Solid Organ Transplantation
At the conclusion of this chapter, the reader should be able to:
• Identify and describe the histocompatibility antigens.
• Explain the clinical applications of histocompatibility antigens and human leukocyte antigens.
• Identify and describe several laboratory methods for evaluating potential transplant recipients and donors.
• List frequently used terms in transplantation.
• Identify various types of transplants.
• Define graft-versus-host disease.
• Explain the etiology, epidemiology, signs and symptoms, manifestations, diagnosis, and prevention of graft-versus-host disease.
• Describe the types of graft rejection.
• Briefly explain the mechanism of organ or tissue rejection.
• Identify and explain some methods of immunosuppression.
• Analyze a representative transplantation case study.
• Correctly answer case study related multiple choice questions.
• Be prepared to participate in a discussion of critical thinking questions.
• Explain the principle and application of the Longitudinal Assessment of Posttransplant Protocol.
At present, a variety of tissues and organs are transplanted in human beings, including bone marrow, peripheral stem cells, bone matrix, skin, kidneys, liver, cardiac valves, heart, pancreas, corneas, and lungs. Transplantation is one of the areas, in addition to hypersensitivity (Chapter 26) and autoimmunity (Chapter 28), in which the immune system functions in a detrimental way.
Histocompatibility Antigens
The major histocompatibility complex (MHC) is a cluster of genes found on the short arm of chromosome 6 at band 21 (6p21; see Fig 2-1). These genes code for proteins that have a role in immune recognition.
Nomenclature of Human Leukocyte Antigen Alleles
Each HLA allele has a unique four-, six-, or eight-letter or digit name (Table 31-1). The length of the allele designation depends on the sequence of the allele and that of its nearest relative. All alleles receive a four-letter or digit name; six- and eight-digit names are only assigned when necessary.
Table 31-1
Nomenclature | Indicates |
HLA | Human leukocyte antigen (HLA) region and prefix for an HLA gene |
HLA-DRB1 | Particular HLA locus (e.g., DRB1) |
HLA-DRB1∗13 | Group of alleles that encode the DR13 antigen |
HLA-DRB1∗1301 | Specific HLA allele |
HLA-DRB1∗1301N | Null allele |
HLA-DRB1∗130102 | Allele that differs by a synonymous mutation |
HLA-DRB1∗13010102 | Allele that contains a mutation outside the coding region |
HLA-A∗2409N | Null allele |
HLA-A∗3014L | Allele encoding a protein with significantly reduced or low cell surface expression |
HLA-A∗24020102L | Allele encoding a protein with significantly reduced or low cell surface expression, where the mutation is found outside the coding region |
HLA-B∗44020102S | Allele encoding a protein expressed as a secreted molecule only |
HLA-A∗3211Q | Allele that has a mutation previously shown to have a significant effect on cell surface expression, but where this has not been confirmed and its expression remains questionable |
∗As of June 2007, no alleles have been named with the “C” or “A” suffixes.
Major Histocompatibility Complex Regions
The MHC is divided into four major regions (Table 31-2)—D, B, C, and A. The A, B, and C regions are the classic or class Ia genes that code for class I molecules. The D region codes for class II molecules. Class I includes HLA-A, B, and C. The three principal loci (A, B, and C) and their respective antigens are numbered 1, 2, 3, and so on. The class II gene region antigens are encoded in the HLA-D region and can be subdivided into three families, HLA-DR, HLA-DC (DQ), and HLA-SB (DP).
Table 31-2
Examples of Nomenclature of HLA Alleles
Allele (New Nomenclature) | Frequently Used Shorthand |
Class I | |
HLA-A∗0101 | HLA-A1 |
HLA-B∗0801 | HLA-B8 |
Class II | |
HLA-DRB1∗0101 | HLA-DR1 |
HLA-DRB1∗0301 | HLA-DR3 |
Adapted from Peakman M, Vergani D: Basic and clinical immunology, ed 2, New York, 2009, Churchill Livingstone.
Classes of Human Leukocyte Antigen Molecules
Structurally, there are two classes of HLA molecules, class I and class II (Table 31-3). Both classes are cell surface heterodimeric structures. Class I HLA molecules consist of an alpha chain, a highly polymorphic glycoprotein, encoded within the MHC on chromosome 6. This alpha chain noncovalently associates with beta-2 microglobulin, a nonpolymorphic glycoprotein, encoded by a non-HLA gene on chromosome 15. Class II HLA molecules are composed of alpha chains and beta chains encoded within the MHC. The conformation of class I and class II HLA molecules provides each with a groove in which linear peptides, consisting of 8 to 25 peptides, are displayed for recognition by the cell surface expression on lymphocytes of a transmembrane heterodimeric receptor. All nucleated cells of the body display transmembrane class I HLA molecules in association with the non–transmembrane beta-2 microglobulin molecule.
Table 31-3
Comparison of Major Histocompatibility Complex Class I and Class II
Parameter | Class I | Class II |
Loci | HLA-A, B, and C | HLA-DN, DO, DP, DQ, and DR |
Distribution | Most nucleated cells | B lymphocytes, macrophages, other antigen-presenting cells, activated T lymphocytes |
Function | To present endogenous antigen to cytotoxic T lymphocytes | To present endogenous antigen to helper T lymphocytes |
Human Leukocyte Antigen Applications
HLA testing has increasingly been used as a diagnostic and genetic counseling tool. Knowledge of HLA antigens and their linkage has become important because of the recognized association of certain antigens (Box 31-1) with distinct immunologic-mediated reactions, autoimmune diseases, some neoplasms, and other disorders; these disorders, although nonimmunologic, are influenced by non-HLA genes also located within the major MHC region.
The estimated relative risks or chances of developing a disease if a given antigen is present may be elevated in individuals bearing certain HLA antigens compared to individuals who lack the antigen (Table 31-4). The HLA-B27 antigen is the only HLA antigen with a disease association strong enough to be useful in differential diagnosis. Although the degree of association between HLA antigens and other diseases may be statistically significant, it is not strong enough to be of diagnostic or prognostic value.
Table 31-4
Relationship of Human Leukocyte Antigens to Risk of Disease
Antigen Present | Related Disease | Risk∗ |
B27 | Ankylosing spondylitis Reiter’s syndrome Anterior uveitis Arthritic infection with Yersinia or Salmonella Psoriatic arthritis with spinal involvement Spondylitis associated with inflammatory bowel disease Juvenile chronic arthritis with spinal involvement |
100׆ 40× 25× 20× 11× 9× 5× |
B8 | Celiac disease Addison’s disease Myasthenia gravis Dermatitis herpetiformis Chronic active hepatitis Sjögren’s syndrome Diabetes mellitus (insulin dependent) Thyrotoxicosis |
9× 6× 5× 4× 4× 3× 2× 2× |
B5 | Behçet’s syndrome | 6× |
BW38 | Psoriatic arthritis | 7× |
BW15 | Diabetes mellitus (insulin-dependent) | 3× |
DR2 | Goodpasture’s syndrome Multiple sclerosis |
16× 4× |
DR3 | Gluten-sensitive enteropathy Dermatitis herpetiformis Subacute cutaneous lupus erythematosus Addison’s disease Sjögren’s syndrome (primary) |
21× 14× 12× 11× 10× |
DR4 | Pemphigus‡ Giant cell arthritis Rheumatoid arthritis Juvenile (insulin-dependent) diabetes mellitus |
32× 8× 6× 5× |
DR5 | Pauciarticular juvenile arthritis Scleroderma Hashimoto’s thyroiditis |
5× 5× 3× |
∗Increased risk of developing the disease over a lifetime.
†Varies with ethnic group (e.g., 3× for Pima Indians and 300× for Japanese).
Adapted from Ashman RF: Rheumatic diseases. In Lawlor GJ, Fischer TJ, editors: Manual of allergy and immunology, ed 2, Boston, 1998, Little, Brown.
Laboratory Evaluation of Potential Transplant Recipients and Donors
Human Leukocyte Antigen Typing
A potential recipient needs to have HLA typing (Fig. 31-1, A). A family search may be conducted for a suitable donor. If a suitable match is not found, the patient is placed on a waiting list (see Fig. 31-1, B). When an organ becomes available, the donor is HLA-typed and a computerized search is made for a suitable recipient (see Fig. 31-1, C).
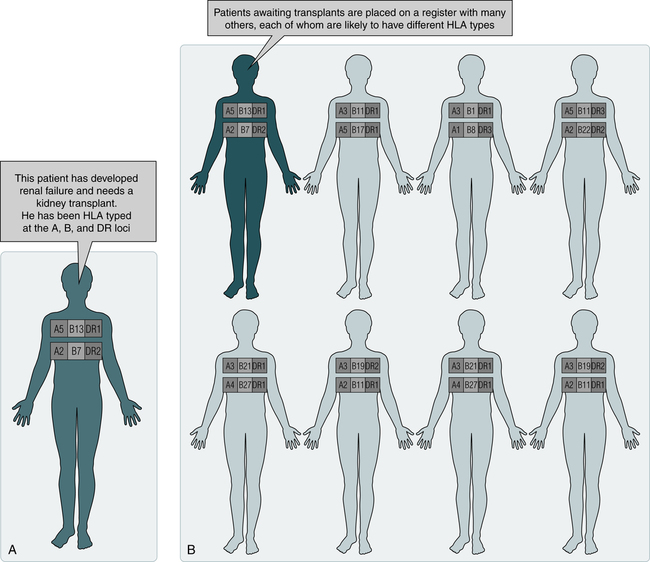
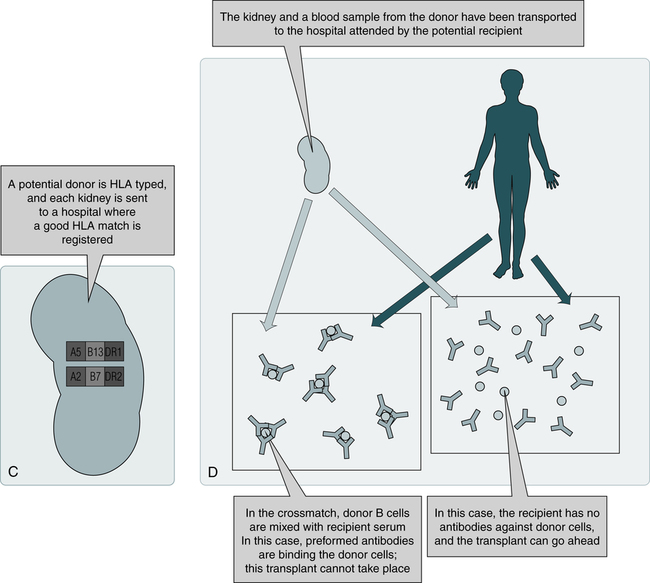
C, When a potential organ becomes available, the donor is HLA-typed. If the donor and recipient demonstrate a suitable match by computer, a blood sample is procured from the donor and crossmatched with the recipient’s blood to determine compatibility. D, If no HLA antibodies are detected against the donor cells, the organ is harvested and transplanted to the recipient. (From Nairn R, Helbert M: Immunology for medical students, ed 2, St Louis, 2007, Mosby.)
Histocompatibility Testing
Because different individuals in a species carry different HLA antigens on their cell surfaces, introduction of foreign antigens can stimulate T cells. These T cells are prominently implicated in graft rejection, and they can also stimulate antibody formation under certain circumstances. Histocompatibility crossmatching is performed to rule out preexisting antibodies capable of causing hyperacute rejection (see Fig. 31-1, D).
Flow Cytometry
Single-cell analysis by flow cytometry is the most sensitive method for crossmatching and antibody identification (see Chapter 13). Tagged T or B lymphocytes are incubated with the patient’s serum to allow the formation of antigen-antibody complexes on the cell surface. Unbound proteins are washed away and the bound antibodies are detected with a second antibody, anti–human immunoglobulin G (IgG) labeled with a chromophore. An alternative flow cytometry format uses microparticles coated with HLA antigens of known specificity (obtained through recombinant techniques) instead of lymphocytes.
Facts About Solid Organ Transplantation
How long a patient waits for a transplant depends on the following factors:
• Blood type (some rarer than others)
• Height and weight of transplant candidate
• Distance between donor’s hospital and potential donor organ
• Number of donors in local area over time
• Transplantation center’s criteria for accepting organ offers
Depending on the type of organ needed, some factors are more important than others.
On March 22, 2012, the United Network for Organ Sharing patient waiting list contained 113,612 names. The list continues to grow because of the scarcity of organs (Box 31-2). Most of these registrants are waiting for a kidney transplant, followed by those waiting for a liver transplant and heart transplant. Other transplant registrants are waiting for lung, kidney and pancreas, pancreas, pancreatic islet cell, heart and lung, and intestine. Approximately 25% of patients waiting for a liver transplant are children younger than 10 years.
The number of patients living with a function graft has generally increased over the last decade. Graft survival time depends on many factors, including the type of organ transplanted (Fig. 31-2 and Table 31-5).
Table 31-5
Follow–up Period | |||||
3 Months | 1 Year | 3 Years | 5 Years | 10 Years | |
TX 2007-2008 | TX 2007-2008 | 2005-2008 | 2003-2008 | 1998-2008 | |
Kidney: Deceased Donor Kidney: Living Donor |
95.6 98.0 |
91.7 96.5 |
81.8 90.5 |
70.8 82.8 |
44.9 61.2 |
Pancreas | 85.0 | 74.8 | 62.6 | 52.0 | 35.1 |
Liver: Deceased Donor | 91.7 | 85.2 | 75.1 | 68.5 | 54.8 |
Liver: Living Donor | 91.3 | 88.2 | 80.1 | 74.6 | 59.6 |
Intestine | 91.3 | 81.7 | 55.2 | 41.5 | 24.0 |
Heart | 93.1 | 88.3 | 80.7 | 73.9 | 54.7 |
Lung | 91.6 | 81.5 | 63.3 | 51.2 | 26.1 |
Tx = year or inclusive years of tranplantation.
Source: Organ Procurement and Transplantation Network (OPTN) and Scientific Registry of Transplant Recipients (SRTR). OPTN / SRTR 2010 Annual Data Report. Rockville, MD: Department of Health and Human Services, Health Resources and Services Administration, Healthcare Systems Bureau, Division of Transplantation; 2011. www.srtr.org/annual_reports/2010
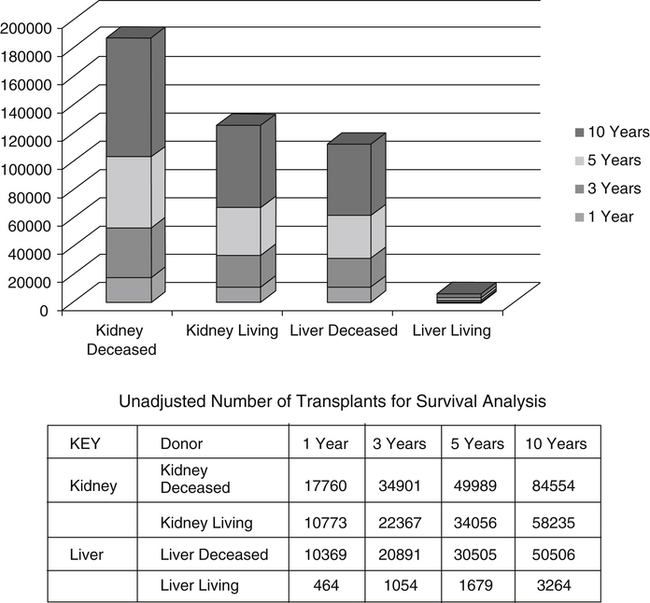
Transplantation Terminology
The transplanting or grafting of an organ or tissue ranges from self-transplantation, such as skin grafts from one part of the body to another to correct burn injuries, or hair transplants from one area of the scalp to another to correct pattern baldness, to the grafting of a body component from one species to another, such as transplanting a pig’s heart valve to a human. Table 31-6 defines the most recent terms used in transplantation.
Table 31-6
Term | Definition |
Autograft | Graft transferred from one position to another in the same individual (e.g., skin, hair, bone) |
Syngraft | Graft transplanted between different but identical recipient and donor (e.g., kidney transplant between monozygous twins) |
Allograft (homograft) | Graft between genetically different recipient and donor of the same species; grafted donor tissue or organ contains antigens not present in recipient |
Xenograft (heterograft) | Graft between individuals of different species (e.g., pig heart valve to a human heart) |
Types of Transplants
Eleven different organs or human body parts can be transplanted—blood vessels, bone, bone marrow or stem cells (see Chapter 32), cornea, heart, kidneys, liver, lung, middle ear, pancreas, and skin. Successful organ transplants have increased since the advent of the immunosuppressive drug cyclosporine (cyclosporin A).
Graft-Versus-Host Disease
Etiology
When immunocompetent T lymphocytes are transfused from a donor to an immunodeficient or immunosuppressed recipient, the transfused or grafted lymphocytes recognize that the antigens of the host are foreign and react immunologically against them (Table 31-7). Instead of the usual transplantation reaction of host against graft, the reverse graft-versus-host reaction occurs and produces an inflammatory response.
Table 31-7
Requirements for Potential Graft-versus-Host Disease
Factor | Comments |
1. Source of immunocompetent lymphocytes | Blood products, bone marrow transplant, organ transplant |
2. Human leukocyte antigen differences between patient and recipient | The stronger the antigen difference, the more severe the reaction. |
3. Inability to reject donor cells | Patients are severely immunocompromised or immunosuppressed. |
Prevention
High-Risk Patients
• Recipients of autologous or allogeneic bone marrow grafts. Recipients of autologous bone marrow may be expected to have the same risk of posttransfusion GVHD as patients receiving allogeneic bone marrow.
• Children with severe congenital immunodeficiency syndromes involving T lymphocytes. The degree of immunodeficiency in the host, rather than the number of transfused immunocompetent cells, determines whether GVHD will occur.
Intermediate-Risk Patients
Patients considered to be at a lower risk of developing GVHD include the following:
• Infants receiving intrauterine transfusions, followed by exchange transfusions, and possibly infants receiving only exchange transfusions. The immune mechanism of the fetus and newborn may not be sufficiently mature to reject foreign lymphocytes and prior transfusions may induce a state of immune tolerance in the newborn. Transfused lymphocytes may continue to circulate for a prolonged period in some immunologically tolerant hosts without the development of GVHD. There is insufficient evidence to recommend irradiation of blood given to all premature infants.
• Patients receiving total-body radiation or immunosuppressive therapy for disorders such as lymphoma and acute leukemia. Although routine irradiation of blood products given to these patients can be justified, it cannot be regarded as absolutely indicated because the risk of developing GVHD is so low. Blood product irradiation, however, is advised for selected patients with hematologic malignancies, especially when transfusions are given during or near the time of sustained and severe therapy-induced immunosuppression.
Low-Risk Patients
Patients also at risk but considered the least susceptible include the following:
• Patients with solid tumors. The incidence of the development of GVHD in these patients is difficult to determine. However, it has developed in nonhematologic malignancies such as neuroblastoma. In one case, GVHD developed after infusion of a single unit of packed red blood cells (RBCs).
• Patients with aplastic anemia receiving antithymocyte globulin theoretically may be at increased risk of posttransfusion GVHD during therapy-induced periods of lymphocytopenia.
• Although a theoretical risk of posttransfusion GVHD may exist in patients with acquired immunodeficiency syndrome (AIDS), the disease has not actually been observed in this disorder. The routine use of irradiated blood is not recommended.
Immunologic Tolerance
The importance of tolerance to self antigens was recognized early in the study of immunology. Immunologic tolerance is the acquisition of nonreactivity toward particular antigens. Self-recognition (tolerance) is a critical process, and the failure to recognize self antigens can result in autoimmune disease (see Chapter 28).
T Cell Tolerance
1. Clonal abortion. Immature T cell clones may be aborted in a manner similar to that of B cells.
2. Functional deletion. The subsets of a mature T cell may be individually deleted, leading to the loss of only one of the functions of the T cell group.
3. T cell suppression. T cell suppressors actively suppress the actions of other T cell subsets or B cells.
B Cell Tolerance
The pathways of B cell tolerance are as follows:
1. Clonal abortion. A low concentration of multivalent antigen may cause the immature clone to abort. Tolerance of immature B cells by this mechanism is high.
2. Clonal exhaustion. Repeated antigen challenge with a T-independent antigen may remove all mature functional B cell clones. Tolerance of mature B cells is moderate.
3. Functional deletion. The combined absence of the helper T subset and presence of T-dependent antigen (or with T suppressor cells), or an excess of T-independent antigen, prevents mature B cells from functioning normally. The ability to tolerize B cells by this mechanism is moderate.
4. Antibody-forming cell blockade. An excess of T-independent antigen interferes with the secretion of antibody by antibody-forming cells. B cell tolerance by this mechanism is low.
Graft Rejection
Organs vary with respect to their susceptibility to rejection based on inherent immunogenicity (Box 31-3), which is influenced by factors such as vascularity.
The role of sensitized lymphocytes and antibodies in graft rejection differs and is influenced by the type of organ transplanted. Lymphocytes, particularly recirculating small lymphocytes, are effective in shortening graft survival. Cell-mediated immunity is responsible for the rejection of skin and solid tumors. However, humoral antibodies can also be involved in the rejection process. The complexity of the action and interaction of cellular and humoral factors in grafts is considerable. Five possible categories of graft rejection have been demonstrated in human kidney transplant rejection—hyperacute, accelerated, acute, chronic, and immunopathologic (Table 31-8; Color Plate 17).
Table 31-8
Categories and Characteristics of Graft Rejection Based on Immune Destruction of Kidney Grafts
Type | Time of Tissue Damage | Predominant Mechanism | Cause |
Hyperacute | Within minutes | Humoral | Preformed cytotoxic antibodies to donor antigens |
Accelerated | 2-5 days | Cell-mediated | Previous sensitization to donor antigens |
Acute | 7-21 days | Cell-mediated (possibly antibody cell-mediated cytotoxicity) | Development of allogeneic reaction to donor antigens |
Chronic | Later than 3 mo | Cell-mediated | Disturbance of host-graft tolerance |
Immunopathologic damage to the new organ | Later than 3 mo | 1. Immune complex disorder 2. Complex formation with soluble antigens |
Immunopathologic mechanisms related to circumstances necessitating transplantation |
First-Set and Second-Set Rejections
Skin transplantation is the most common experimental model for transplantation research (Fig. 31-3). Rejection of skin and solid tumors can be divided into first-set and second-set rejections. Activation of cellular immunity by T cells is the predominant cause of the first-set allograft rejection. Lymphocytes can directly attack cellular antigens to which they are sensitized by previous exposure or by cytotoxic lymphokines. The primary role of lymphocytes in first-set rejection is consistent with the histology of early reaction and shows infiltration by mononuclear cells, with very few polymorphonuclear leukocytes or plasma cells. Sensitization occurs within the first few days of transplantation, and the tissue is lost in 10 to 20 days.
Mechanisms of Rejection
General Characteristics
Rejection of a graft displays the following two key features of adaptive immunity:
Immunosuppression
Immunosuppression is used for the following:
Forms of immunosuppression include chemical (Box 31-4), biologic, and irradiation of the lymphoid system or the donated organ. The immunosuppressive activities of therapeutic agents used in transplantation directly interfere with the allograft rejection response. The problem arising from all immunosuppressive techniques is that the person is more susceptible to infection. If infection occurs, immunosuppression must be suspended, at which time allogeneic reactions frequently develop.
Immunosuppressive measures may be antigen-specific or antigen-nonspecific (Table 31-9). Antigen-nonspecific immunosuppression includes drugs and other methods of specifically altering T cell function. Many cytotoxic drugs are primarily active against dividing cells and therefore have some functional specificity for any cells activated to divide by donor antigens. The use of these drugs is limited by the toxic effects that they may have on other dividing cells or on the physiologic functioning of organs such as the liver.
Table 31-9
Types of Immunosuppressive Treatment
Drug | Mechanism of Action |
Corticosteroids | Reduce inflammation by inhibiting macrophage cytokine secretion |
Cyclosporine and FK506 | Blocks T cell cytokine production by inhibiting the phosphatase calcineurin and then blocking activation of the NFAT transcription factor |
Mycophenolate mofetil | Blocks lymphocyte proliferation by inhibiting guanine nucleotide synthesis in lymphocytes |
Rapamycin | Blocks lymphocyte proliferation by inhibiting IL-2 signaling |
Anti-CD3 monoclonal antibody | Depletes T cells by binding to CD3 and promoting phagocytosis or complement-mediated lysis (used to treat acute rejection) |
Anti–IL-2 receptor antibody | Inhibits T cell proliferation by blocking IL-2 binding; may also opsonize and help eliminate activated IL-2R-expressing T cells |
CTLA4-Ig Nulojix (belatacept) |
Inhibits T cell activation by blocking B7 costimulator binding to T cell CD28 (clinical trials) A selective T cell costimulation blocker |
FDA approves Nulojix for kidney transplant patients www.fda.gov/NewsEvents
Adapted from Abbas AK, Lichtman AH: Basic immunology, ed 3, St Louis, 2011, Saunders.
Cytotoxic Drugs
Cytotoxic drugs are the most common form of therapy and usually include alkylating agents, purine and pyrimidine analogues (Fig. 31-4), folic acid analogues, or the alkaloids. The drugs of choice, excluding alkylating drugs, are azathioprine, 6-mercaptopurine, 6-thioguanine, 5-fluorouracil, cytosine arabinoside, methotrexate and aminopterin, and vinblastine and vincristine.
Immunosuppressive Protocols
Protocols for immunosuppression of transplant recipients vary widely, depending on the transplantation center, type of organ transplanted, after transplantation, underlying cause of organ failure, and preexisting conditions (Box 31-5). Protocols are becoming more complex because of more immunosuppressive drug choices. In general, protocols include the following:
• Lymphokine synthesis inhibitors (e.g., cyclosporine, tacrolimus).
• Nucleoside synthetase inhibitors (e.g., azathioprine, mycophenolate mofetil).
• Steroids (e.g., prednisone).
• Induction or pretransplantation therapy—may include antithymocyte globulin, CD3 or CD25, or dacliximab
Xenotransplantation
Xenotransplantation is any procedure that involves the transplantation, implantation or infusion into a human recipient of either (a) live cells, tissues, or organs from a nonhuman animal source, or (b) human body fluids, cells, tissues or organs that have had ex vivo contact with live nonhuman animal cells, tissues or organs. The development of xenotransplantation is, in part, driven by the fact that the demand for human organs for clinical transplantation far exceeds the supply (Box 31-6). There is a global shortage of organs for transplantation. Pig heart valves are already used to repair human hearts, and porcine pancreatic islet cells are used to treat diabetes, so it is not a big leap to envision trans-species, whole-organ transplantation. Pigs are considered the most likely organ transplant donors for human beings because their organs are similar in size to human organs, they are easy to breed, and the extensive biologic differences between pigs and human beings make it unlikely for porcine diseases to infect human beings.
Biomarkers for Rejection
• Detecting a prerejection profile that will allow therapeutic interventions before rejection causes graft dysfunction
• Improving the sensitivity and specificity of rejection diagnosis
• Developing new classification systems for rejection that will improve prognosis
• Providing information for designing individualized immunosuppressive regimens that could prevent rejection while minimizing drug toxicity
• Performing a Longitudinal Assessment of Posttransplant Immune Status (see Procedural Protocol)
Longitudinal Assessment of Posttransplant Immune Status
Principle
• A noninvasive means of providing a biomarker of patient cellular immune status over time is the ImmuKnow assay (Figure 31-5).
Clinical Application
• This assay assists in identification of transplant patients who are at risk of developing an infection due to over-immunosuppression. Periodic monitoring (Table 31-10) guides clinicians in making therapeutic decisions that avoid overimmunosuppression and underimmunosuppression.
Table 31-10
Patient Immune System Monitoring∗
Time | Interval |
Pretransplant | Test as needed |
Months 1-6 | Test every 2 weeks |
Months 7-12 | Test monthly |
After year 1 | Perform routine monitoring (at minimum, test quarterly) |
∗Additional assays may be required in the event of changes in clinical status or posttransplant complications. This information is based on therapeutic drug monitoring recommendations described in immunosuppressant agent prescribing information.
From Cylex, Inc (with permission) www.cylex.net. Accessed August 22, 2012.
Chapter Highlights
• All vertebrates capable of acute rejection of foreign skin grafts possess a localized complex involving many genes that exert major control over the organism’s immune reactions.
• Some of these antigens are much more potent than others in provoking an immune response and therefore are called the major histocompatibility complex (MHC). In human beings, the MHC is referred to as human leukocyte antigens (HLAs).
• The MHC is divided into four major regions—D, B, C, and A. The A, B, and C regions code for class I molecules, whereas the D region codes for class II molecules.
• Class I and class II antigens can be found on surface membrane proteins of body cells and in body fluids.
• The MHC gene products have an important role in clinical immunology. For example, transplants are rejected if performed against MHC barriers; thus, immunosuppressive therapy is required. These antigens are of primary importance in influencing the genetic basis of survival or rejection of transplanted organs.
• Although HLA was originally identified by its role in transplant rejection, it is now recognized that the products of HLA genes play a crucial role in our immune system. T cells do not recognize antigens directly but do so when the antigen is presented on the surface of an antigen-presenting cell (APC), the macrophage. In addition to presenting the antigen, the macrophage must present another molecule for this response to occur. This molecule is a cell surface glycoprotein coded in each species by the MHC.
• T cells are able to interact with the histocompatibility molecules only if they are genetically identical (MHC restriction). Both class I and class II antigens function as targets of T lymphocytes that regulate the immune response.
• Class I molecules regulate interaction between cytolytic T cells and target cells; class II molecules restrict the activity of regulatory T cells (helper, suppressor, and amplifier subsets).
• Class II molecules regulate the interaction between helper T cells and APCs.
• HLA matching is of value in organ transplantation and in the transplantation of bone marrow.
• Transplantation is one of the areas (in addition to hypersensitivity and autoimmunity) in which the immune system functions in a detrimental way. Tissues and organs transplanted include peripheral stem cells or bone marrow, bone matrix, skin, kidneys, liver, cardiac valves, heart, pancreas, corneas, and lungs.
• Host immunity to the donor can cause graft-versus-host disease (GVHD), believed to result from the patient being sensitized to unshared HLA antigens before transplantation or transfusion. When allogenic T lymphocytes are transfused from donor to recipient with a graft or blood transfusion, the patient can develop acute or chronic GVHD. Patients at risk for GVHD include those who are immunodeficient or immunosuppressed with severe lymphocytopenia and bone marrow suppression.
• Immunologic tolerance is the acquisition of nonreactivity toward particular antigens. Self-recognition (tolerance) is a critical process; the failure to recognize self antigens can result in autoimmune disease.
• Immunosuppressive measures may be antigen-specific or antigen-nonspecific. Antigen-nonspecific immunosuppression includes drugs and other methods of specifically altering T cell function. Immunosuppressive measures directed at T cells include the use of ATG at the time of transplantation and of lymphoid irradiation before transplantation.