Chapter 138 Spinal Implant Attributes
Cantilever Beam Fixation
Cantilever
A cantilever beam is simply defined as a beam that is rigidly supported only at one end and carries a load. Examples of this commonly used engineering construct are numerous (Fig. 138-1). Spinal instrumentation constructs using cantilever beams are also common.1,2 It is important to recognize, however, that spinal instrumentation constructs are rarely composed of pure cantilever beams and may function otherwise in different clinical scenarios and when challenged with other stresses or moments.
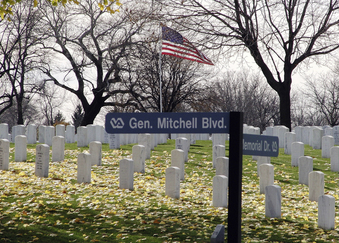
FIGURE 138-1 Cantilever street signs on the grounds of the Wood National Cemetery, Milwaukee, Wisconsin.
An idealized cantilever beam is shown in Figure 138-2. A load applied to the beam is resisted by shear stress and a moment at the point of attachment to the support. In this idealized situation, with axial loading, the beam experiences a shear stress parallel to the z-axis (vertical) and a moment. This moment consists of a force and an instantaneous axis of rotation about which the force is applied.3
Cantilevers in Spinal Instrumentation
Cantilevers are frequently used in modern constrained spinal instrumentation. Figure 138-3 depicts a simple idealized short-segment nonsegmental spinal instrumentation construct. Such a construct has been termed cantilever beam fixation1,2 Brief analysis reveals the presence of four potential cantilevers. Each screw is a cantilever beam supported by the vertebral body support. Each rod is a cantilever beam supported by a screw support. Analysis of such a complex system requires either significant simplification or separate analysis of each component before considering the properties of the entire system.
Bone-Screw Cantilever
Resistance to Shear Stress
The shear stress resistance of a typical screw is typically much greater than the resistance of the bone in which is embedded. Resistance to shear stress at the bone-screw interface is primarily determined by the mechanical properties of the bone composing the vertebra. An idealized diagram of a vertebral body is shown in Figure 138-4. The screw traverses a finite thickness of cortical bone, entering the cancellous bone of the central vertebral body. Shear stress resistance is a function of the relative contributions of the bone-screw interfaces in these locations. This contribution is related to the yield strength of the material, the stress at which a material begins to deform plastically, and the area of contact. Although the cancellous bone has significantly lower yield strength, this property is mitigated by the larger area of the bone-screw interface. The total shear stress resistance is the sum of the values for the engaged areas of cortical and cancellous bone.
Resistance to Bending Moment
Resistance to y-plane bending moment is more complex. An idealized diagram of a screw and vertebral body is depicted in Figure 138-5. The effect of differing material properties between the screw, cortical bone, and cancellous bone produces a complex interaction.
A refined definition of a cantilever is a load-bearing member, such as a beam, that projects beyond a fulcrum and is supported by a balancing member or a downward force behind the fulcrum. This definition applies more accurately to resistance to bending moment at the bone-screw interface below the threshold for cortical bone failure—its yield strength. Because of the difference in yield strength of the two types of bone, the cortical bone at the entrance to the vertebral body, particularly in the region of the pedicle, can be regarded as a fulcrum. The screw functions as the load-bearing beam. A load is applied to the head of the screw across the instantaneous axis of rotation at the cortical fulcrum, creating a moment. The magnitude of this moment varies in accordance with the formula: moment = force × distance (from the fulcrum). The resisting moment is generated by downward stress applied by the body of the screw behind the fulcrum. With only the short screw head protruding beyond the cortex, the applied moment may be quite low because the length of the level arm is minimal unless extended by connection to other implants.
Failure at the bone-screw interface occurs in response to a bending moment if the yield strength of either the fulcrum (cortical bone) or the downward force (cancellous bone) behind the fulcrum is exceeded.4 The yield strength for both regions is increased with increased contact area. This is a function of both screw diameter (cortical and cancellous) and screw length (relevant only for the cancellous portion). An increase in screw length increases contact area only in the cancellous region, whereas contact area is fixed for a given screw diameter and a given thickness of cortical bone at the entrance. In addition, longer screws increase resistance to a bending moment by increasing the distance between the cortical fulcrum and the distal tip of the screw, increasing the moment of the resistive force in the cancellous region.
In response to a bending moment, the mechanical properties of the screw shaft may also be relevant. The bending resistance of the screw is related to the area moment of inertia, determined primarily by the minor diameter of the screw. For a screw of circular cross section, elastic resistance to a bending moment is proportional to the fourth power of the minor diameter.5 The bending moment is least at the proximal and distal end of the screw and is inversely proportional to the distance from the fulcrum. The bending moment reaches its maximum at the point of the cortical fulcrum. The advantage of a larger-diameter screw lies not only in the increased contact area between the screw and the bone behind the fulcrum but also in the larger area moment of inertia. The use of a longer screw increases both the area of bone-screw contact and the length of the lever arm in cancellous bone behind the fulcrum, although this effect occurs at the expense of increasing bending moment at the screw entry point.