to carry out such procedures with reasonable patient comfort. An alternative method, which we often use, is asleep-awake-asleep anesthesia,277 in which the procedure is started with general anesthesia and intubation to enable the routine neurosurgical craniotomy protocol, including cranial fixation and image-guided surgery. Only at the stage of the procedure when mapping is required is the patient allowed to emerge from general anesthesia and subsequently extubated, with a small guide left in place through the vocal cords. After mapping, the patient can be reintubated and fully anesthetized. Electrical stimulation mapping or other patient testing can be carried out throughout the resection if the resection is close to functional processing zones. Intraoperative mapping can be especially important given the wide variability in the location of language areas.18,278
Intraoperative Electrocorticography
Extratemporal lobe epilepsy surgery requires extensive preoperative testing to localize and define the seizure focus as discussed earlier. Even with this significant preoperative evaluation, it is frequently necessary to perform intraoperative electrocorticography to define the seizure focus further and plan the extent of resection. To perform electrocorticography, grid electrodes of various sizes and configurations or individual electrodes are placed directly on the exposed cerebral cortex over suspected regions of epileptogenesis, and the electrical activity is recorded. Anesthesia is lightened as much as possible during electrocorticography so that epileptic activity is not suppressed. General anesthesia can suppress spike frequency279; however, propofol sedation280 and dexmedetomidine sedation281,282 can be used during electrocorticography. Spike activity is usually taken as an indication of an epileptogenic zone, but sometimes severe slowing of activity is also considered. After tissue resection, electrocorticography can be performed around the borders of the resection cavity to verify the elimination of pathologic electrical activity. The utility of electrocorticography in guiding extratemporal epilepsy surgery resection is controversial, with some clinicians arguing that it is not necessary in many situations185 and others presenting some evidence that it may help increase the chance of postoperative freedom from seizures.4,283–285
Frameless Stereotaxy
The use of frameless stereotactic equipment in neurosurgery has become widespread in all areas, including epilepsy surgery,286 and it is especially useful in extratemporal lobe epilepsy surgery. As discussed earlier, extratemporal lobe epilepsy has a better prognosis for postoperative seizure control if a structural lesion can be identified on imaging as the seizure focus. This improved prognosis depends on the ability to resect the lesion completely at the time of surgery. Complete resection can be especially difficult for some pathologic lesions underlying extratemporal lobe epilepsy, such as cortical dysplasia, because they frequently appear similar to normal brain tissue and do not have distinct borders. Frameless stereotactic systems can guide the surgeon directly to the lesion and help determine the borders of the lesion based on the imaging.287
Frameless stereotaxy is also useful in cases of nonlesional extratemporal lobe epilepsy. Many of the evaluation modalities described previously, such as MEG and SPECT, can be combined with the three-dimensional images to help guide surgery. CT or MRI with invasive electrodes in place can be fused with preoperative MRI to help localize the epileptogenic tissue more accurately during surgery.288 fMRI can be added to suggest functional regions that may require extensive stimulation mapping. Frameless stereotaxy has proved very useful in other epilepsy surgeries as well, including corpus callosotomy129 and the placement of intracranial electrodes.213 The use of frameless stereotaxy in extratemporal lobe epilepsy holds considerable promise in improving the safety and efficacy of surgery.
New Modalities in Development
Novel intraoperative techniques are being developed that should prove useful in extratemporal lobe epilepsy surgery. One of these techniques is intraoperative MRI, which has been used in epilepsy surgery mainly for temporal lobe epilepsy,289–291 although it is applicable to extratemporal lobe epilepsy as well. This technology has undergone considerable improvement in being compatible with the operating room environment. In its application to epilepsy surgery, it is especially helpful because it enables the use of various sequences intraoperatively, including FLAIR sequences, which are sensitive to the subtle tissue changes sometimes seen in epileptogenic lesions. With larger resections, especially those involving multiple lobes, brain shift poses difficulties for traditional image-guided navigation. Interventional MRI allows intraoperative evaluation of the extent of resection without the confounding effect of brain shift.
Intraoperative optical imaging of intrinsic signals (iOIS) is another technique under development that should prove useful during surgical resection of extratemporal epileptic foci.292,293 The equipment is attached to the microscope and allows detection of cortical regions that are active during specific activities, such as hand movement and language. Intraoperative studies using this technique suggest that iOIS provides more detailed information about cortical function than stimulation mapping,294 and functions localized by iOIS seem to correspond to localization by fMRI.295 One study has shown that this procedure can be used to localize seizure foci intraoperatively as well.296
Asenbaum S, Baumgartner C. Nuclear medicine in the preoperative evaluation of epilepsy. Nucl Med Commun. 2001;22:835-840.
Berger MS, Ghatan S, Haglund MM, et al. Low-grade gliomas associated with intractable epilepsy: seizure outcome utilizing electrocorticography during tumor resection. J Neurosurg. 1993;79:62-69.
Cook SW, Nguyen ST, Hu B, et al. Cerebral hemispherectomy in pediatric patients with epilepsy: comparison of three techniques by pathological substrate in 115 patients. J Neurosurg. 2004;100:125-141.
Duchowny MS, Harvey AS, Sperling MR. Indications and criteria for surgical intervention. In: Engel JJr, Pedley TA, editors. Epilepsy: A Comprehensive Textbook. Philadelphia: Lippincott-Raven; 1997:1677-1685.
Fried I. Magnetic resonance imaging and epilepsy: neurosurgical decision making. Magn Reson Imaging. 1995;13:1163-1170.
Fried I. Management of low-grade gliomas: results of resections without electrocorticography. Clin Neurosurg. 1995;42:453-463.
Fried I, Wilson CL, Maidment NT, et al. Cerebral microdialysis combined with single-neuron and electroencephalographic recording in neurosurgical patients. Technical note. J Neurosurg. 1999;91:697-705.
Hendler T, Pianka P, Sigal M, et al. Delineating gray and white matter involvement in brain lesions: three-dimensional alignment of functional magnetic resonance and diffusion-tensor imaging. J Neurosurg. 2003;99:1018-1027.
Hoh BL, Chapman PH, Loeffler JS, et al. Results of multimodality treatment for 141 patients with brain arteriovenous malformations and seizures: factors associated with seizure incidence and seizure outcomes. Neurosurgery. 2002;51:303-309.
Janjua FN, Eliashiv DS, Fried I. The added value of magnetic source imaging in a large series of medically refractory partial epilepsy patients that are candidates for epilepsy surgery. Epilepsia. 2003;44(suppl 9):251.
Jayakar P, Dunoyer C, Dean P, et al. Epilepsy surgery in patients with normal or nonfocal MRI scans: integrative strategies offer long-term seizure relief. Epilepsia. 2008;49:758-764.
Jobst BC, Velasco F, Lado F, et al. Brain stimulation for the treatment of epilepsy: What is the evidence in humans and animals [abstract 1W.27]. Epilepsia. 2008;49:176-177.
Jonas R, Nguyen S, Hu B, et al. Cerebral hemispherectomy: hospital course, seizure, developmental, language, and motor outcomes. Neurology. 2004;62:1712-1721.
Mamelak AN, Lopez N, Akhtari M, et al. Magnetoencephalography-directed surgery in patients with neocortical epilepsy. J Neurosurg. 2002;97:865-873.
McGonigal A, Bartolomei F, Regis J, et al. Stereoelectroencephalography in presurgical assessment of MRI-negative epilepsy. Brain. 2007;130:3169-3183.
O’Brien TJ, So EL, Mullan BP, et al. Subtraction peri-ictal SPECT is predictive of extratemporal epilepsy surgery outcome. Neurology. 2000;55:1668-1677.
Olivier A, Awad IA. Extratemporal resections. In: Engel JJr, editor. Surgical Treatment of the Epilepsies. New York: Raven Press; 1993:489-500.
Spencer S, Huh L. Outcomes of epilepsy surgery in adults and children. Lancet Neurol. 2008;7:525-537.
Spencer SS, Berg AT, Vickrey BG, et al. Predicting long-term seizure outcome after resective epilepsy surgery: the multicenter study. Neurology. 2005;65:912-918.
Spencer SS, Schramm J, Wyler A, et al. Multiple subpial transection for intractable partial epilepsy: an international meta-analysis. Epilepsia. 2002;43:141-145.
Sporis D, Hajnsek S, Boban M, et al. Epilepsy due to malformations of cortical development—correlation of clinical, MRI and Tc-99mECD SPECT findings. Coll Antropol. 2008;32:345-350.
Téllez-Zenteno JF, Dhar R, Wiebe S. Long-term seizure outcomes following epilepsy surgery: a systematic review and meta-analysis. Brain. 2005;128:1188-1198.
Toczek MT, Morrell MJ, Risinger MW, et al. Intracranial ictal recordings in mesial frontal lobe epilepsy. J Clin Neurophysiol. 1997;14:499-506.
Williamson PD, Thadani VM, Darcey TM, et al. Occipital lobe epilepsy: clinical characteristics, seizure spread patterns, and results of surgery. Ann Neurol. 1992;31:3-13.
1 Duchowny MS, Harvey AS, Sperling MR. Indications and criteria for surgical intervention. In: Engel JJr, Pedley TA, editors. Epilepsy: A Comprehensive Textbook. Philadelphia: Lippincott-Raven; 1997:1677-1685.
2 Engel JJr. Introduction to temporal lobe epilepsy. Epilepsy Res. 1996;26:141-150.
3 Fried I. Anatomic temporal lobe resections for temporal lobe epilepsy. Neurosurg Clin N Am. 1993;4:233-242.
4 Jayakar P, Dunoyer C, Dean P, et al. Epilepsy surgery in patients with normal or nonfocal MRI scans: integrative strategies offer long-term seizure relief. Epilepsia. 2008;49:758-764.
5 Chen Q, Lui S, Li CX, et al. MRI-negative refractory partial epilepsy: role for diffusion tensor imaging in high field MRI. Epilepsy Res. 2008;80:83-89.
6 Strandberg M, Larsson EM, Backman S, et al. Pre-surgical epilepsy evaluation using 3T MRI. Do surface coils provide additional information? Epileptic Disord. 2008;10:83-92.
7 Mischel PS, Vinters HV. Neuropathology. Neurosurg Clin N Am. 1995;6:565-579.
8 Binnie CD. Vagus nerve stimulation for epilepsy: a review. Seizure. 2000;9:161-169.
9 Loddenkemper T, Pan A, Neme S, et al. Deep brain stimulation in epilepsy. J Clin Neurophysiol. 2001;18:514-532.
10 Fisher RS, Uematsu S, Krauss GL, et al. Placebo-controlled pilot study of centromedian thalamic stimulation in treatment of intractable seizures. Epilepsia. 1992;33:841-851.
11 Jobst BC, Velasco F, Lado F, et al. Brain stimulation for the treatment of epilepsy: what is the evidence in humans and animals [abstract 1W.27]. Epilepsia. 2008;49:176-177.
12 Penfield W, Jasper H. Epilepsy and the Functional Anatomy of the Brain. Boston: Little, Brown; 1954.
13 Babb TL, Brown WJ, Pretorius J, et al. Temporal lobe volumetric cell densities in temporal lobe epilepsy. Epilepsia. 1984;25:729-740.
14 Babb TL, Lieb JP, Brown WJ, et al. Distribution of pyramidal cell density and hyperexcitability in the epileptic human hippocampal formation. Epilepsia. 1984;25:721-728.
15 Kim JH, Guimaraes PO, Shen MY, et al. Hippocampal neuronal density in temporal lobe epilepsy with and without gliomas. Acta Neuropathol. 1990;80:41-45.
16 Babb TL. Bilateral pathological damage in temporal lobe epilepsy. Can J Neurol Sci. 1991;18:645-648.
17 Spencer DD, Spencer SS, Mattson RH, et al. Access to the posterior medial temporal lobe structures in the surgical treatment of temporal lobe epilepsy. Neurosurgery. 1984;15:667-671.
18 Ojemann G, Ojemann J, Lettich E, et al. Cortical language localization in left, dominant hemisphere. An electrical stimulation mapping investigation in 117 patients. J Neurosurg. 1989;71:316-326.
19 Ojemann GA. Interplay between “neocortical” and “limbic” temporal lobe epilepsy. Adv Neurol. 2000;84:615-619.
20 Niemeyer P. The transventricular amygdala-hippocampectomy in temporal lobe epilepsy. In: Baldwin M, Bailey P, editors. Temporal Lobe Epilepsy. Springfield, IL: Charles C Thomas; 1958:461-482.
21 Yasargil MG, Wieser HG, Valavanis A, et al. Surgery and results of selective amygdala-hippocampectomy in one hundred patients with nonlesional limbic epilepsy. Neurosurg Clin N Am. 1993;4:243-261.
22 Patil SG, Cross JH, Kling Chong W, et al. Is streamlined evaluation of children for epilepsy surgery possible? Epilepsia. 2008;49:1340-1347.
23 Spencer S, Huh L. Outcomes of epilepsy surgery in adults and children. Lancet Neurol. 2008;7:525-537.
24 Téllez-Zenteno JF, Dhar R, Wiebe S. Long-term seizure outcomes following epilepsy surgery: a systematic review and meta-analysis. Brain. 2005;128:1188-1198.
25 Wiebe S, Blume WT, Girvin JP, et al. A randomized, controlled trial of surgery for temporal-lobe epilepsy. N Engl J Med. 2001;345:311-318.
26 Tanriverdi T, Olivier A, Poulin N, et al. Long-term seizure outcome after mesial temporal lobe epilepsy surgery: corticalamygdalohippocampectomy versus selective amygdalohippocampectomy. J Neurosurg. 2008;108:517-524.
27 Spencer SS, Berg AT, Vickrey BG, et al. Initial outcomes in the Multicenter Study of Epilepsy Surgery. Neurology. 2003;61:1680-1685.
28 Spencer SS, Berg AT, Vickrey BG, et al. Predicting long-term seizure outcome after resective epilepsy surgery: the multicenter study. Neurology. 2005;65:912-918.
29 Behrens E, Schramm J, Zentner J, et al. Surgical and neurological complications in a series of 708 epilepsy surgery procedures. Neurosurgery. 1997;41:1-9.
30 Engel JJr, Wiebe S, French J, et al. Practice parameter: temporal lobe and localized neocortical resections for epilepsy: report of the Quality Standards Subcommittee of the American Academy of Neurology, in association with the American Epilepsy Society and the American Association of Neurological Surgeons. Neurology. 2003;60:538-547.
31 Vickrey BG, Hays RD, Rausch R, et al. Outcomes in 248 patients who had diagnostic evaluations for epilepsy surgery. Lancet. 1995;346:1445-1449.
32 Sabaz M, Lawson JA, Cairns DR, et al. The impact of epilepsy surgery on quality of life in children. Neurology. 2006;66:557-561.
33 Devinsky O, Barr WB, Vickrey BG, et al. Changes in depression and anxiety after resective surgery for epilepsy. Neurology. 2005;65:1744-1749.
34 Milner B. Psychological aspects of focal epilepsy and its neurosurgical management. Adv Neurol. 1975;8:299-321.
35 Chin PS, Berg AT, Spencer SS, et al. Patient-perceived impact of resective epilepsy surgery. Neurology. 2006;66:1882-1887.
36 Eriksson S, Malmgren K, Rydenhag B, et al. Surgical treatment of epilepsy—clinical, radiological and histopathological findings in 139 children and adults. Acta Neurol Scand. 1999;99:8-15.
37 Dodrill CB, Wilkus RJ, Ojemann GA, et al. Multidisciplinary prediction of seizure relief from cortical resection surgery. Ann Neurol. 1986;20:2-12.
38 Maehara T, Shimizu H, Oda M, et al. Surgical treatment of children with medically intractable epilepsy—outcome of various surgical procedures. Neurol Med Chir (Tokyo). 1996;36:305-309.
39 Munari C, Berta E, Tassi L, et al. Analysis of failures and reoperations in resective epilepsy surgery. Adv Neurol. 2000;84:605-614.
40 Rasmussen T. Tailoring of cortical excisions for frontal lobe epilepsy. Can J Neurol Sci. 1991;18:606-610.
41 Rasmussen T. Surgery for central, parietal and occipital epilepsy. Can J Neurol Sci. 1991;18:611-616.
42 Zentner J, Hufnagel A, Ostertun B, et al. Surgical treatment of extratemporal epilepsy: clinical, radiologic, and histopathologic findings in 60 patients. Epilepsia. 1996;37:1072-1080.
43 Adler J, Erba G, Winston KR, et al. Results of surgery for extratemporal partial epilepsy that began in childhood. Arch Neurol. 1991;48:133-140.
44 Holmes MD, Kutsy RL, Ojemann GA, et al. Interictal, unifocal spikes in refractory extratemporal epilepsy predict ictal origin and postsurgical outcome. Clin Neurophysiol. 2000;111:1802-1808.
45 Mathern GW, Giza CC, Yudovin S, et al. Postoperative seizure control and antiepileptic drug use in pediatric epilepsy surgery patients: the UCLA experience, 1986-1997. Epilepsia. 1999;40:1740-1749.
46 Paolicchi JM, Jayakar P, Dean P, et al. Predictors of outcome in pediatric epilepsy surgery. Neurology. 2000;54:642-647.
47 Prats AR, Morrison G, Wolf AL. Focal cortical resections for the treatment of extratemporal epilepsy in children. Neurosurg Clin N Am. 1995;6:533-540.
48 Wyllie E, Comair YG, Kotagal P, et al. Seizure outcome after epilepsy surgery in children and adolescents. Ann Neurol. 1998;44:740-748.
49 Khajavi K, Comair YG, Wyllie E, et al. Surgical management of pediatric tumor-associated epilepsy. J Child Neurol. 1999;14:15-25.
50 Leiphart JW, Peacock WJ, Mathern GW. Lobar and multilobar resections for medically intractable pediatric epilepsy. Pediatr Neurosurg. 2001;34:311-318.
51 Wyllie E, Comair YG, Kotagal P, et al. Epilepsy surgery in infants. Epilepsia. 1996;37:625-637.
52 Duchowny MS, Resnick TJ, Alvarez LA, et al. Focal resection for malignant partial seizures in infancy. Neurology. 1990;40:980-984.
53 Cascino GD, Hulihan JF, Sharbrough FW, et al. Parietal lobe lesional epilepsy: electroclinical correlation and operative outcome. Epilepsia. 1993;34:522-527.
54 Aykut-Bingol C, Bronen RA, Kim JH, et al. Surgical outcome in occipital lobe epilepsy: implications for pathophysiology. Ann Neurol. 1998;44:60-69.
55 Jackson JH. On the anatomical and physiological localization in the brain. Lancet. 1873;1:84-85.
56 Horsley V. The motor cortex of the brain and the mechanism of the will. BMJ. 1885;1:111-115.
57 Horsley V. Ten consecutive cases of operation upon the brain and cranial cavity to illustrate the details and safety of the method employed. BMJ. 1887;1:863-865.
58 Horsley V. The Linacre lecture on the so-called motor area of the brain. BMJ. 1909;2:125-137.
59 Keen WW. Three successful cases of cerebral surgery. Am J Med Sci. 1888;96:330-365.
60 Lloyd HJ, Deaver JB. A case of focal epilepsy successfully treated by trephining and excision of the motor cortex. Am J Med Sci. 1888;96:471-481.
61 Ajmone-Marsan C, Ralston BL. The Epileptic Seizure: Its Functional Morphology and Diagnosis Significance: A Clinical-Electrographic Analysis of Metrazol Induced Attacks. Springfield, IL: Charles C Thomas; 1957.
62 Chauvel P, Trottier S, Vignal JP, et al. Somatomotor seizures of frontal lobe origin. Adv Neurol. 1992;57:185-232.
63 Bancaud J, Talairach J. Clinical semiology of frontal lobe seizures. Adv Neurol. 1992;57:3-58.
64 Williamson PD, Engel JJr, Munari C. Anatomic classification of localization-related epilepsies. In: Engel JJr, Pedley TA, editors. Epilepsy: A Comprehensive Textbook. Philadelphia: Lippincott-Raven; 1997:2405-2416.
65 Toczek MT, Morrell MJ, Risinger MW, et al. Intracranial ictal recordings in mesial frontal lobe epilepsy. J Clin Neurophysiol. 1997;14:499-506.
66 Bleasel A, Kotagal P, Kankirawatana P, et al. Lateralizing value and semiology of ictal limb posturing and version in temporal lobe and extratemporal epilepsy. Epilepsia. 1997;38:168-174.
67 Salanova V, Quesney LF, Rasmussen T, et al. Reevaluation of surgical failures and the role of reoperation in 39 patients with frontal lobe epilepsy. Epilepsia. 1994;35:70-80.
68 Williamson PD, Mattson RH, Spencer SS, et al. Complex partial seizures of frontal lobe origin: problems with diagnosis and management. Epilepsia. 1983;24:516.
69 Lehman R, Andermann F, Olivier A, et al. Seizures with onset in the sensorimotor face area: clinical patterns and results of surgical treatment in 20 patients. Epilepsia. 1994;35:1117-1124.
70 Olivier A, Awad IA. Extratemporal resections. In: Engel JJr, editor. Surgical Treatment of the Epilepsies. New York: Raven Press; 1993:489-500.
71 Penfield W, Rasmussen T. A clinical Study of Localization of Function: The Cerebral Cortex of Man. New York: MacMillan; 1950.
72 Gaillard WD, Bookheimer SY, Cohen M. The use of fMRI in neocortical epilepsy. Adv Neurol. 2000;84:391-404.
73 Hendler T, Pianka P, Sigal M, et al. Delineating gray and white matter involvement in brain lesions: three-dimensional alignment of functional magnetic resonance and diffusion-tensor imaging. J Neurosurg. 2003;99:1018-1027.
74 Mosewich RK, So EL, O’Brien TJ, et al. Factors predictive of the outcome of frontal lobe epilepsy surgery. Epilepsia. 2000;41:843-849.
75 Janszky J, Jokeit H, Schulz R, et al. EEG predicts surgical outcome in lesional frontal lobe epilepsy. Neurology. 2000;54:1470-1476.
76 Lee S, Vives K, Westerveld M, et al. Quantitative temporal lobe volumetrics in the surgical management of nonlesional frontal and temporal neocortical epilepsies. Adv Neurol. 2000;84:577-593.
77 Jobst BC, Siegel AM, Thadani VM, et al. Intractable seizures of frontal lobe origin: clinical characteristics, localizing signs, and results of surgery. Epilepsia. 2000;41:1139-1152.
78 Schramm J, Kral T, Blumcke I, et al. Surgery for neocortical temporal and frontal epilepsy. Adv Neurol. 2000;84:595-603.
79 Elsharkawy AE, Behne F, Oppel F, et al. Long-term outcome of extratemporal epilepsy surgery among 154 adult patients. J Neurosurg. 2008;108:676-686.
80 Ajmone-Marsan C, Goldhammer L. Clinical patterns and electrographic data in cases of partial seizures of frontal-central-parietal origin. In: Brazier MAB, editor. Epilepsy, Its Phenomena in Man. New York: Academic Press; 1973:235-258.
81 Williamson PD, Boon PA, Thadani VM, et al. Parietal lobe epilepsy: diagnostic considerations and results of surgery. Ann Neurol. 1992;31:193-201.
82 Niedermeyer E. Special types of epilepsy according to the site of focus. In: Niedermeyer E, editor. Compendium of the Epilepsies. Springfield, IL: Charles C Thomas; 1974:106-144.
83 Foerster O, Penfield W. The structural basis of traumatic epilepsy and results of radical operation. Brain. 1930;53:99-119.
84 Penfield W, Gage L. Cerebral localization of epileptic manifestations. Arch Neurol Psychiatry. 1933;30:709-727.
85 Penfield W, Erickson TC. Epilepsy and Cerebral Localization. Baltimore: Charles C Thomas; 1941.
86 Salanova V, Andermann F, Rasmussen T, et al. Parietal lobe epilepsy. Clinical manifestations and outcome in 82 patients treated surgically between 1929 and 1988. Brain. 1995;118:607-627.
87 Olivier A, Boling WJr. Surgery of parietal and occipital lobe epilepsy. Adv Neurol. 2000;84:533-575.
88 Salanova V, Andermann F, Olivier A, et al. Occipital lobe epilepsy: electroclinical manifestations, electrocorticography, cortical stimulation and outcome in 42 patients treated between 1930 and 1991. Surgery of occipital lobe epilepsy. Brain. 1992;115:1655-1680.
89 Williamson PD, Thadani VM, Darcey TM, et al. Occipital lobe epilepsy: clinical characteristics, seizure spread patterns, and results of surgery. Ann Neurol. 1992;31:3-13.
90 Huott AD, Madison DS, Niedermeyer E. Occipital lobe epilepsy. A clinical and electroencephalographic study. Eur Neurol. 1974;11:325-339.
91 Olivier A, Gloor P, Andermann F, et al. Occipitotemporal epilepsy studied with stereotaxically implanted depth electrodes and successfully treated by temporal resection. Ann Neurol. 1982;11:428-432.
92 Aldrich MS, Vanderzant CW, Alessi AG, et al. Ictal cortical blindness with permanent visual loss. Epilepsia. 1989;30:116-120.
93 Caicoya AG, Macarron J, Albisua J, et al. Tailored resections in occipital lobe epilepsy surgery guided by monitoring with subdural electrodes: characteristics and outcome. Epilepsy Res. 2007;77:1-10.
94 Munari C, Francione S, Kahane P, et al. Multilobar resections for the control of epilepsy. In: Schmidek HH, editor. Operative Neurosurgical Techniques. Philadelphia: WB Saunders; 2000:1473-1489.
95 Schramm J. Hemispherectomy techniques. Neurosurg Clin N Am. 2002;13:113-134. ix
96 De Almeida AN, Marino RJr, Aguiar PH, et al. Hemispherectomy: a schematic review of the current techniques. Neurosurg Rev. 2006;29:97-102.
97 Peacock WJ. Hemispherectomy for the treatment of intractable seizures in childhood. Neurosurg Clin N Am. 1995;6:549-563.
98 Fountas KN, Smith JR, Robinson JS, et al. Anatomical hemispherectomy. Childs Nerv Syst. 2006;22:982-991.
99 Villemure JG, Vernet O, Delalande O. Hemispheric disconnection: callosotomy and hemispherotomy. Adv Tech Stand Neurosurg. 2000;26:25-78.
100 Villemure JG, Mascott CR. Peri-insular hemispherotomy: surgical principles and anatomy. Neurosurgery. 1995;37:975-981.
101 Schramm J, Behrens E, Entzian W. Hemispherical deafferentation: an alternative to functional hemispherectomy. Neurosurgery. 1995;36:509-515.
102 Delalande O, Pinard JM, Basdevant C, et al. Hemispherotomy: a new procedure for central disconnection. Epilepsia. 1992;33(suppl 3):99-100.
103 Binder DK, Schramm J. Transsylvian functional hemispherectomy. Childs Nerv Syst. 2006;22:960-966.
104 Kossoff EH, Vining EP, Pillas DJ, et al. Hemispherectomy for intractable unihemispheric epilepsy etiology vs outcome. Neurology. 2003;61:887-890.
105 Jonas R, Nguyen S, Hu B, et al. Cerebral hemispherectomy: hospital course, seizure, developmental, language, and motor outcomes. Neurology. 2004;62:1712-1721.
106 Daniel RT, Joseph TP, Gnanamuthu C, et al. Hemispherotomy for paediatric hemispheric epilepsy. Stereotact Funct Neurosurg. 2001;77:219-222.
107 Smith JR, Lee MR, Jenkins PD, et al. A 13-year experience with epilepsy surgery. Stereotact Funct Neurosurg. 1999;73:98-103.
108 Cook SW, Nguyen ST, Hu B, et al. Cerebral hemispherectomy in pediatric patients with epilepsy: comparison of three techniques by pathological substrate in 115 patients. J Neurosurg. 2004;100:125-141.
109 Wyler AR. Multiple subpial transections in neocortical epilepsy: part II. Adv Neurol. 2000;84:635-642.
110 Smith MC, Byrne R. Multiple subpial transection in neocortical epilepsy: part I. Adv Neurol. 2000;84:621-634.
111 Blount JP, Langburt W, Otsubo H, et al. Multiple subpial transections in the treatment of pediatric epilepsy. J Neurosurg. 2004;100:118-124.
112 Wyler AR, Wilkus RJ, Rostad SW, et al. Multiple subpial transections for partial seizures in sensorimotor cortex. Neurosurgery. 1995;37:1122-1127.
113 Devinsky O, Perrine K, Vazquez B, et al. Multiple subpial transections in the language cortex. Brain. 1994;117:255-265.
114 Spencer SS, Schramm J, Wyler A, et al. Multiple subpial transection for intractable partial epilepsy: an international meta-analysis. Epilepsia. 2002;43:141-145.
115 Mulligan LP, Spencer DD, Spencer SS. Multiple subpial transections: the Yale experience. Epilepsia. 2001;42:226-229.
116 Vaz G, van Raay Y, van Rijckevorsel K, et al. [Safety and efficacy of multiple subpial transections: report of a consecutive series of 30 cases.]. Neurochirurgie. 2008;54:311-314.
117 Devinsky O, Romanelli P, Orbach D, et al. Surgical treatment of multifocal epilepsy involving eloquent cortex. Epilepsia. 2003;44:718-723.
118 Schramm J, Aliashkevich AF, Grunwald T. Multiple subpial transections: outcome and complications in 20 patients who did not undergo resection. J Neurosurg. 2002;97:39-47.
119 Rougier A, Sundstrom L, Claverie B, et al. Multiple subpial transection: report of 7 cases. Epilepsy Res. 1996;24:57-63.
120 Orbach D, Romanelli P, Devinsky O, et al. Late seizure recurrence after multiple subpial transections. Epilepsia. 2001;42:1130-1133.
121 Madsen JR, Carmant L, Holmes GL, et al. Corpus callosotomy in children. Neurosurg Clin N Am. 1995;6:541-548.
122 Kwan SY, Wong TT, Chang KP, et al. Seizure outcome after corpus callosotomy: the Taiwan experience. Childs Nerv Syst. 2000;16:87-92.
123 Fuiks KS, Wyler AR, Hermann BP, et al. Seizure outcome from anterior and complete corpus callosotomy. J Neurosurg. 1991;74:573-578.
124 Turanli G, Yalnizoglu D, Genc-Acikgoz D, et al. Outcome and long term follow-up after corpus callosotomy in childhood onset intractable epilepsy. Childs Nerv Syst. 2006;22:1322-1327.
125 Fandiño-Franky J, Torres M, Nariño D, et al. Corpus callosotomy in Colombia and some reflections on care and research among the poor in developing countries. Epilepsia. 2000;4(41 suppl):S22-S27.
126 Nei M, O’Connor M, Liporace J, et al. Refractory generalized seizures: response to corpus callosotomy and vagal nerve stimulation. Epilepsia. 2006;47:115-122.
127 Shimizu H. Our experience with pediatric epilepsy surgery focusing on corpus callosotomy and hemispherotomy. Epilepsia. 2005;1(46 suppl):30-31.
128 Maehara T, Shimizu H. Surgical outcome of corpus callosotomy in patients with drop attacks. Epilepsia. 2001;42:67-71.
129 Jea A, Vachhrajani S, Johnson KK, et al. Corpus callosotomy in children with intractable epilepsy using frameless stereotactic neuronavigation: 12-year experience at The Hospital for Sick Children in Toronto. Neurosurg Focus. 2008;25(3):E7.
130 Nordgren RE, Reeves AG, Viguera AC, et al. Corpus callosotomy for intractable seizures in the pediatric age group. Arch Neurol. 1991;48:364-372.
131 Reutens DC, Bye AM, Hopkins IJ, et al. Corpus callosotomy for intractable epilepsy: seizure outcome and prognostic factors. Epilepsia. 1993;34:904-909.
132 Cendes F, Ragazzo PC, da Costa V, et al. Corpus callosotomy in treatment of medically resistant epilepsy: preliminary results in a pediatric population. Epilepsia. 1993;34:910-917.
133 Mamelak AN, Barbaro NM, Walker JA, et al. Corpus callosotomy: a quantitative study of the extent of resection, seizure control, and neuropsychological outcome. J Neurosurg. 1993;79:688-695.
134 Andersen B, Rogvi-Hansen B, Kruse-Larsen C, et al. Corpus callosotomy: seizure and psychosocial outcome. A 39-month follow-up of 20 patients. Epilepsy Res. 1996;23:77-85.
135 Sorenson JM, Wheless JW, Baumgartner JE, et al. Corpus callosotomy for medically intractable seizures. Pediatr Neurosurg. 1997;27:260-267.
136 McInerney J, Siegel AM, Nordgren RE, et al. Long-term seizure outcome following corpus callosotomy in children. Stereotact Funct Neurosurg. 1999;73:79-83.
137 Jenssen S, Sperling MR, Tracy JI, et al. Corpus callosotomy in refractory idiopathic generalized epilepsy. Seizure. 2006;15:621-629.
138 Rahimi SY, Park YD, Witcher MR, et al. Corpus callosotomy for treatment of pediatric epilepsy in the modern era. Pediatr Neurosurg. 2007;43:202-208.
139 Rathore C, Abraham M, Rao RM, et al. Outcome after corpus callosotomy in children with injurious drop attacks and severe mental retardation. Brain Dev. 2007;29:577-585.
140 Sunaga S, Shimizu H, Sugano H. Long-term follow-up of seizure outcomes after corpus callosotomy. Seizure. 2009;18:124-128.
141 Jea A, Vachhrajani S, Widjaja E, et al. Corpus callosotomy in children and the disconnection syndromes: a review. Childs Nerv Syst. 2008;24:685-692.
142 Lassonde M, Sauerwein C. Neuropsychological outcome of corpus callosotomy in children and adolescents. J Neurosurg Sci. 1997;41:67-73.
143 McLachlan RS. Vagus nerve stimulation for intractable epilepsy: a review. J Clin Neurophysiol. 1997;14:358-368.
144 Boon P, Raedt R, de Herdt V, et al. Electrical stimulation for the treatment of epilepsy. Neurotherapeutics. 2009;6:218-227.
145 Amar AP, Apuzzo ML, Liu CY. Vagus nerve stimulation therapy after failed cranial surgery for intractable epilepsy: results from the vagus nerve stimulation therapy patient outcome registry. Neurosurgery. 2008;62:506-513.
146 Shon YM, Lee KJ, Kim HJ, et al. Effect of chronic deep brain stimulation of the subthalamic nucleus for frontal lobe epilepsy: subtraction SPECT analysis. Stereotact Funct Neurosurg. 2005;83:84-90.
147 Handforth A, DeSalles AA, Krahl SE. Deep brain stimulation of the subthalamic nucleus as adjunct treatment for refractory epilepsy. Epilepsia. 2006;47:1239-1241.
148 Velasco M, Velasco F, Velasco AL, et al. Subacute electrical stimulation of the hippocampus blocks intractable temporal lobe seizures and paroxysmal EEG activities. Epilepsia. 2000;41:158-169.
149 Velasco AL, Velasco F, Velasco M, et al. The role of neuromodulation of the hippocampus in the treatment of intractable complex partial seizures of the temporal lobe. Acta Neurochir Suppl. 2007;97:329-332.
150 Boon P, Vonck K, De Herdt V, et al. Deep brain stimulation in patients with refractory temporal lobe epilepsy. Epilepsia. 2007;48:1551-1560.
151 Osorio I, Overman J, Giftakis J, et al. High frequency thalamic stimulation for inoperable mesial temporal epilepsy. Epilepsia. 2007;48:1561-1571.
152 Andrade DM, Zumsteg D, Hamani C, et al. Long-term follow-up of patients with thalamic deep brain stimulation for epilepsy. Neurology. 2006;66:1571-1573.
153 Velasco AL, Velasco F, Jimenez F, et al. Neuromodulation of the centromedian thalamic nuclei in the treatment of generalized seizures and the improvement of the quality of life in patients with Lennox-Gastaut syndrome. Epilepsia. 2006;47:1203-1212.
154 Wright GD, McLellan DL, Brice JG. A double-blind trial of chronic cerebellar stimulation in twelve patients with severe epilepsy. J Neurol Neurosurg Psychiatry. 1984;47:769-774.
155 Davis R, Emmonds SE. Cerebellar stimulation for seizure control: 17-year study. Stereotact Funct Neurosurg. 1992;58:200-208.
156 Chkhenkeli SA, Sramka M, Lortkipanidze GS, et al. Electrophysiological effects and clinical results of direct brain stimulation for intractable epilepsy. Clin Neurol Neurosurg. 2004;106:318-329.
157 Chkhenkeli SA, Chkhenkeli IS. Effects of therapeutic stimulation of nucleus caudatus on epileptic electrical activity of brain in patients with intractable epilepsy. Stereotact Funct Neurosurg. 1997;69:221-224.
158 Jerger KK, Netoff TI, Francis JT, et al. Early seizure detection. J Clin Neurophysiol. 2001;18:259-268.
159 Gluckman BJ, Nguyen H, Weinstein SL, et al. Adaptive electric field control of epileptic seizures. J Neurosci. 2001;21:590-600.
160 Fountas KN, Smith JR. A novel closed-loop stimulation system in the control of focal, medically refractory epilepsy. Acta Neurochir Suppl. 2007;97:357-362.
161 Fountas KN, Smith JR, Murro AM, et al. Implantation of a closed-loop stimulation in the management of medically refractory focal epilepsy: a technical note. Stereotact Funct Neurosurg. 2005;83:153-158.
162 Morrell MJ, Hirsch LJ, Bergey G, et al. Long-term safety and efficacy of the RNS™ system in adults with medically intractable partial onset seizures. Epilepsia. 2008;49:480-481.
163 Fregni F, Otachi PT, Do Valle A, et al. A randomized clinical trial of repetitive transcranial magnetic stimulation in patients with refractory epilepsy. Ann Neurol. 2006;60:447-455.
164 Theodore WH, Hunter K, Chen R, et al. Transcranial magnetic stimulation for the treatment of seizures: a controlled study. Neurology. 2002;59:560-562.
165 Kinoshita M, Ikeda A, Begum T, et al. Low-frequency repetitive transcranial magnetic stimulation for seizure suppression in patients with extratemporal lobe epilepsy—a pilot study. Seizure. 2005;14:387-392.
166 Santiago-Rodríguez E, Cárdenas-Morales L, Harmony T, et al. Repetitive transcranial magnetic stimulation decreases the number of seizures in patients with focal neocortical epilepsy. Seizure. 2008;17:677-683.
167 Joo EY, Han SJ, Chung SH, et al. Antiepileptic effects of low-frequency repetitive transcranial magnetic stimulation by different stimulation durations and locations. Clin Neurophysiol. 2007;118:702-708.
168 Fregni F, Thome-Souza S, Bermpohl F, et al. Antiepileptic effects of repetitive transcranial magnetic stimulation in patients with cortical malformations: an EEG and clinical study. Stereotact Funct Neurosurg. 2005;83:57-62.
169 Misawa S, Kuwabara S, Shibuya K, et al. Low-frequency transcranial magnetic stimulation for epilepsia partialis continua due to cortical dysplasia. J Neurol Sci. 2005;234:37-39.
170 Brasil-Neto JP, de Araujo DP, Teixeira WA, et al. Experimental therapy of epilepsy with transcranial magnetic stimulation: lack of additional benefit with prolonged treatment. Arq Neuropsiquiatr. 2004;62:21-25.
171 Rotenberg A, Bae EH, Takeoka M, et al. Repetitive transcranial magnetic stimulation in the treatment of epilepsia partialis continua. Epilepsy Behav. 2009;14:253-257.
172 Rotenberg A, Depositario-Cabacar D, Bae EH, et al. Transient suppression of seizures by repetitive transcranial magnetic stimulation in a case of Rasmussen’s encephalitis. Epilepsy Behav. 2008;13:260-262.
173 Conte A, Gilio F, Iacovelli E, et al. Effects of repetitive transcranial magnetic stimulation on spike-and-wave discharges. Neurosci Res. 2007;57:140-142.
174 Sakkas P, Theleritis CG, Psarros C, et al. Jacksonian seizure in a manic patient treated with rTMS. World J Biol Psychiatry. 2008;9:159-160.
175 Mihajlovic-Madzarevic V, Pappas P. Treatment of refractory seizures due to a benign mass present in the corpus callosum with an ion magnetic inductor: case report. Brain Tumor Pathol. 2005;22:93-95.
176 Rosa MA, Picarelli H, Teixeira MJ, et al. Accidental seizure with repetitive transcranial magnetic stimulation. J ECT. 2006;22:265-266.
177 Reis J, Rosenow F, Fritsch B, et al. Possible transcallosal seizure induction by paired pulse transcranial magnetic stimulation in a patient with frontal lobe epilepsy. J Neurol Neurosurg Psychiatry. 2005;76:454-455.
178 Schrader LM, Stern JM, Koski L, et al. Seizure incidence during single- and paired-pulse transcranial magnetic stimulation (TMS) in individuals with epilepsy. Clin Neurophysiol. 2004;115:2728-2737.
179 Bae EH, Schrader LM, Machii K, et al. Safety and tolerability of repetitive transcranial magnetic stimulation in patients with epilepsy: a review of the literature. Epilepsy Behav. 2007;10:521-528.
180 Fish DR, Smith SJ, Quesney LF, et al. Surgical treatment of children with medically intractable frontal or temporal lobe epilepsy: results and highlights of 40 years’ experience. Epilepsia. 1993;34:244-247.
181 Rasmussen T. Surgery for epilepsy arising in regions other than the temporal and frontal lobes. Adv Neurol. 1975;8:207-226.
182 Goldring S. Pediatric epilepsy surgery. Epilepsia. 1987;1(28 suppl):S82-S102.
183 Morris HH, Estes ML, Gilmore R, et al. Chronic intractable epilepsy as the only symptom of primary brain tumor. Epilepsia. 1993;34:1038-1043.
184 Fried I, Kim JH, Spencer DD. Limbic and neocortical gliomas associated with intractable seizures: a distinct clinicopathological group. Neurosurgery. 1994;34:815-823.
185 Fried I. Management of low-grade gliomas: results of resections without electrocorticography. Clin Neurosurg. 1995;42:453-463.
186 Berger MS, Ghatan S, Haglund MM, et al. Low-grade gliomas associated with intractable epilepsy: seizure outcome utilizing electrocorticography during tumor resection. J Neurosurg. 1993;79:62-69.
187 Moran NF, Fish DR, Kitchen N, et al. Supratentorial cavernous haemangiomas and epilepsy: a review of the literature and case series. J Neurol Neurosurg Psychiatry. 1999;66:561-568.
188 Hoh BL, Chapman PH, Loeffler JS, et al. Results of multimodality treatment for 141 patients with brain arteriovenous malformations and seizures: factors associated with seizure incidence and seizure outcomes. Neurosurgery. 2002;51:303-309.
189 Cepeda C, Andre VM, Levine MS, et al. Epileptogenesis in pediatric cortical dysplasia: the dysmature cerebral developmental hypothesis. Epilepsy Behav. 2006;9:219-235.
190 Kral T, Clusmann H, Blumcke I, et al. Outcome of epilepsy surgery in focal cortical dysplasia. J Neurol Neurosurg Psychiatry. 2003;74:183-188.
191 Sisodiya SM. Surgery for malformations of cortical development causing epilepsy. Brain. 2000;123:1075-1091.
192 Lerner JT, Salamon N, Hauptman JS, et al. Assessment and surgical outcomes for mild type I and severe type II cortical dysplasia: a critical review and the UCLA experience. Epilepsia. 2009;50:1310-1335.
193 Karenfort M, Kruse B, Freitag H, et al. Epilepsy surgery outcome in children with focal epilepsy due to tuberous sclerosis complex. Neuropediatrics. 2002;33:255-261.
194 Benbadis SR. The EEG in nonepileptic seizures. J Clin Neurophysiol. 2006;23:340-352.
195 Ding L, Worrell GA, Lagerlund TD, et al. 3D source localization of interictal spikes in epilepsy patients with MRI lesions. Phys Med Biol. 2006;51:4047-4062.
196 McGonigal A, Bartolomei F, Regis J, et al. Stereoelectroencephalography in presurgical assessment of MRI-negative epilepsy. Brain. 2007;130:3169-3183.
197 Binnie CD, Stefan H. Modern electroencephalography: its role in epilepsy management. Clin Neurophysiol. 1999;110:1671-1697.
198 Cascino GD. Video-EEG monitoring in adults. Epilepsia. 2002;3(suppl 43):80-93.
199 Krendl R, Lurger S, Baumgartner C. Absolute spike frequency predicts surgical outcome in TLE with unilateral hippocampal atrophy. Neurology. 2008;71:413-418.
200 Blume WT, Kaibara M. Localization of epileptic foci in children. Can J Neurol Sci. 1991;18:570-572.
201 Lee SK, Kim JY, Hong KS, et al. The clinical usefulness of ictal surface EEG in neocortical epilepsy. Epilepsia. 2000;41:1450-1455.
202 Spencer SS, Spencer DD, Williamson PD, et al. The localizing value of depth electroencephalography in 32 patients with refractory epilepsy. Ann Neurol. 1982;12:248-253.
203 Shenai MB, Ross DA, Sagher O. The use of multiplanar trajectory planning in the stereotactic placement of depth electrodes. Neurosurgery. 2007;60:272-276.
204 Brekelmans GJ, van Emde Boas W, Velis DN, et al. Comparison of combined versus subdural or intracerebral electrodes alone in presurgical focus localization. Epilepsia. 1998;39:1290-1301.
205 Palmini A, Andermann F, Dubeau F, et al. Occipitotemporal epilepsies: evaluation of selected patients requiring depth electrodes studies and rationale for surgical approaches. Epilepsia. 1993;34:84-96.
206 Smith JR, Flanigin HF, King DW, et al. An 8-year experience with depth electrodes in the evaluation of ablative seizure surgery candidates. Stereotact Funct Neurosurg. 1990;54-55:60-66.
207 Espinosa J, Olivier A, Andermann F, et al. Morbidity of chronic recording with intracranial depth electrodes in 170 patients. Stereotact Funct Neurosurg. 1994;63:63-65.
208 Fried I, Wilson CL, Maidment NT, et al. Cerebral microdialysis combined with single-neuron and electroencephalographic recording in neurosurgical patients. Technical note. J Neurosurg. 1999;91:697-705.
209 Cornford EM, Shamsa K, Zeitzer JM, et al. Regional analyses of CNS microdialysate glucose and lactate in seizure patients. Epilepsia. 2002;43:1360-1371.
210 Ikeda A, Miyamoto S, Shibasaki H. Cortical motor mapping in epilepsy patients: information from subdural electrodes in presurgical evaluation. Epilepsia. 2002;9(suppl 43):56-60.
211 Spencer SS, Spencer DD, Williamson PD, et al. Combined depth and subdural electrode investigation in uncontrolled epilepsy. Neurology. 1990;40:74-79.
212 Behrens E, Zentner J, van Roost D, et al. Subdural and depth electrodes in the presurgical evaluation of epilepsy. Acta Neurochir (Wien). 1994;128:84-87.
213 Mehta AD, Labar D, Dean A, et al. Frameless stereotactic placement of depth electrodes in epilepsy surgery. J Neurosurg. 2005;102:1040-1045.
214 Wyler AR, Walker G, Somes G. The morbidity of long-term seizure monitoring using subdural strip electrodes. J Neurosurg. 1991;74:734-737.
215 Hamer HM, Morris HH, Mascha EJ, et al. Complications of invasive video-EEG monitoring with subdural grid electrodes. Neurology. 2002;58:97-103.
216 Fried I. Magnetic resonance imaging and epilepsy: neurosurgical decision making. Magn Reson Imaging. 1995;13:1163-1170.
217 Phal PM, Usmanov A, Nesbit GM, et al. Qualitative comparison of 3-T and 1.5-T MRI in the evaluation of epilepsy. AJR Am J Roentgenol. 2008;191:890-895.
218 Eriksson SH, Free SL, Thom M, et al. Correlation of quantitative MRI and neuropathology in epilepsy surgical resection specimens—T2 correlates with neuronal tissue in gray matter. Neuroimage. 2007;37:48-55.
219 Betting LE, Mory SB, Lopes-Cendes I, et al. MRI reveals structural abnormalities in patients with idiopathic generalized epilepsy. Neurology. 2006;67:848-852.
220 Wieshmann UC, Free SL, Everitt AD, et al. Magnetic resonance imaging in epilepsy with a fast FLAIR sequence. J Neurol Neurosurg Psychiatry. 1996;61:357-361.
221 Riederer SJ, Jack CR, Grimm RC, et al. New technical developments in magnetic resonance imaging of epilepsy. Magn Reson Imaging. 1995;13:1095-1098.
222 Cascino GD, Jack CRJr, Parisi JE, et al. MRI in the presurgical evaluation of patients with frontal lobe epilepsy and children with temporal lobe epilepsy: pathologic correlation and prognostic importance. Epilepsy Res. 1992;11:51-59.
223 Radhakrishnan K, Fried I, Cascino GD. Lesionectomy: management of substrate-directed epilepsies. In: Engel JJr, editor. Epilepsy. Philadelphia: Lippincott Williams & Wilkins, 2008.
224 Ken S, Di Gennaro G, Giulietti G, et al. Quantitative evaluation for brain CT/MRI coregistration based on maximization of mutual information in patients with focal epilepsy investigated with subdural electrodes. Magn Reson Imaging. 2007;25:883-888.
225 Drzezga A, Arnold S, Minoshima S, et al. 18F-FDG PET studies in patients with extratemporal and temporal epilepsy: evaluation of an observer-independent analysis. J Nucl Med. 1999;40:737-746.
226 Theodore WH, Gaillard WD. Positron emission tomography in neocortical epilepsies. Adv Neurol. 2000;84:435-446.
227 Van Paesschen W, Dupont P, Sunaert S, et al. The use of SPECT and PET in routine clinical practice in epilepsy. Curr Opin Neurol. 2007;20:194-202.
228 Asenbaum S, Baumgartner C. Nuclear medicine in the preoperative evaluation of epilepsy. Nucl Med Commun. 2001;22:835-840.
229 Harvey AS, Hopkins IJ, Bowe JM, et al. Frontal lobe epilepsy: clinical seizure characteristics and localization with ictal 99mTc-HMPAO SPECT. Neurology. 1993;43:1966-1980.
230 Ho SS, Berkovic SF, Newton MR, et al. Parietal lobe epilepsy: clinical features and seizure localization by ictal SPECT. Neurology. 1994;44:2277-2284.
231 O’Brien TJ, So EL, Mullan BP, et al. Subtraction ictal SPECT co-registered to MRI improves clinical usefulness of SPECT in localizing the surgical seizure focus. Neurology. 1998;50:445-454.
232 O’Brien TJ, So EL, Mullan BP, et al. Subtraction SPECT co-registered to MRI improves postictal SPECT localization of seizure foci. Neurology. 1999;52:137-146.
233 O’Brien TJ, So EL, Mullan BP, et al. Subtraction peri-ictal SPECT is predictive of extratemporal epilepsy surgery outcome. Neurology. 2000;55:1668-1677.
234 Sporis D, Hajnsek S, Boban M, et al. Epilepsy due to malformations of cortical development—correlation of clinical, MRI and Tc-99mECD SPECT findings. Coll Antropol. 2008;32:345-350.
235 Lee DS, Lee SK, Kim YK, et al. Superiority of HMPAO ictal SPECT to ECD ictal SPECT in localizing the epileptogenic zone. Epilepsia. 2002;43:263-269.
236 Siegel A, Lewis P, Siegel AM. The value of interictal brain SPECT in epilepsy patients without mesial-temporal sclerosis. Clin Nucl Med. 2002;27:716-720.
237 Wheless JW, Willmore LJ, Breier JI, et al. A comparison of magnetoencephalography, MRI, and V-EEG in patients evaluated for epilepsy surgery. Epilepsia. 1999;40:931-941.
238 Eliashiv DS, Elsas SM, Squires K, et al. Ictal magnetic source imaging as a localizing tool in partial epilepsy. Neurology. 2002;59:1600-1610.
239 Janjua FN, Eliashiv DS, Fried I. The added value of magnetic source imaging in a large series of medically refractory partial epilepsy patients that are candidates for epilepsy surgery. Epilepsia. 2003;44(suppl 9):251.
240 Mamelak AN, Lopez N, Akhtari M, et al. Magnetoencephalography-directed surgery in patients with neocortical epilepsy. J Neurosurg. 2002;97:865-873.
241 Minassian BA, Otsubo H, Weiss S, et al. Magnetoencephalographic localization in pediatric epilepsy surgery: comparison with invasive intracranial electroencephalography. Ann Neurol. 1999;46:627-633.
242 RamachandranNair R, Otsubo H, Shroff MM, et al. MEG predicts outcome following surgery for intractable epilepsy in children with normal or nonfocal MRI findings. Epilepsia. 2007;48:149-157.
243 Lundbom N, Gaily E, Vuori K, et al. Proton spectroscopic imaging shows abnormalities in glial and neuronal cell pools in frontal lobe epilepsy. Epilepsia. 2001;42:1507-1514.
244 Laxer KD. Magnetic resonance spectroscopy in neocortical epilepsies. Adv Neurol. 2000;84:405-414.
245 Hajek M, Dezortova M, Krsek P. (1)H MR spectroscopy in epilepsy. Eur J Radiol. 2008;67:258-267.
246 Garcia PA, Laxer KD, van der Grond J, et al. Phosphorus magnetic resonance spectroscopic imaging in patients with frontal lobe epilepsy. Ann Neurol. 1994;35:217-221.
247 Garcia PA, Laxer KD, van der Grond J, et al. Proton magnetic resonance spectroscopic imaging in patients with frontal lobe epilepsy. Ann Neurol. 1995;37:279-281.
248 Stanley JA, Cendes F, Dubeau F, et al. Proton magnetic resonance spectroscopic imaging in patients with extratemporal epilepsy. Epilepsia. 1998;39:267-273.
249 Krsek P, Hajek M, Dezortova M, et al. (1)H MR spectroscopic imaging in patients with MRI-negative extratemporal epilepsy: correlation with ictal onset zone and histopathology. Eur Radiol. 2007;17:2126-2135.
250 Chandra PS, Salamon N, Huang J, et al. FDG-PET/MRI coregistration and diffusion-tensor imaging distinguish epileptogenic tubers and cortex in patients with tuberous sclerosis complex: a preliminary report. Epilepsia. 2006;47:1543-1549.
251 Swanson SJ, Sabsevitz DS, Hammeke TA, et al. Functional magnetic resonance imaging of language in epilepsy. Neuropsychol Rev. 2007;17:491-504.
252 Fried I. Functional neuroimaging in presurgical localization of essential cortical processing zones. Adv Neurol. 2000;83:297-303.
253 Andelman F, Neufeld M, Reider-Groswasser I, et al. [Presurgical neuropsychological assessment in epilepsy: the Wada test.]. Harefuah. 2000;138:440-444. 519
254 Szaflarski JP, Holland SK, Jacola LM, et al. Comprehensive presurgical functional MRI language evaluation in adult patients with epilepsy. Epilepsy Behav. 2008;12:74-83.
255 Lemieux L, Krakow K, Fish DR. Comparison of spike-triggered functional MRI BOLD activation and EEG dipole model localization. Neuroimage. 2001;14:1097-1104.
256 Krakow K, Woermann FG, Symms MR, et al. EEG-triggered functional MRI of interictal epileptiform activity in patients with partial seizures. Brain. 1999;122:1679-1688.
257 Cunningham CJ, Zaamout Mel F, Goodyear B, et al. Simultaneous EEG-fMRI in human epilepsy. Can J Neurol Sci. 2008;35:420-435.
258 Lengler U, Kafadar I, Neubauer BA, et al. fMRI correlates of interictal epileptic activity in patients with idiopathic benign focal epilepsy of childhood. A simultaneous EEG-functional MRI study. Epilepsy Res. 2007;75:29-38.
259 Al-Asmi A, Benar CG, Gross DW, et al. fMRI activation in continuous and spike-triggered EEG-fMRI studies of epileptic spikes. Epilepsia. 2003;44:1328-1339.
260 Federico P, Archer JS, Abbott DF, et al. Cortical/subcortical BOLD changes associated with epileptic discharges: an EEG-fMRI study at 3 T. Neurology. 2005;64:1125-1130.
261 Gotman J. Epileptic networks studied with EEG-fMRI. Epilepsia. 2008;3(suppl 49):42-51.
262 Keogh BP, Cordes D. Quantitative approaches to functional MRI: applications in epilepsy. Epilepsia. 2007;4(suppl 48):27-36.
263 Jones-Gotman M. Clinical neuropsychology and neocortical epilepsies. Adv Neurol. 2000;84:457-462.
264 Nelson E, Fischer M. Neuropsychological evaluation of the child with epilepsy. Dis Mon. 2007;53:162-168.
265 Lassonde M, Sauerwein HC, Gallagher A, et al. Neuropsychology: traditional and new methods of investigation. Epilepsia. 2006;2(suppl 47):9-13.
266 Helmstaedter C. Neuropsychological aspects of epilepsy surgery. Epilepsy Behav. 2004;1(suppl 5):S45-S55.
267 Blackburn LB, Lee GP, Westerveld M, et al. The Verbal IQ/Performance IQ discrepancy as a sign of seizure focus laterality in pediatric patients with epilepsy. Epilepsy Behav. 2007;10:84-88.
268 Wada J, Rasmussen T. Intracarotid injection of sodium Amytal for the lateralization of cerebral speech dominance: experimental and clinical observations. J Neurosurg. 1960;17:266-282.
269 Rausch R, Silfvenius H, Wieser H-G. Intraarterial amobarbital procedures. In: Engel JJr, editor. Surgical Treatment of the Epilepsies. New York: Raven Press; 1993:341-357.
270 Haag A, Knake S, Hamer HM, et al. The Wada test in Austrian, Dutch, German, and Swiss epilepsy centers from 2000 to 2005: a review of 1421 procedures. Epilepsy Behav. 2008;13:83-89.
271 Ramsey NF, Sommer IE, Rutten GJ, et al. Combined analysis of language tasks in fMRI improves assessment of hemispheric dominance for language functions in individual subjects. Neuroimage. 2001;13:719-733.
272 Richardson MP. Epilepsy and surgical mapping. Br Med Bull. 2003;65:179-192.
273 Kim CH, Chung CK, Lee SK, et al. Parietal lobe epilepsy: surgical treatment and outcome. Stereotact Funct Neurosurg. 2004;82:175-185.
274 Shinoura N, Yamada R, Kodama T, et al. Intraoperative cortical mapping has low sensitivity for the detection of motor function in proximity to a tumor in the primary motor area. Stereotact Funct Neurosurg. 2005;83:135-141.
275 Erickson KM, Cole DJ. Anesthetic considerations for awake craniotomy for epilepsy. Anesthesiol Clin. 2007;25:535-555. ix
276 Taylor MD, Bernstein M. Awake craniotomy with brain mapping as the routine surgical approach to treating patients with supratentorial intraaxial tumors: a prospective trial of 200 cases. J Neurosurg. 1999;90:35-41.
277 Huncke K, Van de Wiele B, Fried I, et al. The asleep-awake-asleep anesthetic technique for intraoperative language mapping. Neurosurgery. 1998;42:1312-1316.
278 Goldmann RE, Golby AJ. Atypical language representation in epilepsy: implications for injury-induced reorganization of brain function. Epilepsy Behav. 2005;6:473-487.
279 Asano E, Benedek K, Shah A, et al. Is intraoperative electrocorticography reliable in children with intractable neocortical epilepsy? Epilepsia. 2004;45:1091-1099.
280 Herrick IA, Craen RA, Gelb AW, et al. Propofol sedation during awake craniotomy for seizures: electrocorticographic and epileptogenic effects. Anesth Analg. 1997;84:1280-1284.
281 Souter MJ, Rozet I, Ojemann JG, et al. Dexmedetomidine sedation during awake craniotomy for seizure resection: effects on electrocorticography. J Neurosurg Anesthesiol. 2007;19:38-44.
282 Oda Y, Toriyama S, Tanaka K, et al. The effect of dexmedetomidine on electrocorticography in patients with temporal lobe epilepsy under sevoflurane anesthesia. Anesth Analg. 2007;105:1272-1277. table of contents
283 Tran TA, Spencer SS, Javidan M, et al. Significance of spikes recorded on intraoperative electrocorticography in patients with brain tumor and epilepsy. Epilepsia. 1997;38:1132-1139.
284 Kuruvilla A, Flink R. Intraoperative electrocorticography in epilepsy surgery: useful or not? Seizure. 2003;12:577-584.
285 Pondal-Sordo M, Diosy D, Tellez-Zenteno JF, et al. Epilepsy surgery involving the sensory-motor cortex. Brain. 2006;129:3307-3314.
286 Eisner W, Burtscher J, Bale R, et al. Use of neuronavigation and electrophysiology in surgery of subcortically located lesions in the sensorimotor strip. J Neurol Neurosurg Psychiatry. 2002;72:378-381.
287 Maciunas RJ. Computer-assisted neurosurgery. Clin Neurosurg. 2006;53:267-271.
288 Schulze-Bonhage AH, Huppertz HJ, Comeau RM, et al. Visualization of subdural strip and grid electrodes using curvilinear reformatting of 3D MR imaging data sets. AJNR Am J Neuroradiol. 2002;23:400-403.
289 Kaibara T, Myles ST, Lee MA, et al. Optimizing epilepsy surgery with intraoperative MR imaging. Epilepsia. 2002;43:425-429.
290 Schwartz TH, Marks D, Pak J, et al. Standardization of amygdalohippocampectomy with intraoperative magnetic resonance imaging: preliminary experience. Epilepsia. 2002;43:430-436.
291 Levivier M, Wikler D, Massager N, et al. [Intraoperative MRI and epilepsy surgery.]. Neurochirurgie. 2008;54:448-452.
292 Prakash N, Uhlemann F, Sheth SA, et al. Current trends in intraoperative optical imaging for functional brain mapping and delineation of lesions of language cortex. Neuroimage. 2008;47(suppl 2):T116-T126.
293 Pouratian N, Cannestra AF, Martin NA, et al. Intraoperative optical intrinsic signal imaging: a clinical tool for functional brain mapping. Neurosurg Focus. 2002;13(4):e1.
294 Cannestra AF, Bookheimer SY, Pouratian N, et al. Temporal and topographical characterization of language cortices using intraoperative optical intrinsic signals. Neuroimage. 2000;12:41-54.
295 Cannestra AF, Pouratian N, Bookheimer SY, et al. Temporal spatial differences observed by functional MRI and human intraoperative optical imaging. Cereb Cortex. 2001;11:773-782.
296 Schwartz TH. The application of optical recording of intrinsic signals to simultaneously acquire functional, pathological and localizing information and its potential role in neurosurgery. Stereotact Funct Neurosurg. 2005;83:36-44.
* See references 29, 36, 40–44, 47, 50–54, 69, 77, 81, 86, 88, 89, 180, 181.
† See references 39, 42–44, 49–51, 53, 54, 74, 77, 78, 86, 88, 89, 94.
* See references 42–45, 50, 51, 53, 54, 74, 75, 77, 81, 86–89.
* See references 38, 41–45, 48–51, 53, 54, 74, 75, 77, 78, 86–89, 94.
† See references 38, 42–45, 48, 50, 51, 53, 54, 74, 75, 77, 86–89, 94.