CHAPTER 19 Single-Gene Disorders
To date, more than 10,000 single-gene traits and disorders have been identified. Most of these are individually rare, but together they affect between 1% and 2% of the general population at any one time. The management of these disorders in affected individuals and in their extended families presents the major workload challenge in clinical genetics.
Huntington Disease
Genetics
Mapping and Isolation of the HD Gene
HD was one of the first disorders to be mapped by linkage analysis using polymorphic DNA markers when, in 1983, the disorder was found to show close linkage with a probe known as G8 on the short arm of chromosome 4, greatly aided by collection of blood samples from the huge pedigree containing over 100 affected subjects living on the shores of Lake Maracaibo in Venezuela. As well as providing the first means of predictive testing for HD, this work also revealed that HD homozygotes are no more severely affected than heterozygotes. This is in contrast to many other autosomal dominant disorders (p. 109). When the gene itself was isolated in 1993, it was found to contain a highly polymorphic CAG (polyglutamine) repeat sequence located in the 5′ region. The messenger RNA (mRNA) codes for a protein of approximately 350 kDa, known as huntingtin (also IT15). Huntingtin is expressed in many different cells throughout the central nervous system, as well as other tissues, although its function remains unclear.
The Mutation in HD
Almost all individuals with HD possess an expansion of a CAG polyglutamine (triplet) repeat sequence located in the 5′ region of the HD gene, a mutational mechanism first identified in humans in contrast to almost all other types of mutation that were first reported in other species such as Drosophila and mice. A joint working party of the American College of Medical Genetics and the American Society of Human Genetics recommended that HD genes should be categorized under four headings on the basis of CAG repeat length (Table 19.1).
Table 19.1 Comparison of Genetic Aspects of Huntington Disease and Myotonic Dystrophy
Huntington Disease | Myotonic Dystrophy | |
---|---|---|
Inheritance | Autosomal dominant | Autosomal dominant |
Chromosome locus | 4p16.3 | 19q13.3 |
Trinucleotide repeat | CAG in 5′ translated region | CTG in 3′ untranslated region |
Repeat sizes | Normal ≤26 | Normal <37 |
Mutable 27–35 | ||
Reduced penetrance | Full mutation | |
36–39 | 50–2000+ | |
Fully penetrant ≥40 | ||
Protein product | Huntingtin | MD protein kinase (DMPK) |
Early-onset form | Juvenile | Congenital |
Usually paternally transmitted | Usually maternally transmitted |
Parent of Origin Effect in Disease Transmission
Explanations for this include the possibility that expansion is caused by slippage (p. 24) of DNA polymerase, simply reflecting the number of mitoses undergone during gametogenesis (p. 41). An alternative possibility is based on the observation that huntingtin is expressed in oocytes, so that there could be selection against oocytes with large expansions as a consequence of preferential apoptosis.
Clinical Applications and Future Prospects
Prenatal diagnosis is possible for those couples who find this acceptable, although only about 25 such tests are performed in the United Kingdom annually. Obviously there are considerable emotional and ethical issues associated with termination of pregnancy; the condition is late in onset and the couple must consider the possibility of effective therapy being available in the foreseeable future. One appealing therapeutic approach is based on the observation that large CAG repeats result in intracellular accumulation of huntingtin ‘aggregates’, which are cleaved by a protease known as caspase to form a toxic product that causes cell death (apoptosis). Caspase inhibitors have been shown to have a beneficial effect in a HD mouse model. Another therapeutic approach under consideration is fetal neuronal cell transfer into regions of the brain, such as the caudate nucleus and putamen, which become atrophic in the early stages of the disease. This approach carries ethical considerations that will be difficult for some couples.
Myotonic Dystrophy
Myotonic dystrophy (MD) is the most common form of muscular dystrophy seen in adults, with an overall incidence of approximately 1 : 8000. It shares many features in common with HD (see Table 19.1)—both show autosomal dominant inheritance with anticipation, and an early-onset form with different clinical features. However, in MD the early-onset form is transmitted almost exclusively by the mother and presents at birth, in contrast to juvenile HD, which is generally paternally transmitted with an age of onset in the teens.
Clinical Features
In contrast to most forms of muscular dystrophy, clinical features in MD are not limited exclusively to the neuromuscular system. Individuals with MD usually present in adult life with slowly progressive weakness and myotonia. This latter term refers to tonic muscle spasm with prolonged relaxation, which can manifest as a delay in releasing the grip on shaking hands. Other clinical features include cataracts (Figure 19.1), cardiac conduction defects, disturbed gastrointestinal peristalsis (dysphagia, constipation, diarrhea), weak sphincters, increased risk of diabetes mellitus and gallstones, somnolence, frontal balding, and testicular atrophy. The age of onset is very variable and in its mildest form usually runs a relatively benign course. However, as the age of onset becomes earlier, so the clinical symptoms increase in severity and more body systems are involved. In the ‘congenital’ form, affected babies present at birth with hypotonia, talipes, and respiratory distress that can prove life threatening (see Figure 7.19). Children who survive tend to show a lack of facial expression (‘myopathic facies’) with delayed motor development and learning difficulties (Figure 19.2).
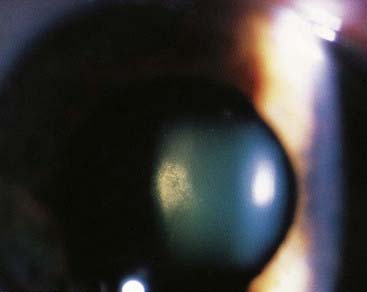
FIGURE 19.1 Refractile lens opacities in an asymptomatic person with myotonic dystrophy.
(Courtesy Mr. R. Doran and Mr. M. Geall, Department of Ophthalmology, General Infirmary, Leeds, UK.)
Genetics
It follows autosomal dominant inheritance with increasing severity in succeeding generations—anticipation (p. 120). It used to be thought that this reflected ascertainment bias, caused by the greater likelihood of detecting a mildly affected parent with a severely affected child, rather than the other way round. However, studies in the 1980s confirmed that anticipation is a real phenomenon.
Genotype–Phenotype Correlation in Myotonic Dystrophy
In unaffected persons the CTG sequence lying 3′ to the DMPK gene consists of up to 37 repeats (see Table 19.1). Affected individuals have an expansion of at least 50 copies of the CTG sequence. There is a close correlation between disease severity and the size of the expansion, which can exceed 2000 repeats. The severe congenital cases show the largest repeat copy number, with almost invariable inheritance from the mother. Thus, meiotic or germline instability is greater in the female for alleles containing large sequences. Curiously, expansion of a relatively small number of repeats appears to occur more commonly in the male, and most MD mutations are thought to have occurred originally during meiosis in the male. One possible explanation for these observations is that mature spermatozoa can carry only small expansions, whereas ova can accommodate much larger expansions.
Another puzzling feature of MD is the reported tendency for healthy individuals who are heterozygous for MD alleles in the normal size range to preferentially transmit alleles greater than 19 CTG repeats in size. This possible example of meiotic drive (p. 135) could explain the relatively high frequency of MD with constant replenishment of a reservoir of potential MD mutations.
Clinical Applications and Future Prospects
Presymptomatic genetic testing and prenatal diagnosis can be offered to those families for whom it is appropriate and acceptable. This is particularly relevant for couples who have had a child with the severe congenital form, for whom the risk of recurrence is relatively high. As in HD, presymptomatic testing should not be undertaken without an offer of long-term support and medical care, and a discussion about possible difficulty in obtaining life and health insurance (p. 366).
Hereditary Motor and Sensory Neuropathy
Clinical Features
In autosomal dominant HMSN-I—the most common form—there is onset of slowly progressive distal muscle weakness and wasting in the lower limbs between the ages of 10 and 30 years, followed later by the upper limbs in many patients, and associated ataxia and tremor. The appearance of the lower limbs has been likened to that of an ‘inverted champagne bottle’ (Figure 19.3). With age, locomotion becomes more difficult and the feet tend to show exaggeration of their normal arch, known as ‘pes cavus’. Despite these quite striking changes, many patients retain reasonable muscle strength and are not too seriously disabled. Other faculties such as vision, hearing, and intellect are not impaired. Palpable thickening of peripheral nerves can sometimes be detected.
Genetics
HMSN can show autosomal dominant, autosomal recessive or X-linked inheritance, although autosomal dominant forms are by far the most common. More than 70% of cases of HMSN-I are due to a DNA duplication of 1.5 Mb chromosome 17p that encompasses the peripheral myelin protein-22 (PMP22) gene. The glycoprotein product is present in the myelin membranes of peripheral nerves, where it helps to arrest Schwann cell division. HMSN-I in humans is therefore thought to be the result of a PMP22 dosage effect, though point mutations can be found some patients. The duplication is generated by misalignment and subsequent recombination between homologous sequences that flank the PMP22 gene (Figure 19.4); this event usually occurs in male gametogenesis (rather than in the female, which is the case in Duchenne muscular dystrophy [p. 307]). The reciprocal deletion product of this misaligned recombination event, giving rise to haploinsufficiency, causes a relatively mild disorder known as hereditary neuropathy with liability to pressure palsies. Minor nerve trauma, such as pressure from prolonged sitting on a long-haul flight, causes focal numbness and weakness. The same misalignment recombination mechanism occurs in Hb Lepore and anti-Lepore (see Figure 10.3; p. 157), congenital adrenal hyperplasia (p. 174), and deletion 22q11 syndrome (p. 282), to name but a few.
Other Forms of Hereditary Motor and Sensory Neuropathy
A more rare form of HMSN shows X-linked dominant inheritance, with males having typical HMSN-I features and females being more mildly affected—sometimes with HMSN-II characteristics. This is due to mutations in the gene that encodes a gap junction protein called GJB1 (previously Connexin 32).
Neurofibromatosis
There are two main types of neurofibromatosis, NF1 and NF2. Both conditions, especially NF2, could be included under familial cancer syndromes (see Chapter 14) but are covered in more detail here. NF1 has a birth incidence of approximately 1 : 3000; NF2 approximately 1 : 35,000 and a prevalence of around 1 : 200,000.
Clinical Features
The most notable features of NF1 are small pigmented skin lesions, known as café-au-lait (CAL) spots, and small soft fleshy growths known as neurofibromata (Figure 19.5). CAL spots first appear in early childhood and continue to increase in both size and number until puberty. A minimum of six CAL spots at least 5 mm in diameter is required to support the diagnosis in childhood, and axillary and/or inguinal freckling should be present. Neurofibromata are benign tumors that arise most commonly in the skin, usually appearing in adolescence or adult life, and increasing in number with age.
Other clinical findings include relative macrocephaly (large head) and Lisch nodules. These are small harmless raised pigmented hamartomata of the iris (Figure 19.6). The most common complication, occurring in a third of childhood cases, is mild developmental delay characterized by a non-verbal learning disorder. For many, significant improvement is seen through the school years. Most individuals with NF1 enjoy a normal life and are not unduly inconvenienced by their condition. However, a small number of patients develop one or more major complications, such as epilepsy, a central nervous system tumor, or scoliosis.
Genetics
NF1 shows autosomal dominant inheritance with virtually 100% penetrance by the age of 5 years. The effects are very variable and affected members of the same family can show striking differences in disease severity. The features in affected MZ twins are usually very similar, so the variability in family members with the same mutation may be due to modifying genes at other loci. Approximately 50% of cases of NF1 are due to new mutations, with the estimated mutation rate being approximately 1 per 10,000 gametes. This is around 100 times greater than the average mutation rate per generation per locus in humans.
There are a few reports of more than one affected child born to unaffected parents—the result of gonadal mosaicism (p. 121), usually paternal in origin. Somatic mosaicism in NF1 can manifest with features limited to a particular part of the body. This is referred to as segmental NF.
The Neurofibromatosis Type 1 Gene and its Product
The NF1 gene, neurofibromin, was successfully mapped to chromosome 17, adjacent to the centromere, in 1987. Its isolation was aided by the identification of two patients who both had a balanced translocation with a breakpoint at 17q11.2. A cosmid clone was identified containing both translocation breakpoints and a search for transcripts from this region yielded four genes, one of which was shown to be neurofibromin. It is large, spanning over 350 kilobases (kb) of genomic DNA and comprising at least 59 exons. The other three genes identified in this region were found to lie within a single intron of the neurofibromin gene, where they are transcribed in the opposite direction from the complementary strand (p. 14).
The neurofibromin protein encoded by this gene shows structural homology to the guanosine triphosphatase (GTPase)-activating protein (GAP), which is important in signal transduction (p. 214) by downregulating RAS activity. The place of neurofibromin in the RAS-MAPK pathway is shown in Figure 16.12, highlighting the link with Noonan syndrome (p. 254). Loss of heterozygosity (p. 215) for chromosome 17 markers has been observed in several malignant tumors in patients with NF1, as well as in a small number of benign neurofibromata. These observations indicate that the neurofibromin gene functions as a tumor suppressor (p. 214). It has been shown to contain a GAP-related domain (GRD), which interacts with the RAS proto-oncogene product. A mRNA editing site exists in the neurofibromin gene and edited transcript causes GRD protein truncation, which inactivates the tumor suppressor function. A higher range of editing is seen in more malignant tumors.
Other genes, including TP53 (p. 218) on the short arm of chromosome 17, are also involved in tumor development and progression in NF1. Conversely, it is also known that the neurofibromin gene is implicated in the development of sporadic tumors not associated with NF, including carcinoma of the colon, neuroblastoma, and malignant melanoma. These observations confirm that the neurofibromin gene plays an important role in cell growth and differentiation.