Respiratory system
Respiration is the totality of those processes that ultimately result in energy being supplied to the cells. In pregnancy the respiratory system undergoes significant changes. The increased metabolic needs of the pregnant woman, fetus, and placenta require improved maternal respiratory efficiency to ensure adequate oxygenation. The placenta functions as the respiratory system for the fetus, providing nutrients and oxygen and removing carbon dioxide. This makes the relationship between the mother and fetus not only intimate but also interdependent. At birth, tremendous energy is expended by the neonate to generate sufficient negative pressure to convert to an air-liquid interface in the alveoli and maintain functional residual capacity (FRC). Transitional events continue over the first week of life as the neonate adjusts to the new environment, more alveoli are recruited, and the surfactant system stabilizes. This chapter examines alterations in the respiratory system and acid-base homeostasis during the perinatal period and their implications for the mother, fetus, and neonate. Fetal blood gases and responses to hypoxia are discussed in Chapter 6.
Maternal physiologic adaptations
Antepartum period
Pregnancy is associated with major changes in the respiratory system, including changes in lung volumes and ventilation. Both biochemical and mechanical factors interact to increase the delivery of oxygen and the removal of carbon dioxide.147 Otolaryngeal changes are discussed in Chapter 15.
Factors influencing respiratory function
Mechanical factors.
The gradual enlargement of the uterus leads to changes in abdominal size and shape, shifting the resting position of the diaphragm up to 4 cm above its usual position to accommodate the growing uterus (Figure 10-1).23,43,96,147,247 The thoracic circumference increases by 5 to 7 cm and the transverse diameter of the chest by about 2 cm in response to the increased intraabdominal pressure with a flaring of the lower ribs.43,96,247 The subcostal angle progressively increases from 68 to 103 degrees in late gestation.23,96,247 Not all of these changes can be attributed to intraabdominal pressure, in that the increase in the subcostal angle occurs before the increasing mechanical pressure.147,247,250 Relaxation of the ligamentous rib attachments increases rib cage elasticity. This change is similar to those seen in the pelvis and mediated by similar factors, such as relaxin (see Chapter 15).
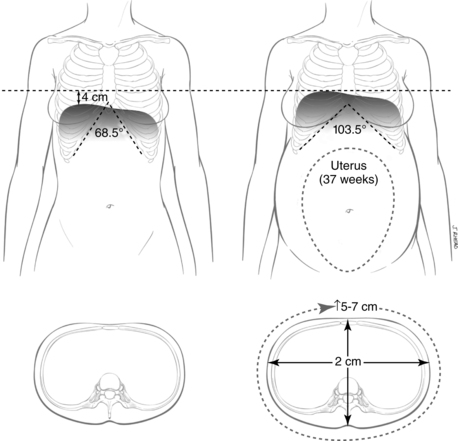
Although the aforementioned changes suggest that diaphragmatic motion decreases, the reverse is actually true. Diaphragmatic movement actually increases about 2 cm during pregnancy, with the major work of breathing being accomplished by the diaphragm rather than by the costal muscles.23,43,96,147 These changes in thoracic structures modify the abdominal space, perhaps in preparation for the increase in uterine size and peak at 37 weeks.96 The changes in thoracic configuration have a major impact on lung volumes, however, to which the increasing intra-abdominal pressure contributes.
Biochemical factors.
Hormones and other biochemical factors are important in stimulating changes in the respiratory system in pregnancy. These substances can act centrally via stimulation of the respiratory center or directly on smooth muscle and other tissues of the lung. The most important influences are mediated by progesterone, in combination with estradiol (which increases progesterone receptors in the hypothalamus) and prostaglandins (PGs).108
Serum progesterone levels increase progressively throughout pregnancy and are thought to be a major factor in the changes seen in ventilation.250 Progesterone is a respiratory stimulant. The administration of progesterone to nonpregnant subjects increases minute ventilation and enhances responses to hypercapnia.96,108,147 This suggests an increased sensitivity to carbon dioxide by the respiratory center and that progesterone lowers the carbon dioxide threshold of the respiratory center.3,108,250 For example, in the nonpregnant woman, an increase of 1 mmHg (0.13 kPa) in the PaCO2 results in a 1.5 L/min increase in ventilation, whereas during pregnancy the same 1-mmHg increase in PaCO2 leads to a 6-L/min increase in ventilation.247 More than 60% of the increase in CO2 sensitivity occurs by 20 weeks’ gestation.213
This increased sensitivity most likely contributes to the sensation of dyspnea that is experienced by many pregnant women and may also lead to some of the hyperventilation that occurs during the second stage of labor after pushing efforts (as carbon dioxide levels increase during breath holding).147 Progesterone may also exert a local effect on the lung, causing water retention in the lung that results in decreased diffusion capacity. Therefore hyperventilation is an attempt to maintain normal PO2 levels.247
Lung volume
Changes in lung volumes begin in the middle of the second trimester and are progressive to term (Figure 10-2). The most significant change is a 30% to 40% (from 500 to 700 mL) increase in VT, with a progressive 15% to 20% decrease in expiratory reserve volume (ERV), 20% to 25% decrease in residual volume (RV), and 20% to 30% decrease in FRC.96 The FRC is further decreased in the supine position at term.96 The decreased FRC may increase uptake and elimination of inhaled anesthetics.23 Along with the change in VT, there is a concomitant increase in inspiratory capacity (IC) by 5% to 10%, thereby allowing the total lung capacity (TLC) to remain relatively stable, with a slight decrease of up to 5% by term.23,96,147 Vital capacity (VC) and inspiratory reserve volume (IRV) are essentially unaltered, although some literature notes a small change in IRV.147,247,250 Peak expiratory flow rates tend to decrease during pregnancy, possibly due to mechanical changes in the respiratory system.95 Physiologic dead space is increased by 60 mL.247
The decreased RV, in conjunction with an elevated metabolic rate, increases the risk of hypoxia if respiratory depression occurs.250 On the other hand, forced expiratory volume in 1 second (FEV1) does not change nor does the FEV1/VC ratio.147,250 These assessments are used in individuals with asthma and are generally thought to be reliable in pregnant women.43,147,250 However, recent studies suggest that peak inspiratory flow rates are altered by maternal position (lower in supine versus standing or sitting) and may in fact decrease to some extent during pregnancy.95
Changes in lung volumes result from the elevation of the diaphragm and the changes in the configuration of the chest. The alteration in RV is also the result of a 35% to 40% decrease in chest wall compliance; lung compliance is unchanged. 43,96,147,247 The decreased chest wall compliance is caused by hormonal influences as well as changes in abdominal pressure. This reduction in compliance allows for more inward movement of the chest wall and reduces the amount of trapped air (residual trapped volume) that contributes to the RV. Therefore the RV decreases 200 to 300 mL. This, along with an approximately 200 mL decrease in ERV, brings the total deficit in the FRC to 500 mL (see Figure 10-2). The FRC falls progressively from 20 weeks’ gestation for a change of 10% to 24% by term.147,250
Lung function
Changes in lung function are related to three major factors: ventilation, airflow, and diffusing capacity.147 Oxygen consumption increases during pregnancy; however, the arterial oxygen pressure (PaO2), though it increases, does not change significantly, even though the arteriovenous oxygen difference decreases. This indicates that there must be a change in ventilation.247
Ventilation.
Minute ventilation (VT x Respiratory rate [RR]) increases up to 30% to 50% during pregnancy.23,147 Changes begin by 8 weeks’ gestation and result in an increase in minute volume, from 6.5 to 7.5 L/min in early pregnancy to 10 to 10.5 L/min at term.108 The elevated resting ventilation exceeds the demands in oxygen consumption, indicating that women tend to hyperventilate during pregnancy.147,247,250 The greater increase in resting ventilation is thought to be due to the stimulatory effects of progesterone on the respiratory center.147 Progesterone may also increase red blood cell levels of carbonic anhydrase B, which facilitates CO2 transfer and lowering of the PCO2.247 The hyperventilation of pregnancy and associated respiratory system alterations are also mediated by the interaction of acid-base balance changes, increased breathing drive, increased central chemoreflex sensitivity, increased metabolism and decreased cerebral blood flow.109–111
The increase in minute ventilation is probably due primarily to the 30% to 40% increase in VT rather than changes in respiratory rate, which usually is unchanged.23,43,96,247 It is much more efficient to increase alveolar ventilation through an increase in VT than with a proportionately equal increase in respiratory rate. The increased metabolic rate and carbon dioxide production also influence VT changes.250 Maximal inspiratory and expiratory pressures are not altered in pregnancy. The reduced RV further enhances alveolar ventilation. This change in RV decreases the amount of gas mixing that occurs with each tidal exchange in the alveolus, thereby improving gas exchange at the alveolar level. Alveolar ventilation increases by 50% to 70% during pregnancy.15,147,247
Airflow.
Airflow is dependent upon resistance encountered in the bronchial tree. Two important determining factors for resistance are smooth muscle tone in the bronchi and the degree of congestion encountered in bronchial wall capillaries. Despite the changes in minute ventilation and the concomitant increase in alveolar ventilation, the work of breathing (airway resistance and lung compliance) remains unchanged. In the larger airways, congestion has very little to do with resistance. Airway resistance does not change significantly during pregnancy because of a balance between bronchoconstricting (PGF2α, decreased RV, and decreased PaCO2) and bronchodilating (PGE2 and progesterone) forces.96,147
Assessment of small airway function is most often determined by evaluation of closing volume (CV) and closing capacity (CC). CV is the point at which the small airways close (collapse and cease to ventilate) in the lowest part of the lung. Small airway (<1 mm) patency is believed to be the result of transpulmonary pressure, compliance of the airway walls, and the presence of sufficient surfactant. Closure in the lung bases normally occurs somewhere between the RV and FRC. CV is usually expressed as a percentage of vital capacity (CV/VC). CC is the term applied to the sum of the CV and RV (CC = CV + RV) and is expressed as a percentage of total lung capacity (CC/TLC).43,147,250
Under normal circumstances, airway closure does not occur during tidal breathing. When closure occurs at a higher than normal volume, however, gas exchange may be affected because ventilation to the lung bases is decreased. In pregnancy, airway closure above the FRC has been reported near term and is attributed to the 20% to 30% decrease in ERV.147 Airway closure is more likely to occur in supine versus sitting.147 This alteration changes gas distribution and may result in a decrease in oxygen content and PaO2 in the third trimester.43,147,247,250 When airway closure is at or above FRC, there is the possibility of altering PaO2 due to ventilation-perfusion (V./Q.) mismatch in the lung bases. If these differences are significant, compensatory maternal physiologic responses such as an increase in respiratory rate may result. However, this is not a usual event in healthy women who compensate by changes in the hemoglobin-oxygen dissociation curve (see “Acid-Base Changes”).26
Diffusing capacity.
Diffusing capacity refers to the ease with which gas is transferred across the pulmonary membrane. Diffusion capacity of carbon dioxide may show an increase or no change in early pregnancy, followed by a decrease, reaching a plateau in the second half of pregnancy in many women. These changes are not thought to be clinically significant.23,33,96,147 Carbon dioxide production increases by 30% due to changes in cholesterol and fat metabolism.250 Oxygen consumption increases by 32 to 58 mL/min.171 This change is accounted for by the needs of the fetus (average of 12 mL/min) and placenta (4 mL/min), and by increased maternal cardiac output (7 mL/min), ventilation (2 mL/min), renal function (7 mL/min), and extra tissue in the breasts and uterus (5 mL/min). The increase in carbon dioxide production exceeds the changes in oxygen consumption, leading to an increase in the respiratory quotient from 0.70 to 0.83 by term.247
Acid-base changes
Oxygen consumption, carbon dioxide production, and basal metabolic rate all increase due to the increased metabolic demands from the mother, placenta, and fetus.96 The normal pregnant woman is in a state of compensated respiratory alkalosis, which is thought to be the result of the effects of progesterone on the respiratory system and lung volume changes, especially the increased minute volume.247,250 The result is a reduction in arterial and alveolar carbon dioxide and a slight increase in PaO2. The fall in PCO2 begins early in pregnancy, paralleling changes in ventilation.147,247 Definitions of common terms used in acid-base physiology are listed in Table 6-1 on p. 165.
The purpose of the respiratory alkalosis seems to be facilitation of carbon dioxide transfer from the fetus to the mother by increasing the arterial carbon dioxide pressure (PaCO2) gradient. Hyperventilation leads to average PaCO2 values of 27 to 32 mmHg (3.59 to 4.25 kPaa) and a concomitant decrease in serum bicarbonate levels to between 18 and 21 mEq/L (mmol/L) with a base deficit of −3 to −4 mEq/L (mmol/L).23,147 The latter is a consequence of increased renal excretion of bicarbonate, reflecting a metabolic compensation for the lower PaCO2. The lower PaCO2 produces a gradient within the intervillous space that enhances fetal offloading of carbon dioxide.23 This may be the primary function of the hyperventilation of pregnancy.160 PaCO2 values vary with the altitude at which the mother resides; the decrease in PaCO2 is greater at higher altitudes (compensatory hyperventilation to maintain and adequate PaO2.23,96,247 The pH increases to the high end of normal (7.40 to 7.45) to maintain homeostasis. These changes are stable throughout pregnancy until the onset of labor.33,43,147 The reduction in blood buffer reduces the mother’s ability to compensate for a metabolic acidosis that could develop during prolonged labor or other states in which tissue perfusion may be reduced.
In contrast, PaO2 levels increase from those of prepregnancy (95 to 100 mmHg [12.63 to 13.30 kPaa]) because of the increase in alveolar ventilation. During the first trimester, PaO2 levels range from 106 to 108 mmHg, dropping to 101 to 104 mmHg (13.43 to 13.83 kPaa) by the third trimester.247 Even though the PaO2 level remains elevated, the alveolar-arterial PO2 gradient (AaDO2) is unchanged throughout most of pregnancy, but may increase near term due to an attempt to offset hyperventilation.147 Oxygen delivery is maintained within normal limits during pregnancy due to the increased carbon dioxide, which compensates for changes in the respiratory system and the decrease in red blood cells.247 Supine positioning versus sitting in late pregnancy further decreases PaO2 levels and increases the AaDO2 gradient.147,247 However, these changes are thought to have little clinical significance.147
Oxygen-hemoglobin dissociation curve.
The oxygen-hemoglobin dissociation curve (see Box 10-1 below) demonstrates that once the plateau of the curve is achieved it takes large changes in oxygen concentration in order to make small changes in SaO2. The P50 increases from 26 to 30 mmHg (3.45 to 3.99 kPaa) by term (Figure 10-3), thus decreasing the affinity of hemoglobin and enhancing transfer of oxygen from mother to fetus.247,250 These changes are mediated by the increase in 2,3-diphosphoglycerate (2,3-DPG), which is stimulated by the increase in maternal pH.43 The increased 2,3-DPG shifts the oxygen-hemoglobin dissociation curve to the right to enhance oxygen release to the fetus.
It is unclear whether the small changes seen in PaO2 levels during pregnancy are significant to the maternal-fetal oxygen gradient and therefore to the fetus. Considering that fetal PaO2 levels are between 25 and 35 mmHg (3.32 and 4.65 kPaa), this seems unlikely. However, this increase may reflect increased maternal pulmonary circulation, with a greater volume of blood contained within the pulmonary vasculature at any given point in time. This may be significant at higher altitudes (see “Effects of Altitude and Air Travel”). SaO2 does not change significantly during pregnancy, although saturations below 96% may be seen in women who smoke.
Intrapartum period
The major effect of labor upon the respiratory system is related to the increased muscular work, metabolic rate, and oxygen consumption, which increases 40% to 60% during labor.23 Consequently, alterations in ventilation and acid-base status can be anticipated. The healthy woman tolerates these changes since oxygen delivery is much greater than needs, but this can become a problem in the compromised woman.247
With the onset of labor, there is an increased demand for oxygen; oxygen consumption increases with uterine muscle activity. If there is insufficient time for uterine relaxation and restabilization after a contraction, oxygen content is lower and myometrial hypoxia as well as metabolic acidosis may occur with the next contraction. Over time this can lead to inadequate oxygenation, which increases the severity of the pain experienced. Most studies have evaluated respiratory system changes during active and painful labor. There are few studies evaluating the labor process with the use of sporadic analgesia and psychoprophylaxis. The pain experienced during labor is the result of the interaction of a number of factors (see Chapter 15). The subjective component includes the discomfort that is perceived by the mother. Objective factors are related to changes in the functioning of the cardiorespiratory system, alterations in the autonomic nervous system, and physical changes that occur during labor and delivery.
Ventilatory alterations related to pain vary significantly from patient to patient; therefore, each laboring woman must be evaluated individually. Changes seen during the intrapartum period include an increase in respiratory rate and a change in VT, with a tendency toward further hyperventilation. Hyperventilation is a natural response to pain and becomes evident as the pain and apprehension of labor increase. Hyperventilation is diminished when pain is alleviated.21 Maximal inspiratory pressure decreases during strong expulsive efforts, possibly as a result of development of transient diaphragmatic fatigue.
Although pain seems to be the major cause of this hyperventilatory response, anxiety, drugs, and the use of psychoprophylactic breathing exercises can contribute to the elevated respiratory rate. VT may be further increased during the second stage of labor as hyperventilation following breath holding with expulsive efforts is encountered. Risks of the Valsalva maneuver are discussed in Chapter 4.
Increases in oxygen uptake and minute ventilation are seen during labor, with a significant increase noted between early latent and active phases. Oxygen consumption increases 40% to 60% during labor with further increases (up to twofold) with contractions.33,247 This is balanced by an increase in cardiac output. Women with low oxygen delivery may become compromised with inadequate oxygen delivery to both the woman and fetus.247 The increase in ventilation can lead to a progressive and substantial decline in PaCO2. There are wide variations in values between patients; however, a PaCO2 level around 25 mmHg is representative of what might be encountered during the first stage of labor. A transient decline in PaCO2 occurs with each contraction until cervical dilation is complete, at which time the decline in PaCO2 may be seen even between contractions.
The changes in PaCO2 are markedly reduced or eliminated when continuous lumbar epidural anesthesia is used during labor. Variables affecting PaCO2 levels that need to be considered include breath holding (which elevates PaCO2 levels), compensatory hyperventilation following breath holding, length of contractions, and frequency of contractions. The timing of analgesia administration as well as the frequency and efficacy of the analgesia may also alter PaCO2 values.43 The respiratory alkalosis that ensues from hyperventilation is normally associated with a drop in base excess and possibly a decrease in arterial pH, according to some researchers. Others have documented a rise in pH. In either case, the degree of change indicates that labor and hyperventilation are highly significant events that may lead to alterations in physiologic parameters.21
Significant changes in acid-base status due to hyperventilation and increased oxygen consumption are potentially hazardous to both the mother and fetus.21 Extremely low PaCO2 levels result in cerebral vasoconstriction and possibly reduce intervillous perfusion and blood flow. The alkalemia that results shifts the oxygen-hemoglobin dissociation curve to the left. This shift impairs the release of oxygen from maternal blood to fetal blood, thereby decreasing the availability of oxygen to the fetus during a time when oxygenation may already be impaired due to uterine contractions.21 Hyperventilation may lead to dizziness and tingling in the mother due to low PaCO2 levels. Interventions can include counting respirations out loud to help the mother slow her respiratory rate, letting her know when the contraction is ending so that she can begin to relax, avoiding the Valsalva maneuver, and promoting deep breathing between contractions to cleanse the system and promote oxygenation and restabilization.
The acid-base changes encountered in the first and second stages of labor quickly reverse in the third stage and postpartum period with compensatory respiratory efforts. These efforts are largely due to a decrease in respiratory rate. Acid-base levels return to pregnancy values within 24 hours of delivery and to nonpregnant levels by several weeks after delivery. Table 10-1 summarizes the changes in arterial blood gases during the intrapartum period.
Table 10-1
Maternal Blood Gas Alterations During the Intrapartum Period
STAGE OF LABOR | PARAMETER | RANGE VALUE | ACID-BASE STATUS |
Early | PaCO2 | 21 to 26 mmHg (2.79 to 3.45 kPaa) | Respiratory alkalosis |
Plasma base deficit | −0.9 to 26.9 mEq/L (mmol/L) | ||
Blood pH | 7.43 to 7.49 | ||
End of first stage | PaCO2 | 21 to 35 mmHg (2.79 to 4.65 kPaa) | Mild metabolic acidosis compensated by respiratory alkalosis |
Plasma base deficit | −1.2 to 29.2 mEq/L (mmol/L) | Mild respiratory acidosis during bearing down | |
Blood pH | 7.41 to 7.54 | ||
End of second stage (delivery) | PaCO2 | 16 to 24 mmHg (2.12 to 3.19 kPaa) | Metabolic acidosis uncompensated by respiratory alkalosis |
Plasma base deficit | −2.3 to −12.3 mEq/L (mmol/L) | ||
Blood pH | 7.37 to 7.45 |
From Burgess, A. (1979). The nurse’s guide to fluid and electrolyte balance. New York: McGraw-Hill.
Research on the effects of labor on acid-base status has been conducted in conjunction with the delivery of epidural analgesia. Psychoprophylaxis (psychoanalgesia) has been shown to reduce the need for medication, reduce tension and pain by self-report, and engender a positive attitude toward the labor and delivery experience. Lamaze psychoprophylaxis is based on the hypothesis that childbirth is a natural physiologic process and that pain can be minimized through education and specified exercises. Education and antenatal preparation are designed to reduce anxiety through knowledge of the processes of labor. Relaxation techniques are designed to reduce skeletal muscle spasm and tension that may contribute to pain. Reduction of pain sensations and perception is achieved through distraction by utilizing conditional responses such as breathing patterns and other nonpharmacologic measures (see Chapter 15).
Postpartum period
Chest wall compliance changes immediately after delivery due to a decrease in pressure on the diaphragm and reduction in pulmonary blood volume. A 20% to 25% increase in static compliance has been reported following delivery. Changes in rib cage elasticity may persist for months after delivery.250 VT and RV return to normal soon after delivery, whereas ERV may remain in an abnormal state for several months. As progesterone levels fall in the first 2 days after delivery, PaCO2 levels rise. Diffusing capacity, which at term is slightly below postpartum levels, increases during the postpartum period. Overall, anatomic changes and ventilation return to prepregnant status by 24 weeks after delivery, although the subcostal angle tends to remain about 20% wider than prepregnancy vaues.96
Clinical implications for the pregnant woman and her fetus
The respiratory changes that occur with pregnancy can be annoying as well as limiting in some circumstances. Common complaints and experiences include dyspnea, capillary engorgement of the upper respiratory tract, and altered exercise tolerance. As a result, modifications in activity levels as well as in the activities themselves may need to be considered. Discussions with women regarding their usual activities can help provide sufficient information for determining when change is needed. In addition, changes in the maternal respiratory system can influence the course of disease processes (e.g., asthma in some women), affecting not only the mother but also the fetus. Specific areas addressed in this section include dyspnea of pregnancy, upper respiratory capillary engorgement, respiratory infections, asthma, smoking, and anesthesia. Exercise is discussed briefly; further information can be found in Chapter 9.
Dyspnea
The sensation of dyspnea is reported by 60% to 70% of pregnant women, usually beginning during the first or second trimester (Figure 10-4). A maximal incidence is reached between 28 and 31 weeks and remains relatively stable to term.147,247,250 Because abdominal girth has not increased significantly at the time of dyspnea onset, intraabdominal pressure cannot be ascribed as the cause.
Dyspnea during pregnancy is a physiologic dyspnea; that is, it occurs even at rest or with mild exertion.250 The exact cause remains unclear, but it is thought to be due to the increased respiratory drive and load, changes in oxygenation, or a combination of these events.250 Increased sensitivity to carbon dioxide and hypoxia may be important contributing factors, especially in early pregnancy. During late pregnancy, mechanical factors may aggravate these changes.171 The increased VT and lower PaCO2 have also been implicated. In addition, the heightened maternal awareness of the normal hyperventilation of pregnancy might result in the sensation of dyspnea. An improvement in symptoms in some women with increasing gestation suggests an adjustment to this normal process.
Dyspnea can be quite uncomfortable and anxiety provoking. The hyperventilation and dyspnea may decrease the ability of some pregnant women to maintain their usual activity levels. Pathologic dyspnea—which may occur with disorders such as pulmonary emboli (a complication sometimes seen in pregnancy)—must be differentiated from physiologic dyspnea during pregnancy. Pathologic dyspnea is characterized by respiratory rates greater than 20, PCO2 below 30 mmHg (3.99 kPaa) or greater than 35 mmHg (4.66 kPaa), or abnormal FEV1.250
Upper respiratory tract capillary engorgement
Hormonal changes (especially the increase in estrogens) along with the increased blood volume, hyperemia, edema, glandular hypersecretion and increased phagocyte activity alter the mucosa of the oro- and nasopharynx with capillary engorgement throughout the respiratory tract.96 Progesterone may contribute to engorgement by inducing vascular smooth muscle relaxation and nasal vascular pooling. Increased circulating blood volume may also play a role in this pooling. The results of these changes can be uncomfortable to some women and may be exacerbated in women with preeclampsia.137 With preeclampsia, the airways may be narrower due to soft tissue edema.169 In some situations these changes can be hazardous. For example, swelling of the airway along with other physiologic changes in pregnancy (e.g., weight gain, changes in the gastrointestinal system, and increased total body water) increase the risk for the pregnant woman who requires intubation for obstetric or nonobstetric problems.23,71,169 The rate of failed intubations is seven to eight times higher during pregnancy.23
The nasopharynx, larynx, trachea, and bronchi may become swollen and reddened. For some individuals this may be uncomfortable, but it usually does not pose any unusual difficulties. These symptoms can be markedly aggravated with minor upper respiratory infections and preeclampsia. The swelling can lead to inflammation (noninfective in nature) that causes changes in the voice (e.g., hoarseness), make nose breathing difficult, and increase the incidence of nosebleeds. Abrasions and lacerations of the mucosa may occur, and bleeding may ensue.21,71,169
Rhinitis (defined as nasal congestion that lasts 6 or more weeks without signs of infection or allergy) is seen in up to 30% of all pregnant women and in approximately two thirds of those who smoke.62,84,96,137 These symptoms may appear any time but usually begin in the second trimester and parallel increasing estrogen levels.137 Estrogens and placental growth hormone (a stimulator of nasal mucosal growth) have been implicated in the etiology of pregnancy rhinitis.62,84,96 Rhinitis usually disappears by 48 hours postpartum, but may take up to 2 weeks in some women.96 Upper respiratory tract changes may also increase snoring and exacerbate sleep-disordered breathing or increase obstructive sleep apnea.23,26 These changes are discussed further in Chapter 15.
Exercise
The effect of exercise on the respiratory system is related to alveolar ventilation and is dependent upon the age, body weight, body composition, and physical condition of the individual. Cardiovascular function, uterine blood flow, respiratory function, blood gases, aerobic capacity, metabolism, temperature, and psychologic state are all affected by exercise. Cardiorespiratory changes with exercise during pregnancy are discussed further in Chapter 9.
The usual changes in maternal respiratory function during pregnancy are similar to those with mild to moderate exercise.111,180 Studies of respiratory rates with exercise in pregnant and nonpregnant women demonstrate that respiratory rates in pregnant women are higher than those in nonpregnant women during mild exercise, but this difference disappears during moderate exercise. VT and minute volume remain higher in pregnant subjects during all levels of exercise.180 Ventilation increases 38% and oxygen consumption is 15% greater during exercise in pregnant versus nonpregnant women.147,180 The efficiency of gas exchange does not seem to be impaired in the pregnant woman during exercise. During prolonged exercise, however, PaO2 increases and PaCO2 decreases. This decrease in PaCO2 is most likely due to the effects of progesterone on the respiratory center. Oxygen consumption also increases with advancing gestational age. Part of this increase is due to the increased work of carrying the extra weight associated with pregnancy. Exercise-induced changes in acid-base balance are similar in pregnant and nonpregnant women.
Effects of altitude and air travel
With increasing altitude, compensatory decreases in PaO2 and changes in other parameters can be seen. These are an attempt by the maternal system to maintain higher PaO2 levels under relatively hypoxic conditions.147,153 The change in altitude has a significant effect on oxygen saturation and changes the oxygen-hemoglobin dissociation curve. Pregnant women living at high altitude have a higher minute ventilation, FRC, TLC, forced VC, forced expiratory volume, and diffusion capacity than women at sea level.23,121 The hyperventilation of pregnancy is also accentuated, and maternal dyspnea may be more prominent; therefore patients need to be given assurance that it is normal.8 The woman increases her ventilation and oxygen saturation during the first trimester, while diffusing capacity decreases to the third trimester, leading to decreased oxygen content by term. These changes are not significant if the woman comes from a family that has lived at altitude for at least 3 generations.23,121 Pregnancy at high altitude is also associated with morphologic differences in placental villi with increased capillary diameter.64 Women living at altitude have lower birth weight infants. Birth weight is decreased by an average of 100 g for each 3000 ft (1000 m) increase in altitude.23
Commercial aircraft are usually pressurized to 6000 to 8000 feet (1829 to 2434 meters) above sea level, so flying results in a transient exposure to altitude. During ascent, the pregnant woman may experience transient cardiopulmonary adaptations (increased heart rate and blood pressure; decreased aerobic capacity).4 The fetal heart rate may also increase transiently during both takeoff and landing, but stays within normal limits.71 Pregnant women who travel by airplane may also experience an increase in dyspnea and respiratory rate as their bodies attempt to compensate for the increased altitude. Fetal hemoglobin (HbF) and the fetal circulation protect the fetus from desaturation during commercial flights.201 Thus flying in commercial aircraft is generally not a risk in women with uncomplicated pregnancies.4,35,71,145 The American College of Obstetricians and Gynecologists states that “in the absence of obstetric or medical complications, pregnant women can observe the same general precautions for air travel as the general population and can fly safely.”4 Most airlines allow travel up to 36 weeks of pregnancy, with travel up to 7 days before the woman’s expected due date permitted with a certificate from their health care provider.4 For international travel the cutoff may be earlier.