Chapter 68 Sleep and Its Disorders
In the first part of this chapter, a brief overview of sleep is given, covering the definition and architecture of sleep, sleep habits and requirements, the ontogeny of sleep, dreams and sleep, the neurobiology of sleep and wakefulness, circadian rhythm and chronobiology of sleep, and functions of sleep. In the sections that follow, physiological changes in sleep are summarized, sleep deprivation, sleepiness, and causes/consequences of excessive daytime sleepiness are discussed, sleep disorders are classified, and the approach to a patient with sleep complaints is outlined. Finally, the clinical phenomenology of a variety of sleep disorders is covered, and the chapter concludes with recommendations for laboratory assessment and treatment of selected sleep disorders. For more in-depth information about the order and disorders of sleep, the reader is referred to the growing number of sleep medicine texts (Avidan and Zee, 2006; Chokroverty, 2009a; Chokroverty et al., 2003; Kryger et al., 2005; Lee-Chiong, 2006; Montagna and Chokroverty, 2011).
Definition of Sleep and Moment of Sleep Onset
Modern researchers define sleep on the basis of both behavioral and physiological criteria (Chokroverty, 2009b) (Table 68.1). The behavioral criteria include lack of mobility or slight mobility, closed eyes, a characteristic species-specific sleeping posture, reduced response to external stimulation, quiescence, increased reaction time, elevated arousal threshold, impaired cognitive function, and a reversible unconscious state. Physiological criteria (see Sleep Architecture and Sleep Stages, later) are based on EEG, electro-oculography (EOG), and electromyography (EMG) findings as well as other physiological changes in ventilation and circulation.
Sleep Architecture and Sleep Stages
In their 1968 criteria, Rechtschaffen and Kales (RK) divided NREM sleep into stages 1, 2, 3, and 4. In 2007, this staging was modified slightly by an American Academy of Sleep Medicine (AASM) Task Force, and NREM sleep is now divided into three stages: N1, N2, and N3 (slow-wave sleep). In the modified staging criteria adopted by the AASM (Iber et al., 2007), the traditional RK stage 1 NREM sleep was labeled N1, RK stage 2 was labeled N2, and RK stages 3 and 4 were combined into one stage, N3.
NREM sleep accounts for 75% to 80% of sleep time in adult humans. In stage N1 sleep, making up approximately 3% to 8% of sleep time, the alpha rhythms (8-13 Hz) characteristic of wakefulness (Fig. 68.1) diminish to less than 50% in an epoch (i.e., a 30-second segment of a polysomnographic (PSG) tracing with a paper or monitor speed of 10 mm/sec), and a mixture of slower theta rhythms (4-7 Hz) and beta waves (>13 Hz) appear. EMG activity decreases slightly, and slow, rolling eye movements may be recorded (Fig. 68.2). Vertex sharp waves are noted toward the end of stage N1 sleep. Stage N2 sleep begins after approximately 10 to 12 minutes of stage N1 sleep. The characteristic EEG findings of stage N2 sleep include sleep spindles (12-18 Hz, most often 14 Hz) and K complexes intermixed with vertex sharp waves (Fig. 68.3). The EEG recording contains theta activity and fewer than 20% slow waves (0.5-2 Hz). Stage N2 sleep lasts for approximately 30 to 60 minutes. During stage N3 sleep, the slow waves occupy 20% to 100% of the epoch (Fig. 68.4). Toward the end of slow-wave sleep, or N3, body movements are registered as artifacts in the PSG recordings.
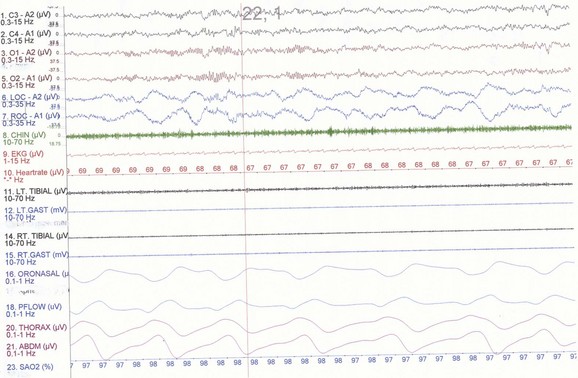
Fig. 68.2 Polysomnographic recording shows stage 1 non–rapid eye movement (NREM) sleep (N1) in an adult. Electroencephalograms (top 4 EEG channels) show a decrease of alpha activity to less than 50% and low-amplitude beta and theta activities. Left and right electro-oculograms (EOG) show slow rolling eye movements. A1, Left ear; A2, right ear; Thorax, respiratory effort (chest). Rest of montage is same as in Fig. 68.1.
(From Chokroverty, S., 2009. Sleep Disorders Medicine: Basic Science, Technical Considerations, and Clinical Aspects, third ed. Saunders, Philadelphia, Fig. 2.2.)
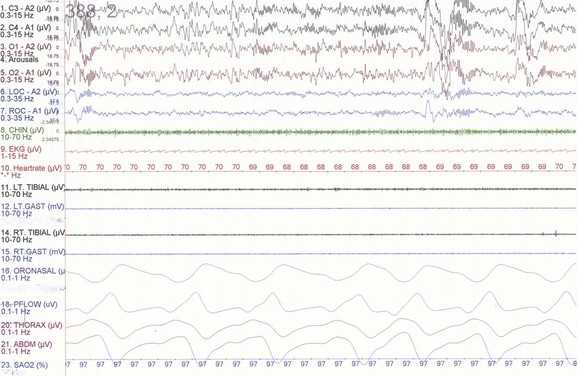
Fig. 68.3 Polysomnographic recording shows stage 2 non–rapid eye movement (NREM) sleep (N2) in an adult. Note approximately 14-Hz sleep spindles and K complexes intermixed with delta waves (0.5-2 Hz) and up to 75 µV in amplitude occupying less than 20% of the epoch. See Fig. 68.2 for description of rest of montage.
(From Chokroverty, S., 2009. Sleep Disorders Medicine: Basic Science, Technical Considerations, and Clinical Aspects, third ed. Saunders, Philadelphia, Fig. 2.3.)
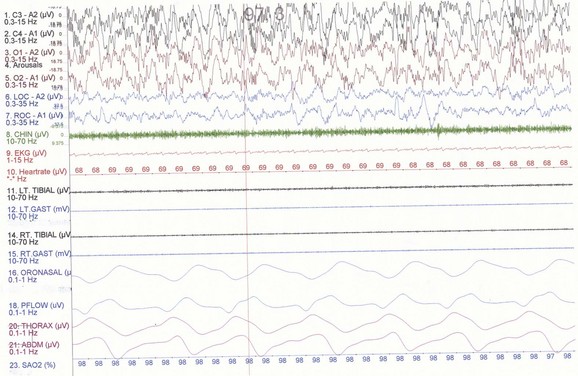
Fig. 68.4 Polysomnographic recording shows stage 4 non–rapid eye movement (NREM) sleep (N3) in an adult. Delta waves occupy more than 50% of the epoch in the traditional R&K scoring technique. See Fig. 68.2 for description of the montage.
(From Chokroverty, S., 2009. Sleep Disorders Medicine: Basic Science, Technical Considerations, and Clinical Aspects, third ed. Saunders, Philadelphia, Fig. 2.5.)
The first REM sleep (R sleep) episode is noted 60 to 90 minutes after the onset of sleep. REM sleep accounts for 20% to 25% of sleep time. Based on EEG, EMG, and EOG characteristics, REM sleep can be subdivided into two stages: tonic and phasic. However, this subdivision is not recognized in the recently modified staging. The EEG tracings during REM sleep are characterized by fast rhythms and theta activity, some of which may have a sawtooth appearance (Fig. 68.5). A desynchronized EEG, hypotonia, or atonia of the major muscle groups and depression of monosynaptic and polysynaptic reflexes are characteristics of the tonic stage. Phasic REM sleep is characterized by rapid eye movements in all directions, as well as phasic swings in blood pressure and heart rate, irregular respiration, spontaneous middle-ear muscle activity, and tongue movements. A few periods of apnea or hypopnea may arise during REM sleep. An illustration of sleep stage distribution during the night is shown in Fig. 68.6.
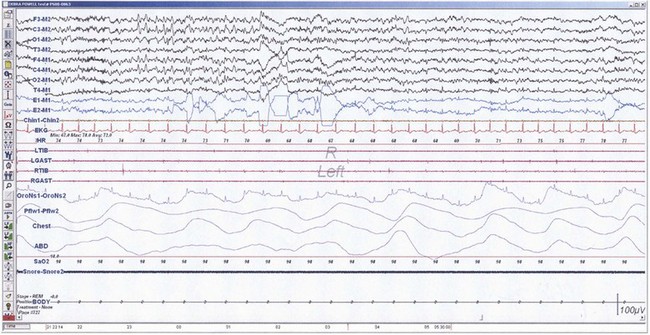
Fig. 68.5 Polysomnographic recording shows rapid eye movement (REM) sleep in an adult. Electroencephalogram (top 8 channels) shows mixed-frequency theta, low-amplitude beta, and a small amount of alpha activity. Note characteristic sawtooth waves (seen prominently in channels, 1, 2, 5, and 6 from top) of REM sleep preceding bursts of REMs in electro-oculograms (E1-M1; E2-M2). Chin electromyogram (EMG) shows marked hypotonia, whereas TIB and GAST EMG channels show very low-amplitude phasic myoclonic bursts. See Fig. 68.1 for description of the montage.
(From Chokroverty, S., 2009. Sleep Disorders Medicine: Basic Science, Technical Considerations, and Clinical Aspects, third ed. Saunders, Philadelphia, Fig. 2.6.)
Thus, there is an orderly progression from wakefulness to sleep onset to NREM sleep and then to REM sleep. A relaxed wakefulness is characterized by a behavioral state of quiescence and a physiological state of alpha and beta frequencies on the EEG recording, waking eye movements, and increased muscle tone. Table 68.2 summarizes NREM and REM sleep states. Sleep staging and scoring address normal adult sleep and the macrostructure (Box 68.1) and microstructure (Box 68.2) of sleep. In patients with sleep disorders such as sleep apnea, parasomnias, or nocturnal seizures disrupting sleep, such assessments may be difficult.
Sleep State | % Sleep Time |
---|---|
NREM sleep | 75-80 |
N1 | 3-8 |
N2 | 45-55 |
N3 | 13-23 |
REM sleep (stage R) | 20-25 |
Tonic stage | Continuous |
Phasic stage | Intermittent |
NREM, Non–rapid eye movement; REM, rapid eye movement.
Sleep Microstructure
In 1992, a Task Force of the American Sleep Disorders Association (ASDA), now the AASM, offered descriptions of sleep microstructure that included momentary dynamic phenomena such as arousals and the cyclic alternating pattern (CAP), which has been described in various publications by Terzano and co-investigators (2002). Sleep microstructure also includes K complexes and sleep spindles (see Box 68.2). According to the operational definition of the ASDA Task Force, an arousal is a shift in EEG frequency lasting for 3 to 14 seconds and includes alpha, beta, or theta activities but not spindles or delta waves. The subject must be asleep for 10 consecutive seconds before an arousal can be scored. In REM sleep, arousals are scored only when accompanied by a concurrent increase in submental EMG amplitude. Unless accompanied by EEG frequency shifts, K complexes, delta waves, artifacts, and increased submental EMG activities are not counted as arousals. An arousal index is defined as the number of arousals per hour of sleep; up to 10 can be considered a normal arousal index.
In contrast to the usual pattern of arousals signifying sleep fragmentation, the CAP indicates sleep instability. The CAP is a repetitive EEG pattern that is noted mainly during NREM sleep and lasts for 2 to 60 seconds. A phase of CAP is marked by increased EEG potentials, with contributions from both synchronous high-amplitude slow and desynchronized fast rhythms in the EEG recording. A CAP cycle consists of an unstable phase (phase A) and a relatively stable phase (phase B) (Fig. 68.7). During phase A, heart rate, respiration, blood pressure, and muscle tone increase. The rate of CAP cycles and arousals increase in both older individuals and a variety of sleep disorders. A period without CAP is thought to indicate a state of sustained stability. Evaluation of periods with and without a CAP is a promising technique for understanding normal and abnormal sleep.
Ontogeny of Sleep Patterns with Age
The newborn infant spends approximately 50% of the time in REM sleep, but by age 6 years this time is decreased to the normal adult pattern of 25% (Fig. 68.8). On falling asleep, a newborn baby goes immediately into REM sleep, or active sleep, which is accompanied by restless movements of the arms, legs, and facial muscles. In premature babies, it is often difficult to differentiate REM sleep from wakefulness. By age 3 months, the NREM-REM cyclical pattern of adult sleep is established. However, the duration of the NREM-REM cycle is shorter in infants, lasting for approximately 45 to 50 minutes and increasing to 60 to 70 minutes by age 5 to 10 years, and to the normal adult cyclical pattern of 90 to 100 minutes by age 10 years. Sleep spindles begin to appear at about 3 months of age; K complexes are seen by about 6 months. A characteristic feature of sleep in old age is marked attenuation of the amplitude of slow waves; therefore, during scoring of slow sleep, which depends not only on the rate but also on the amplitude of slow waves, the percentage of slow waves decreases. The other characteristic feature during old age is repeated awakenings throughout the night, including early-morning awakenings. The percentage of REM sleep in normal elderly individuals remains relatively constant, and the total duration of sleep time within 24 hours is not different from that of young adults, but elderly individuals often nap during the daytime, compensating for lost sleep during the night. Figs. 68.9 and 68.10 schematically show the evolution of sleep state distribution in newborns, infants, children, adults, and elder adults.
Sleep Habits
Depending on the sleep habit, two groups of individuals are recognized: evening types and morning types. Evening types (“owls”) have difficulty getting up early and feel tired in the morning; however, they feel fresh and energetic toward the end of the day. These people perform best in the evening; they go to sleep late and wake up late. In contrast, the morning types (“larks”) wake up early, rested and refreshed, and work efficiently in the morning. The body temperature rhythm shows two different curves in these two types of people. The body temperature reaches the evening peak an hour earlier in morning types than in evening types. Morning and evening types are most likely determined by genetic factors. Katzenberg and colleagues (1998), using the 19-item Horne-Östberg Questionnaire to determine “morningness/eveningness” in human circadian rhythms, discovered a CLOCK gene polymorphism associated with human diurnal preference. One of two H CLOCK alleles (3111C) is associated with eveningness. These findings were contradicted by a later study (Robilliard et al., 2002).
Sleep Requirements and Quantity of Sleep
Sleep requirement is defined as the optimal amount of sleep required to remain alert and fully awake and to function adequately throughout the day. Sleep requirement for an average adult is approximately 7.5 to 8 hours regardless of environment or cultural differences. Sleep need is determined by heredity rather than by different personality traits or other psychological factors. Social or biological factors may also play a role. An important epidemiological study from 1979 (Kripke et al.) found that the chances of death from coronary artery disease, cancer, or stroke are greater for adults who sleep less than 4 hours or more than 9 hours a night when compared to those who sleep an average of 8 hours. Some more recent studies by Kripke and colleagues (2002) and others have confirmed these observations. In later studies, other confounding factors such as sleep medications may have confounded these issues. There is no clear-cut conclusion yet. There is also some controversy over whether a person can extend sleep beyond the average requirement. In 1969, Taub and Berger study showed that sleep extension beyond the average may cause exhaustion and irritability, with detriment of sleep efficiency. The overall conclusion, however, is that sleep extension has minimal effect on sleepiness and performance in absence of sleep debt, which is defined as the difference between the ideal sleep requirement and the actual duration of sleep obtained. Modern society appears to be chronically sleep deprived. Data demonstrate detrimental loss of vigilance with progressive sleep loss, confirming the possibility that sleep deprivation’s consequences are serious. Fig. 68.11 shows psychomotor vigilance task (PVT) performance lapses under varying doses of daily sleep, confirming the data of serious lapses when sleep is curtailed beyond 8 hours. A 1979 study examining 8- to 17-year-old adolescents between the years 1910 and 1963 showed a mean reduction of 1.5 hours of sleep in that time. There may, however, be a significant sampling error in this survey. A 1992 study by Bliwise and colleagues in healthy adults aged 50 to 65 showed a reduction of about 1 hour of sleep between 1959 and 1980 surveys.
Neurobiology of Sleep and Wakefulness
The neuroanatomical substrates for wakefulness, REM sleep, and NREM sleep are located in separate parts of the CNS (McCarley, 2009; Steriade and McCarley, 2005). There are no discreet sleep-promoting centers; sleep/wake states are produced by changes in interconnected systems rather than by discreet centers.
Neuroanatomical Substrates of Wakefulness
Wakefulness is controlled by the ascending reticular activating system (ARAS) containing glutamatergic, cholinergic, aminergic, and hypocretinergic neurons (Chokroverty, 2003; Steriade and McCarley, 2005). Projections from the ARAS terminating in the thalamus and thalamocortical projections to widespread areas of the cerebral cortex produce cerebral cortical activation during wakefulness. Extrathalamic projections from the brainstem reticular neurons terminate in the posterior hypothalamus and the basal forebrain regions; the latter project to the cerebral cortex (basocortical projections) to maintain wakefulness (Fig. 68.12). In the 1930s, Bremer discovered evidence of an ascending arousal system necessary for cortical arousal when he demonstrated that transection of the brainstem at the midcollicular level (i.e., cerveau isolé), but not the spinomedullary junction (i.e., encéphale isolé), produced coma in anesthetized cats. Bremer hypothesized that the resulting reduction in “cerebral tone” following the cerveau isolé was due to interruption of ascending sensory inputs—that is, a passive “deafferentation theory” of sleep (Bremer, 1937).
All these pathways regulating the wakefulness system use the cholinergic, noradrenergic, dopaminergic, and histaminergic neurons (Fig. 68.13). The cholinergic neurons fire at the highest rate during wakefulness and REM sleep but decrease their rates of firing at the onset of NREM sleep. Wakefulness-promoting aminergic neurons include noradrenergic neurons in the locus ceruleus, serotonergic neurons in the dorsal raphe of the brainstem, histaminergic neurons in the tuberomammillary nucleus of the hypothalamus, and possibly also dopaminergic neurons in the ventral tegmental area, substantia nigra, and ventral periaqueductal area. After a period of uncertainty regarding the role of dopamine, it has recently been confirmed that the midbrain dopaminergic system (A8-A10), particularly the dopaminergic neurons in the ventral periaqueductal gray (vPAG), play an active role in maintaining wakefulness through their widespread reciprocal connections with sleep/wake regulatory systems of neurons (Fuller and Lu, 2009). Norepinephrine-containing locus ceruleus neurons show their highest firing rates during wakefulness, their lowest during REM sleep, and intermediate rates during NREM sleep. Pharmacological studies suggest that posterior hypothalamic histaminergic neurons also help maintain wakefulness.
The excitatory amino acids, glutamate and aspartate, are intermingled within the ARAS and are present in many neurons projecting to the cerebral cortex, forebrain, and brainstem. These excitatory amino acids are maximally released during wakefulness. Recent discovery of hypothalamic hypocretin neurons and their widespread CNS projections have directed attention to the role of the hypocretin system in sleep/wake regulation. In 1998, deLecea and coauthors described two neuropeptides in the lateral hypothalamus and perifornical region that were termed hypocretin 1 and hypocretin 2. Independently in the same year, Sakurai and colleagues (1998) described two neuropeptides in the same region, which they named orexin A and orexin B (corresponding to hypocretin 1 and hypocretin 2, respectively). It was shown thereafter that these hypocretin systems have widespread ascending and descending projections to the locus ceruleus, dorsal raphe, ventral tegmental area, tuberomammillary nuclei of the posterior hypothalamus, laterodorsal tegmental (LDT) and pedunculopontine tegmental (PPT) nuclei, ventrolateral preoptic (VLPO) neurons in the hypothalamus, basal forebrain, limbic system (hippocampus and amygdala), cerebral cortex, thalamus (intralaminar and midline nuclei), and autonomic neurons (nucleus tractus solitarius [NTS], dorsal vagal nuclei, and intermediolateral neurons of the spinal cord). Hypocretin systems promote wakefulness mainly through excitation of tuberomammillary histaminergic, locus ceruleus noradrenergic, and midline raphe serotonergic neurons as well as dopaminergic neurons. Reduced activity of hypocretin systems may be partly responsible for inducing sleepiness. These systems also suppress REM sleep through activation of the aminergic neurons (REM-off), which in turn inhibit REM-on neurons in the LDT/PPT nuclei. As shown in Fig. 68-13, brainstem arousal centers were identified and characterized, and support was later provided for the concept of sleep-promoting circuitry in the anterior hypothalamus/preoptic area. It was later in the mid-1990s that the identity of this sleep-promoting circuitry was revealed. In these investigations (see Fig. 68.13, B), it was demonstrated that the VLPO nucleus contains sleep-active cells that contain the inhibitory neurotransmitters, γ-aminobutyric acid (GABA) and galanin (Gal).
Neuroanatomical Substrates for REM Sleep
The existence of REM sleep–generating neurons in the pontine cat has been established by transection experiments (see Fig. 68.12) through different regions of the midbrain, pons, and medulla (McCarley, 2009). A transection at the junction of the pons and midbrain produced all the physiological findings compatible with REM sleep in the section caudal to that transection, whereas in the forebrain region rostral to the section, the recording showed no signs of REM sleep. After transection between pons and medulla, structures rostral to the section showed signs of REM sleep, but structures caudal to the section had no signs of REM sleep. After transection at the junction of the spinal cord and medulla, REM sleep signs were noted in the rostral brain areas. Finally, transection at the pontomesencephalic and pontomedullary junctions produced an isolated pons that showed all the signs of REM sleep. The pons is therefore sufficient and necessary to generate all the signs of REM sleep.
There are two main models to explain the mechanism of REM sleep. The earliest and most generally well known is the McCarley-Hobson reciprocal interaction model (Fig. 68.14) based on the reciprocal interaction of REM-on and REM-off neurons (McCarley, 2009). Neurons in the PPT and LDT nuclei in the pontomesencephalic region are cholinergic and are REM-on cells, which are responsible for REM sleep, showing highest firing rates at this stage. The REM-off cells are located in the locus ceruleus and dorsal raphe nuclei. These cells are aminergic neurons and are inactive during REM sleep. Histaminergic neurons in the tuberomammillary region of the posterior hypothalamus can also be considered REM-off cells. Thus, the cholinergic REM-on and aminergic REM-off cells are all located within the transections in the pons. LDT-PPT cholinergic neurons promote REM sleep through pontine reticular formation (PRF) effector neurons, which in turn send feedback loops to LDT-PPT neurons. Cholinergic neurons of the PPT and LDT projecting to the thalamus and basal forebrain regions, as well as to the PRF, are responsible for activation and generation of REM sleep. Aminergic cells seem to play a permissive role in the appearance of REM sleep state. In the latest modification of the reciprocal interaction model, McCarley suggested that in addition to cholinergic excitation of PRF, a reduction of GABA inhibition in the PRF also may play a role in REM sleep generation. For example, GABA levels in the PRF (as measured by microdialysis technique) are lowest during REM and intermediate between wakefulness and REM and NREM sleep. Also, injection of GABA antagonists (e.g., bicuculline) into rostral PRF produced REM sleep in cats and rats. There is evidence of GABA-ergic inhibition of locus ceruleus/dorsal raphe nuclei (REM-off neurons). The source of GABA-ergic neurons is probably both local (e.g., a subgroup of PRF GABA-ergic neurons) and distant (e.g., GABA-ergic neurons in the ventrolateral periaqueductal gray matter).
In the model (Fig. 68.15) proposed by Lu and coworkers (2006), there is reciprocal interaction between GABA-ergic REM-off neurons in ventrolateral periaqueductal gray matter (vlPAG) and lateral pontine tegmentum and GABA-ergic REM-on neurons in the sublaterodorsal nucleus (SLD; corresponding to the dorsal subceruleus or peri–locus ceruleus alpha in cats) and a dorsal extension of the SLD termed the preceruleus (PC). These mutually inhibitory neuronal populations (SLD GABA-ergic REM-on and GABA-ergic REM-off neurons in the vlPAG- lateral pontine tegmentum) serve as a flip-flop switch. Ascending glutamatergic projections from PC neurons to medial septum are responsible for EEG hippocampal theta rhythm during REM sleep. Descending glutamatergic projections from ventral SLD directly to spinal interneurons, apparently without a relay in the medial medulla, inhibit spinal ventral horns by both glycerinergic and GABA-ergic mechanisms. Cholinergic and aminergic neurons play a modulatory role and are not part of the flip-flop switch. The question arises as to how two mutually inhibitory neuronal populations (e.g., GABA-ergic REM-on and GABA-ergic REM-off neurons) stabilize the flip-flop switch permitting NREM-REM cycling. It has been suggested that inhibitory external projections from extended ventrolateral preoptic neurons (eVLPO) and excitatory projections from hypocretinergic and aminergic neurons to the REM-off neurons stabilize the switch, permitting NREM-REM cycling. McCarley (2009) suggested that this model is based on C-fos labeling, only without electrophysiological recordings. Furthermore, this model does not address how REM sleep duration progressively increases with the progression of the night. A third promising model has recently been proposed by Luppi et al. (2011).
Neuroanatomical Substrates of NREM Sleep
The contemporary theory for the mechanism of NREM sleep suggests a reciprocal interaction between two antagonistic neurons in the VLPO area of the anterior hypothalamus and wake-promoting neurons in the tuberomammillary nuclei of the posterior hypothalamus, basal forebrain, and mesopontine tegmentum (McCarley, 2009; Steriade and McCarley, 2005). Reciprocal interaction between sleep-promoting neurons in the region of the NTS and wake-promoting neurons within the ARAS of the brainstem independent of the reciprocal interaction of the neurons of the forebrain also plays a role in the generation of NREM sleep. The latter is the old reticular passive hypothesis, or the disfacilitation hypothesis, of sleep. In this model, sleep is said to result from a cascade of disfacilitation within the brainstem. The passive theory originated in the two classic preparations in cats by Bremer (1937), noted earlier in the chapter: cerveau isolé and encéphale isolé. Bremer found that in cerveau isolé (e.g., midcollicular transection), all specific sensory stimuli were withdrawn and the animals were somnolent, whereas in encéphale isolé (transection at C1 vertebral level disconnecting the entire brain from the spinal cord), these specific stimuli maintained activation of the brain and animals were awake. Moruzzi and Magoun postulated that withdrawal of generalized activation from ARAS is responsible for somnolence in cerveau isolé preparations, whereas activation of the midbrain reticular neurons causing direct excitation of the thalamocortical projections results in EEG desynchronizations and behavioral arousal. These observations support the later suggestion of Steriade and colleagues that at the onset of NREM sleep, there is a deafferentation of the brain due to blockage of afferent information, first at the thalamic level, causing the waking “open” brain to be converted to a “closed” brain owing to thalamocortical inhibition. This passive reticular theory, however, was challenged by the experiments of Batini and colleagues in 1959, producing the midpontine pretrigeminal section, which was only a few millimeters below the section that produced cerveau isolé. In this midpontine preparation, there were persistent EEG and behavioral signs of alertness, suggesting that structures located in the brainstem between cerveau isolé and midpontine pretrigeminal preparations are responsible for wakefulness. Thus an active inhibitory role of the lower brainstem hypnogenic neurons in the region of the NTS on the upper brainstem ARAS was clearly demonstrated by this preparation. Both active and passive theories partly explain the generation of NREM sleep, but the contemporary theory favors the activation of VLPO neurons and inhibition of posterior hypothalamic neurons containing histamine and hypocretin. Reduced activity of the hypocretin projections to the locus ceruleus noradrenergic, midline raphe serotonergic, mesopontine dopaminergic, and tuberomammillary hypothalamic histaminergic cells may also decrease the level of arousal, causing sleepiness.
The suprachiasmatic nuclei (SCN), the paired nuclei above the hypothalamus, function as the body clock to control circadian rhythm. The recent discovery of anatomical projections from SCN to lateral hypothalamic neurons containing hypocretin (wake-promoting) and anterior hypothalamic VLPO containing sleep-promoting neurons suggested that the SCN may also affect sleep regulation and homeostasis independent of circadian rhythm generation (Turek and Vitaterna, 2011). Finally, recent advances in neuroimaging studies, including positron emission tomography (PET) and single-photon emission computed tomography (SPECT) scans, have been able to visualize dramatic changes in function in cortical and subcortical neuronal networks in different sleep states and stages, advancing our understanding of the functional neuroanatomy of sleep/wakefulness (Dang-Vu et al., 2007). PET scans have shown marked activation of the amygdala and the anterior cingulate region (part of the limbic system) during REM sleep, which is, of course, generated by brainstem neurons. In contrast, in NREM sleep, neuroimaging techniques have shown declining function in the thalamocortical circuits, including the association cortex of the frontoparietal and temporal lobes. Thus the brainstem, hypothalamic, and forebrain sleep/wake-promoting neurons modulate functions of widespread forebrain cortical areas, keeping in balance cortical and subcortical circuits to control sleep/wake regulation.
Circadian Rhythm and Chronobiology of Sleep
The 18th-century, French astronomer de Mairan first directed our attention to the existence of circadian rhythm when he noted that in a heliotrope plant, the leaves closed at sunset and opened at sunrise even when the plant was kept in darkness (see Chokroverty, 2009a). This observation clearly pointed to a 24-hour rhythm controlled by an internal clock. The existence of such a circadian rhythm in human beings and other animals was confirmed toward the last half of the last century. The term circadian rhythm originates from the Latin circa, meaning “about,” and dies, meaning “day.” Human circadian rhythm generally has a cycle length close to 24 hours (approximately 24.2 hours) (Czeisler and Gooley, 2007). The existence of circadian rhythms independent of environmental stimuli has been clearly demonstrated by experimental isolation of humans from all environmental time cues (the German term, Zeitgeber, or “time giver”), as in a cave or underground bunker, to study free-running rhythms. The existence of environment-independent autonomous rhythms suggests that the human body also has an internal biological clock. The paired SCN of the hypothalamus above the optic chiasma are the sites of the biological clock. The master circadian clock in the SCN receives photic information from the retinohypothalamic tract, which sends signals to multiple synaptic pathways in other parts of the hypothalamus, the superior cervical ganglion, and the pineal gland where melatonin is released. The SCN contain melatonin receptors, and there is a feedback loop from the pineal gland to the SCN. Time isolation experiments have clearly shown the presence of daily rhythms in many physiological processes, such as the sleep/wake cycle, body temperature, and neuroendocrine secretion. Melatonin is secreted maximally during the night and is an important modulator of human circadian rhythm for entrainment by the light/dark cycle. The melatonin level rises fairly abruptly in the evening and then reaches its maximum level between 3:00 am and 5:00 am, after which it decreases to low levels during the daytime.
Sleep scientists have begun to identify the molecular basis of the mammalian circadian clock. A total of eight or nine genes (e.g., CLOCK, PER, Bmal) and their protein products have been identified within the circadian clock system; understanding of these is still evolving (Turek and Vitaterna, 2011). Remarkable progress has been made in the past few years in the key components of the circadian clock in both fruitflies (Drosophila) and mammals. Dysfunction of circadian rhythm results in some important human sleep disorders. The molecular mechanism of two human circadian rhythm disorders—advanced sleep phase syndrome (ASPS) and delayed sleep phase syndrome (DSPS)—has been uncovered by applying gene sequencing techniques. ASPS seems to be due to a mutation of the PER2 gene (a human homolog of the period 2 gene in Drosophila) causing advancing of the clock (i.e., alteration of the circadian timing of sleep propensity). DSPS is due to polymorphism of the PER3 gene.
Circadian, Homeostatic, and Other Sleep Factors
Sleep and wakefulness are controlled by both homeostatic and circadian factors. The duration of prior wakefulness determines the propensity to sleepiness (homeostatic factor), whereas circadian factors determine the timing, duration, and characteristics of sleep. There are two types of sleepiness: physiological and subjective (Dinges, 1995). Physiological sleepiness is the body’s propensity to sleepiness. There are two highly vulnerable periods of sleepiness: 2:00 to 6:00 am, particularly 3:00 to 5:00 am, and 2:00 to 6:00 pm, particularly 3:00 to 5:00 pm. The propensity to physiological sleepiness (e.g., midafternoon and early-morning hours) depends on circadian factors. The highest number of sleep-related accidents have been observed during these periods. Subjective sleepiness is the individual’s perception of sleepiness; it depends on several external factors such as a stimulating environment and ingestion of coffee and other caffeinated beverages.
Physiological sleepiness depends on two processes: homeostatic factor and circadian phase. Homeostatic factor refers to a prior period of wakefulness and sleep debt. After a prolonged period of wakefulness, there is an increasing tendency to sleep. The recovery from sleep debt is aided by an additional amount of sleep, but this recovery is not linear. Thus, an exact number of hours of sleep are not required to repay sleep debt; rather, the body needs an adequate amount of slow-wave sleep for restoration. The interaction between the circadian and the homeostatic drive producing alertness is depicted in Fig. 68.16. The circadian factor determines the body’s propensity to maximal sleepiness between 3:00 am and 5:00 am. The second period of maximal sleepiness (3:00 to 5:00 pm) is not as strong as the first. Sleep and wakefulness and the circadian pacemaker have a reciprocal relationship: the biological clock can affect sleep and wakefulness, and sleep and wakefulness can affect the clock. There are two variables that seem to play a role in regulating the timing of sleep. First is the homeostatic sleep drive, which increases as the day progresses and the longer a person is awake. The second is timing information from the suprachiasmatic nucleus (SCN). In this two-process model, the SCN promotes wakefulness by stimulating arousal networks. The activity of the circadian system appears to oppose that of the homeostatic sleep drive, and thus the alerting mediated by the SCN increases during the day. The propensity to be awake or asleep at any time is related to the homeostatic sleep drive and the opposing SCN alerting signal. At normal bedtime, both the alerting drive and the sleep drive are at their highest level. The SCN has at least two types of melatonin receptors, MT1 and MT2, involved in the regulation of sleep. Stimulation of MT1 receptors is believed to decrease the alerting signal from the SCN, while MT2 stimulation is thought to be involved in synchronizing the circadian system.
Various sleep factors have been identified, but their role in maintaining homeostasis has not been clearly established (Krueger et al., 2011). Several cytokines, such as interleukin-1, interferon-α, and tumor necrosis factor, promote sleep. Other sleep factors increase in concentration during prolonged wakefulness and infection. It has been shown that adenosine in the basal forebrain can fulfill the major criteria for the neural sleep factor that mediates the somnogenic effect of prolonged wakefulness by acting through adenosine A1 and A2A receptors. Several other endogenous compounds may serve as sleep factors, including delta sleep-inducing peptides, muramyl peptides, cholecystokinins, arginine vasotocin, vasoactive intestinal peptides, growth hormone–releasing factors, and somatostatins.
Functions of Sleep
The function of sleep remains the greatest biological mystery of all time. There are several theories about the function of sleep (Box 68.3), but none are satisfactory (Crick and Mitchison, 1995; Kavanau, 1997; Mahowald et al., 1997). Sleep deprivation experiments in animals have clearly shown that sleep is necessary for survival, but from a practical point of view, complete sleep deprivation for a prolonged period cannot be conducted in humans. Sleep deprivation studies in humans have shown an impairment of performance, which demonstrates the need for sleep. The performance impairment of prolonged sleep deprivation results from a decreased motivation and frequent “microsleeps.” Overall, human sleep deprivation experiments have proven that sleep deprivation causes sleepiness and impairment of performance, vigilance, attention, concentration, and memory. Sleep deprivation may also cause some metabolic, hormonal, and immunological effects. Sleep deprivation causes immune suppression; even partial sleep deprivation reduces cellular immune responses. Studies from Van Cauter’s (Spiegel et al., 1999