26 Spinal Cord Tumors
Primary Tumors Involving the Spinal Cord
Epidemiology and Genetics
Primary tumors involving the spinal cord are uncommon1 and tend to occur in young patients. In adults, they are outnumbered by primary brain tumors at a ratio of roughly 20 : 1; for children, a ratio of 5 : 1 is reported by most centers. Tumors arising from the spinal canal and vertebrae show a more heterogeneous age distribution. The rarity of primary spinal tumors makes it difficult to establish a clear-cut association with specific cytogenetic abnormalities. Some investigators have found an association between spinal and brain ependymomas and the loss of sequences in chromosome arm 22q, suggesting the presence of one or more ependymoma tumor suppressor genes in this location.2–4 Pediatric ependymomas and astrocytomas have been associated with loss of chromosome arm 17p.3,4 Some myxopapillary and papillary ependymomas have been associated with bcl-2 oncoprotein expression.5 Other ependymomas have been associated with mutations in the MEN1 gene and loss of heterozygosity in the chromosome arm 11q region.6
Prior exposure to therapeutic irradiation probably increases the risk for developing spinal canal meningiomas, soft tissue sarcomas, vertebral body sarcomas, and possibly spinal cord gliomas, although the magnitude of this risk is unclear. Neurofibromatosis is strongly associated with an increased incidence of spinal canal neurofibrosarcoma and has been associated with both intracranial and spinal cord ependymomas as well.2 This latter association may be indirect, with the actual cause being the loss of a separate ependymoma tumor suppressor gene located near the NF2 gene.3
Anatomy
Approximately 10% of all primary tumors involving the cord arise from the vertebrae, 65% from the spinal canal, and 25% from the cord proper. For purposes of treatment, it is useful to group primary tumors based on whether they arise from the cord, spinal canal, or vertebrae (Table 26-1). This system helps to predict whether radical surgery is feasible. It also suggests whether the normal spinal cord must be included within the high-dose irradiation volume or could instead be partially excluded from treatment with the use of sophisticated irradiation techniques.
Table 26-1 Common Primary Tumor Types Involving the Spinal Cord
Location | Histologic Types |
---|---|
Vertebral body (excluding myeloma/plasmacytoma) | Osteogenic sarcoma |
Chondrosarcoma | |
Chordoma | |
Spinal canal | Neurofibrosarcoma |
Malignant schwannoma | |
Meningioma | |
Spinal cord/cauda equina | Astrocytoma |
Ependymoma | |
Oligodendroglioma |
Pathology
Grade is an important consideration for several tumor types. Low-grade spinal canal sarcomas appear to be at low risk for both local and distant recurrence if completely resected. High-grade, malignant meningiomas are probably at higher risk for recurrence than the more typical benign meningiomas. The importance of grade is uncertain for ependymomas, as some series have identified grade as a significant prognostic factor,7–9 and others have not.10 The overall risk of subsequent intracranial failure for low-grade spinal ependymomas is low, on the order of 7%.11 However, this value is probably higher for histologically malignant ependymomas.12,13 Myxopapillary ependymomas reportedly have a particularly favorable prognosis.9
Grade is clearly the most important factor influencing outcome for spinal astrocytomas.14,15 Although prolonged survival is generally the rule for patients with low-grade astrocytomas, malignant astrocytomas such as glioblastoma multiforme (GBM) and highly anaplastic (Grade 3) astrocytomas (HAAs) are aggressive tumors characterized by rapid local recurrence following treatment, craniospinal axis dissemination, and short survival.13–17 The pilocytic astrocytoma subtype reportedly has a better prognosis than the diffuse fibrillary astrocytoma subtype.18
Diagnostic and Staging Studies
The workup for primary cord tumors is chiefly radiographic. Magnetic resonance imaging (MRI) with gadolinium for contrast is the imaging study of choice.19 Standard or metrizamide-enhanced computed tomographic (CT) myelography is indicated for patients unable to undergo MRI (e.g., pacemaker-dependent patients). Intramedullary tumors usually extend for multiple segments of cord, and sagittal MRI is particularly useful for delineating the rostral and caudal extent of tumor. Imaging of the entire craniospinal axis is required for all ependymomas, because spinal axis metastases develop in 5% to 15% of intracranial ependymomas.20 These spinal “drop metastases” can produce the initial symptoms of a disseminated, primary intracranial ependymoma.
The entire neuraxis should also be imaged for cord HAAs and GBMs. Low-grade spinal astrocytomas and oligodendrogliomas do not require imaging of the entire craniospinal axis. CT scanning of the chest to rule out pulmonary metastases should be performed for vertebral osteogenic sarcomas and chondrosarcomas, as well as for spinal canal neurofibrosarcomas. Other tests such as angiography may be of limited use for AVMs or meningiomas. CSF cytology may be informative, but its use in determining treatment or influencing outcome is controversial. No chemical markers appear to be clinically useful in the diagnosis or follow-up of spinal tumors. The role of the bromodeoxyuridine (BrdU) and Ki-67 labeling indices as prognostic indicators for spinal ependymomas is under investigation.21,22 An elevated cyclin D1 labeling index, high MIB-1 proliferation index, and p53 immunolabeling might be indicative of high grade in ependymomas, although a correlation with clinical outcome is unclear.5,23
Staging System
Presently there is no commonly accepted TNM-type staging system for primary cord tumors. Primary vertebral body tumors and spinal canal tumors should be staged using the American Joint Committee on Cancer (AJCC) staging systems for bone and soft tissues, respectively.24
Standard Therapeutic Approaches
Surgical exploration combining maximal resection (preferably en bloc) with minimal risk of iatrogenic injury is the preferred initial treatment procedure. In addition to decompressing the cord, essential histologic information is obtained. There is no role for preoperative irradiation or irradiation without a prior attempt at histologic diagnosis. As many as 4% of radiographically diagnosed “cord gliomas” have proven to be benign upon pathologic examination, with infarcts, demyelinating processes, amyloidosis, and sarcoidosis comprising some of the disease processes which mimic gliomas.25
The extent of resection is variable for primary cord gliomas. Using modern microsurgical techniques with intraoperative monitoring, complete resection of low-grade gliomas has become more common, and outcome has been successful without the use of adjuvant radiotherapy.26,27,97 Postoperative megavoltage irradiation provides favorable long-term results following subtotal resection. Craniospinal irradiation is employed in the management of malignant as well as benign, multifocal ependymomas.
The favorable location of ependymomas of the cauda equina usually permits complete resection. Intramedullary ependymomas frequently have tissue planes separating the tumor and cord that facilitate complete resection, as well. Low-grade astrocytomas and oligodendrogliomas are infiltrative and generally lack tissue planes separating the tumor from normal cord. Aggressive resection in this situation can result in substantial neurologic injury, so subtotal resection or biopsy is often performed. However, aggressive resection has been performed successfully in some expert hands.28 If frozen sections suggest HAA or GBM, resection is usually abandoned after biopsy is performed.
Role of Radiation Therapy
The radiotherapeutic approach is different for each situation (Table 26-2). Postoperative irradiation is not indicated for completely resected low-grade gliomas.26,29,30 Incompletely resected low-grade astrocytomas and ependymomas are generally treated with postoperative focal irradiation. High-grade (malignant) astrocytomas are palliatively irradiated with focal fields. Malignant ependymoma and multifocal, benign ependymomas are treated with curative-intent, craniospinal irradiation.
Table 26-2 Radiotherapeutic Management of Primary Spinal Tumors
Type | Treatment and Total Radiation Dose, Gy* |
---|---|
Low-grade glioma,† complete resection | Observation |
Low-grade glioma,† subtotal resection | Focal field XRT,‡ 50.4 |
High-grade (malignant) glioma (HAA, GBM) | Focal field XRT, 54 |
Malignant ependymoma and benign multifocal ependymoma | Craniospinal XRT to 45, then 9 focal boost to gross tumor sites (54 total) |
Meningioma, completely resected | Observation |
Meningioma, subtotally resected | Observation vs. focal field XRT, 50.4-54, or charged particle beams,§ 52-54 Eq |
Spinal canal sarcomas and vertebral body chondrosarcomas, chordomas, osteogenic sarcomas | Charged particle beams,§ 60-72 Eq |
HAA, Highly anaplastic (Grade 3) astrocytoma; GBM, glioblastoma multiforme; XRT, x-ray therapy.
* Total dose prescribed using standard (1.8-Gy) once-daily fractions with megavoltage photons unless otherwise specified.
† Includes astrocytoma, ependymoma, and oligodendroglioma.
‡ Megavoltage photon irradiation.
Completely resected spinal canal meningiomas require no additional therapy, since the risk of recurrence is only on the order of 6%.31 However, subtotally resected meningiomas have a higher risk of local recurrence, making postoperative irradiation a reasonable consideration.
Nerve sheath sarcomas are approached in a similar manner: postoperative irradiation is generally withheld if the tumor has been completely resected. Particle beam irradiation is given if macroscopic tumor remains. Incompletely removed vertebral chondrosarcomas and chordomas are likewise irradiated with charged particles (see Table 26-2). Osteogenic sarcomas are treated with a combination of chemotherapy, surgery, and postoperative particle beam irradiation if complete resection is not achieved.
Simulation
Low-grade cord gliomas are treated with megavoltage photon fields encompassing the radiographically apparent lesion (gross target volume [GTV]) with a 3- to 5-cm margin of normal spinal cord (or brainstem for high cervical lesions) both rostrally and caudally (clinical target volume [CTV]). The preoperative sagittal MRI is the most useful study for determining the size and location of the GTV. Field width for non-IMRT cases rarely needs to exceed 7 to 8 cm, since only the cord requires irradiation. Sophisticated immobilization devices are useful when treating with IMRT, and thermoplastic face masks are useful for conventionally-treated tumors located in the cervical spine. A typical clinical, non-IMRT setup for a patient with a thoracic cord glioma is shown in Fig. 26-1.
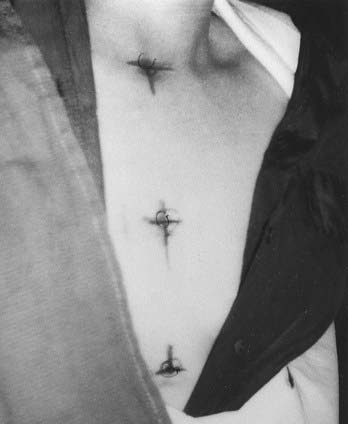
FIGURE 26-1 • Clinical setup showing cephalad and caudal field borders for a localized low-grade thoracic spinal cord glioma.
Whether to include an associated syrinx (a dilated, fluid-filled intramedullary cavity) in the treatment volume is controversial. At times, the syrinx results from local mass effect causing obstruction and dilation of the central canal of the spinal cord; in this situation, the syrinx is not actually part of the neoplastic process, but instead represents a reaction of normal tissue. At other times, the tumor itself may be from a cystic cavity or syrinx as part of the neoplastic process, and in this situation the syrinx must be included in the GTV treatment volume. Clinically distinguishing these situations from one another is often difficult; consultation with the neurosurgeon regarding his or her impression at the time of surgery is often beneficial. In general, a small syrinx can easily be included in the treatment volume. An extensive syrinx extending for virtually the entire length of the cord can be technically difficult to encompass with conventional treatment fields. IMRT can be particularly beneficial in this situation (Fig. 26-2).
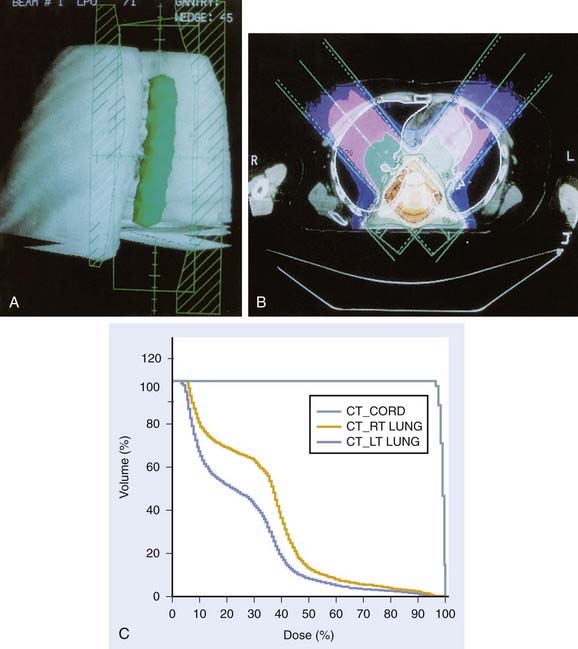
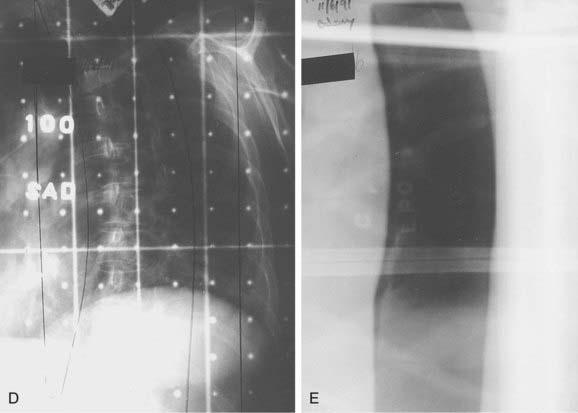
FIGURE 26-2 • A, Beam’s eye view achieved using three-dimensional conformal reconstruction of a thoracic cord glioma target volume and adjacent lungs with a posterior oblique wedged-pair irradiation technique. Custom low melting point alloy blocking or multileaf collimation is designed using the beam’s eye view of the target volume. B, Transverse section showing dosimetry from the treatment plan developed using the target volume derived from A. Note the substantial portion of lung included in the beam’s exit path at this level. C, Dose-volume histogram derived from the treatment plan shown in B using dosimetry information for the entire treatment volume. Virtually the entire target volume receives 100% of the total dose of 50.4 Gy at 1.8 Gy per fraction. Despite the impressive amount of lung included in the exit beam shown in B, the histogram shows that only 10% to 15% of each lung actually receives more than 25 Gy (50% of the total dose) under this plan. Nonetheless, 40% of the left lung and 60% of the right lung receives a dose greater than 15 Gy (30% of the total dose). See also Fig. 26-2. D, Verification simulator film taken with the beam’s eye view geometry based on the treatment plan developed in A, B, and C. E, Port film taken during treatment on the linear accelerator using the treatment field shown in D confirms the accuracy of the daily patient setup.
When IMRT is not utilized, tumors in the upper cervical spine are generally treated with opposed lateral fields to avoid unnecessary irradiation of the aerodigestive mucosa. Tumors located more caudally are often treated with differentially weighted anterior-posterior–posterior-anterior (AP-PA) fields using compensators. Use of beam-split abutting opposed lateral and AP-PA fields to treat tumors at the cervicothoracic junction is discouraged; the risk of overdosing the normal cord and underdosing the tumor owing to setup variation or error far outweighs any advantage in reducing acute morbidity. Again, IMRT is of particular benefit for tumors located in this region, eliminating the problems of possible overlap/underdosing inherent in the use of abutting fields (Fig. 26-3). When the use of abutting fields is unavoidable, a matching beam-split technique using independent jaws is required. The match line should be shifted 1 cm after every 10 Gy to minimize the risk of inadvertent overlap. A medulloblastoma-type “gap” technique should only be used when treating the craniospinal axis, and the gap should never be located over a site of macroscopic disease.
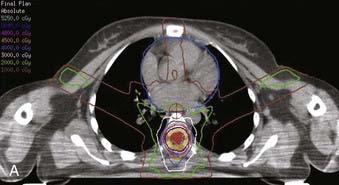
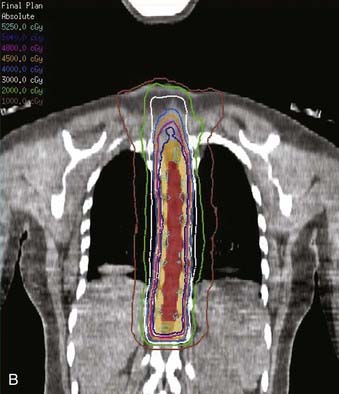
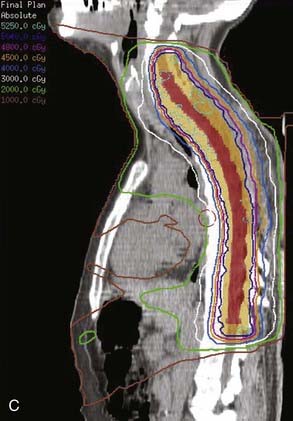
FIGURE 26-3 • The advantages of intensity modulated radiation therapy (IMRT) are highlighted by this difficult low-grade astrocytoma involving the thoracic cord, associated with a neoplastic syrinx extending cephalad through the cervicothoracic junction into the cervical cord. These three images demonstrate the superior dose-homogeneity IMRT provides within the high-dose treatment volume and the substantially greater sparing of adjacent normal structures throughout the treatment volume (compare the isodose curves involving the lung with the discussion in Fig. 26-2). Conventional treatment techniques are not able to provide this level of precise dose-localization, although, theoretically, stereotaxic body radiosurgery would provide similar advantages. A, Transverse image. B, Coronal image. C, Sagittal image. The 5040 cGy isodose line is shown in dark blue.
Wedged pair, posterior, oblique fields offer the theoretical advantage of decreased morbidity by minimizing exit dose in any given location; however, the planning and setup are technically more difficult, and scrupulous verification is necessary to ensure adequate coverage during treatment. Care must also be taken with this technique to ensure that the kidneys, liver, or substantial portions of the lungs are not irradiated beyond tolerance. The use of three-dimensional (3D) conformal treatment planning with dose-volume histograms has greatly improved the reliability and safety of this technique (see Fig. 26-2).
It is worth noting that a series from Iowa with small numbers of cauda ependymomas found that adjuvant thecal sac irradiation was beneficial when piecemeal rather than en bloc resection was performed.32 However, several other series found no advantage to the use of traditional extended fields over focal fields.8,33 Following an uncomplicated resection, the use of fields extended inferiorly and laterally to encompass the entire sacrum appear unnecessary from both a clinical and theoretical standpoint.