Chapter 20 Sunscreens
CHEMICAL SUNSCREENS
The FDA-approved chemical sunscreens and the maximum allowed concentration for each are listed in Table 20.1. These ‘sunscreen active ingredients’ are defined as absorbing, reflecting, or scattering radiation in the ultraviolet range at wavelengths of 290–400 nm. The chemical (also called organic or soluble) sunscreen active ingredients prevent sunburn by absorbing UV radiation as photons of light energy that are transformed into harmless long wave radiation and then re-emitted as heat. The FDA defined maximum, rather than minimum, concentrations of each to avoid subjecting consumers to unnecessarily high levels of any active ingredient in sunscreen combination products. This provision also recognizes that final product testing, not the concentration of each active ingredient, determines efficacy.
Active ingredient | Maximum concentration (%) |
Aminobenzoic acid (PABA) | 15 |
Avobenzone | 3 |
Cinoxate | 3 |
Dioxybenzone | 3 |
Ecamsule | 10 |
Homosalate | 15 |
Methyl anthranilate | 5 |
Octocrylene | 10 |
Octyl methoxycinnamate | 10 |
Octyl salicylate | 5 |
Oxybenzone | 6 |
Padimate O | 8 |
Phenylbenzimidazole sulfonic acid | 4 |
Sulisobenzone | 10 |
Trolamine salicylate | 12 |
PHYSICAL SUNSCREENS
Traditionally, physical agents used to prevent sunburn were called ‘sunblocks’ while chemical agents were ‘sunscreens’. The terminology is misleading because it suggests that the former merely scatter or reflect UV radiation. In fact, the physical (also called inorganic or insoluble) agents, titanium dioxide and zinc oxide, also act as semiconductors that absorb UV radiation and release it as heat. The use of the term ‘chemical-free’ for sunscreens containing only physical, not chemical, sunscreen agents is also confusing for consumers, since all active and inactive ingredients have been obtained and/or combined through some chemical process. The FDA-approved maximum concentration of these agents in sunscreen is listed in Table 20.2.
Active ingredient | Maximum concentration (%) |
Titanium dioxide | 25 |
Zinc oxide | 25 |
RATING EFFICACY
Sunscreen ingredients differ in their absorption spectrum, as shown in Table 20.3. Ideally a sunscreen should provide protection against the full spectrum of ultraviolet radiation. Until now most of the focus of the FDA’s attention has been on reducing exposure to UVB light. The sun protection factor (SPF), which measures UVB protection, was the only internationally standardized measure of a sunscreen’s ability to filter UV radiation. It is the ratio of the UV energy required to produce a minimal erythema dose (MED) on sunscreen-protected skin to the UV energy required to produce a MED on unprotected skin (Box 20.1). The MED is the quantity of energy required to produce the first perceptible redness reaction of the skin with clearly defined borders. Energy is delivered utilizing a filtered light source simulating the solar emission spectrum, with 94% of its output between 290 and 400 nm. (This mimics sunlight at sea level at a zenith angle of 10°.) For any given product, measurement must be done on between 20 and 25 test subjects of Fitzpatrick skin types I, II, and III. Test material is applied to an area of at least 50 cm2 at a thickness of 2 mg/cm2.
Table 20.3 Absorbance range of selected sunscreen active ingredients
Sunscreen | Absorbance range (nm) |
Homosalate | 300–310 |
Octyl salicylate | 300–310 |
Aminobenzoic acid (PABA) | 260–313 |
Padimate O | 290–315 |
Methyl anthralinate | 290–320 |
Phenylbenzimidazole sulfonic acid | 290–320 |
Trolamine salicylate | 260–320 |
Cinoxate | 270–328 |
Octyl methoxycinnamate | 270–328 |
Oxybenzone | 270–350 |
Sulisobenzone | 270–360 |
Dioxybenzone | 260–380 |
Zinc oxide | 250–380 |
Avobenzone | 310–400 |
Ecamsule | 290–400 |
Titanium dioxide | 250–400 |
The SPF of a given OTC topical sunscreen is determined by testing of that product as above. In accordance with FDA regulations, multiple sun protective active ingredients can be combined as long as each contributes a minimum SPF of at least 2 to the finished product. This requirement is meant to avoid the addition of unnecessary ingredients. FDA will revise some of the existing SPF testing procedures to decrease the health risk to persons enrolled in the SPF test and further enhance accuracy of SPF values.
Regulations concerning UVA were delayed until recently since reliable testing methodologies were not available. However, the new proposed regulations address manufacturing, testing, and labeling of UVA sunscreens. Sunscreen manufacturers will now have to post the degree of protection afforded by a particular product against UVA rays. The scale of one to four stars corresponding to low, medium, high or very high UVA protection is to be prominently displayed on OTC sunscreen products near the SPF rating (Figs 20.1 and 20.2). Because consumers are familiar with SPF numbers, the FDA believes there may be confusion if UVB and UVA protection levels were both identified by numbers. Since star rating has been used in a variety of industries (e.g. hotels and restaurants), the FDA expects consumers will learn how to use this information to select the appropriate sunscreen, as they have done with SPF values. Furthermore, the product will bear a ‘no UVA protection’ marking on the front label if the sunscreen does not provide even a low level of protection.
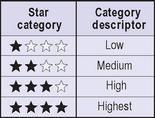
Fig. 20.1 The principal display panel.
Adapted from http://www.fda.gov/cder/drug/infopage/sunscreen/qa.htm
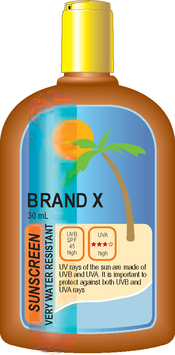
Fig. 20.2 Proposed labeling configuration for UVA protection.
Adapted from http://www.fda.gov/cder/drug/infopage/sunscreen/qa.htm