Renal system and fluid and electrolyte homeostasis
The kidneys are critical organs in maintaining body homeostasis by regulation of water and electrolyte balance, excretion of metabolic waste products and foreign substances, regulation of vitamin D activity and erythrocyte production (via erythropoietin), and gluconeogenesis.161 The kidneys also have an important role in control of arterial blood pressure through the renin-angiotensin system and regulation of sodium balance. This chapter examines the alterations in basic renal processes and regulation of fluids and electrolytes observed in the pregnant woman, fetus, and neonate, and discusses the implications of these changes for clinical practice. Basic renal processes are summarized in Figure 11-1 and Box 11-1 on page 358.
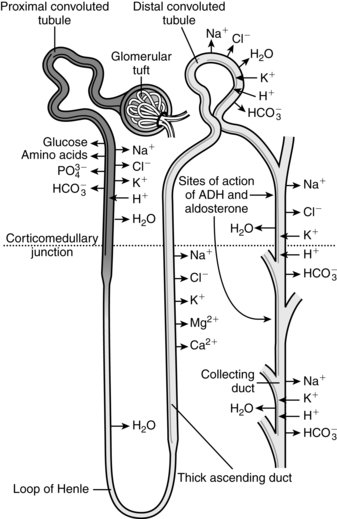
Maternal physiologic adaptations
Antepartum period
The renal system must handle the effects of increased maternal intravascular and extracellular volume and metabolic waste products as well as serve as the primary excretory organ for fetal wastes. Changes in the renal system are related to hormonal effects (particularly the influence of progesterone on smooth muscle), pressure from the enlarging uterus, effects of position and activity, and alterations in the cardiovascular system and vasoactive substances. Cardiovascular system changes that interact with alterations in renal hemodynamics include increased cardiac output, increased blood and plasma volume, and alterations in the venous system and plasma proteins (see Chapters 8 and 9). The predominant structural change in the renal system during pregnancy is dilation of the renal pelvis and ureters; functional changes include alterations in hemodynamics, glomerular filtration, and tubular handling of certain substances. Changes in fluid and electrolyte homeostasis result from changes in renal handling of water and sodium and alterations in the renin-angiotensin system. Table 11-1 summarizes changes in the renal system during pregnancy and their clinical implications. Even with these changes, renal reserve is maintained during pregnancy, so that the pregnant woman has the capacity for further vasodilation and filtration above baseline if needed.
Table 11-1
Changes in the Renal System During Pregnancy
PARAMETER | ALTERATION | SIGNIFICANCE |
Renal calyces, pelvis, and ureters |
Structural changes
Pregnancy is characterized by physiologic hydroureter and hydronephrosis. Significant dilation of the renal calyces, pelvis, and ureters beginning as early as the seventh week, is seen in up to 80% of women.21,53,78,113,125 The mean kidney length increases by approximately 1 cm due to the increased renal blood flow (RBF), renal vascular and volume, and renal hypertrophy.66,113 Renal volume increases by 30%.78,113 The diameter of the ureteral lumen increases with hypertonicity and hypomotility of the ureteral musculature. Hypomotility and reduced peristaltic movements of the ureters may be mediated by prostaglandin E2 (PGE2).21,54
The ureters elongate and become more tortuous, especially during the last half of pregnancy as they are laterally displaced by the growing uterus.21 These changes are seen within the renal pelvis and the upper portion of the ureters to the pelvic brim. The portion of the ureters below the pelvic brim (linea terminalis) is usually not enlarged. The ureters may contain as much as 300 mL of urine by the third trimester.54 This reservoir of urine leads to stasis and can interfere with evaluation of glomerular filtration rate (GFR), tubular function, and influence the accuracy of 24-hour urine collections.113,125 The stasis increases the risk of ascending urinary tract infection (UTI), nephrolithiasis, and pyelonephrosis.53,68,70,113,125
The etiology of physiologic hydroureter is unclear. Dilation begins before the uterus reaches the pelvic brim, so initial changes are probably hormonally mediated. Hormonal influences, particularly progesterone, may induce hypertrophy of the longitudinal smooth muscles surrounding distal portions of the ureters and hyperplasia of periurethral connective tissue.21,54,113 This may lead to a temporary stenosis and mild dilation of the upper portion of the ureters. These findings are similar to those seen in women on oral contraceptives and in postmenopausal women given estrogen and progesterone.54
The major contributing factor to hydronephrosis in later pregnancy is probably external compression of the ureters at the pelvic brim. As pregnancy progresses, the ureters are compressed at the pelvic brim by the iliac arteries, enlarging ovarian vein complexes, and the growing uterus, leading to further marked dilation and urinary stasis.51 Dilation is more prominent in the primipara, whose firmer abdominal wall may increase resistance and pressure on the ureters.21
In most women, the right ureter is dilated to a greater extent than the left.51,78,113 These differences become more prominent after midgestation.51 The right ureter makes a right-angle turn as it crosses the iliac and ovarian veins at the pelvic brim; the turn of the left ureter is less acute, and it parallels rather than crosses the left ovarian vein. The iliac vessels are more rigid on the right than on the left, thus further compressing the ureters. Compression is maximal by around 30 weeks’ gestation, with no further significant changes to term. The sigmoid colon contributes to dextroversion of the uterus and may increase ureteral compression on the contralateral side during the last trimester.21,54,78,113 The position of the fetus does not seem to influence ureteral dilation. The site of placental attachment may increase venous flow on that side with subsequent compression of the ureters by the dilated vessels.54
Bladder tone decreases as a result of the effects of progesterone on smooth muscle. Bladder capacity doubles by term. The bladder becomes displaced anteriorly and superiorly by the end of the second trimester. Under the influence of estrogen, the trigone undergoes hyperplasia with hypertrophy of the bladder musculature. The bladder mucosa becomes hyperemic with increased size and tortuosity of the blood vessels. The mucosa becomes more edematous and vulnerable to trauma or infection after engagement of the presenting part.54
The baseline intravesical pressure doubles due to the enlarged uterus.106 The decreased bladder tone and flaccidity may lead to incompetence of the vesicoureteral valve and reflux of urine. Vesicoureteral reflux is seen in up to 3.5% of pregnant women, especially in the third trimester.106 Predisposing factors for this reflux include hypertrophy and hyperplasia of the ureteral wall, increased elasticity of the ureters, increased bladder pressure, and decreased peristalsis in the distal ureter.106 Alterations in bladder placement by the growing uterus stretch the trigone and displace the intravesical portion of the ureters laterally. This shortens the terminal ureter, decreasing intravesical pressure. If intravesical pressure subsequently increases with micturition, urine regurgitates into the ureters.54
Urodynamic changes
Urine output increases from a mean of 1475 to 1919 mL per 24 hours primarily because of changes in sodium excretion.106 Mean flow rate decreases in the second and third trimesters with an increase in flow time and time to maximal flow throughout pregnancy.106 The number of voids per day and mean daily urine output increase throughout gestation.152 Studies of changes in bladder capacity during pregnancy have reported variable findings. Increased bladder capacity due to the decreased tone has been reported by some investigators, especially in primiparas.10 Others have found a mean bladder capacity similar to that of the nonpregnant state in the first two trimesters, but decreased capacity in the third trimester due to elevation of the trigone and the size of the presenting part.142 Another study reported that the pregnant woman’s bladder has a larger capacity with a lower pressure per volume.134 In nonpregnant women the first urge to void was at a bladder capacity of 150 to 200 mL and maximum at 450 to 550 mL with an intravesicular pressure of 20 cmH2O (1.96 kPa); in pregnant women the first urge to void was at a bladder capacity of 250 to 400 mL (intravesical pressure of 4 to 8 cmH2O [0.39 to 0.78 kPa]) and often did not reach maximum until 1000 to 1200 mL (intravesicular pressure of 12 to 15 cmH2O [1.17 to 1.47 kPa]).134
Changes in renal hemodynamics
Significant hemodynamic changes occur within the kidneys beginning early in pregnancy in conjunction with systemic vasodilation (see Chapter 9). RBF and GFR increase. Increases in these parameters, although not to the degree seen in pregnancy, also occur during the luteal phase of the menstrual cycle.78
RBF increases up to 50% to 80% by the mid–second trimester and then slowly decreases to term.91,113 The higher values are seen in the left lateral recumbent position.33 This change is accompanied by increased glomerular filtration rate (GFR), decreased renal vascular resistance (RVR), and activation of the renin-angiotensin-aldosterone system. Renal hemodynamic changes begin before significant expansion of plasma volume and are thought to be primarily related to the decrease in systemic vascular resistance (SVR), which may stimulate decreased RVR leading to increased RBF and GFR, and by sodium retention. These changes may be mediated by nitric oxide (NO), prostacyclin (PGI2), atrial natriuretic factor (ANF), progesterone, endothelin B, and relaxin, possibly via their effects on GFR. Increased flow is enhanced by vasodilation of preglomerular and postglomerular capillaries.33,78,113,125 Another measure of renal hemodynamics is the effective renal plasma flow (ERPF), which increases 80% by midpregnancy.40,113,153 ERPF then gradually decreases during the third trimester to values 50% greater than nonpregnant values.66,113
Changes in glomerular filtration
The GFR increases 40% to 60% during pregnancy.29,34,66,91,113 The rise begins as early as 6 weeks and precedes plasma volume expansion and cardiac output (see Chapter 9).29,91 Changes in GFR are detectable 3 to 4 weeks after conception, with 25% of the increase occurring by 2 weeks postconception, peak at 9 to 16 weeks, then remain relatively stable to near term.113 Values for GFR in pregnancy, as measured by creatinine clearance, average 110 to 150 mL/min.113 Differences in reported values for RBF and GFR during pregnancy vary with the method of measurement. The increased GFR is related to the increased glomerular blood flow (and glomerular capillary hydrostatic pressure) and decreased colloid osmotic (plasma oncotic) pressure due to a reduction in the concentration of plasma proteins.71,91 Failure of the GFR to increase early in pregnancy has been associated with pregnancy loss.163
The increases in renal plasma flow (RPF) and GFR parallel each other, although RPF changes are slightly greater than GFR changes. This alters the filtration fraction (GFR/RPF), the portion of the RBF that is filtered.70,78,113 The filtration fraction is decreased in the first half of gestation and increased in late gestation as ERPF decreases.78,91 As a result, renal excretion of amino acids, glucose, protein, electrolytes, and vitamins increases, whereas serum urea, creatinine, blood urea nitrogen (BUN), and uric acid levels decrease (Table 11-2).
Table 11-2
Changes in Laboratory Values Associated with Renal Function during Pregnancy
VARIABLE | NONPREGNANT VALUES | VALUES DURING PREGNANCY | VALUES REQUIRING FURTHER INVESTIGATION |
Creatinine clearance | 85-120 mL/min | 110-150 mL/min | |
Plasma creatinine | 0.65 ± 0.14 mg/dL | 0.46 ± 0.13 mg/dL | >0.80 mg/dL |
Blood urea nitrogen | 13 ± 3 mg/dL | 8.7 ± 1.5 mg/dL | >14 mg/dL |
Urinary protein | <100-150 mg/24 hours | <250-300 mg/24 hours | >300 mg/24 hours |
Urinary glucose | 20-100 mg/24 hours | >100 mg/24 hours (up to 10 g/24 hours) | |
Plasma urate | 4-6 mg/dL | 2.5-4 mg/dL | >5.8 mg/dL |
Urinary amino acids | Up to 2 g/24 hours | >2 g/24 hours |
The causes of the increased GFR during pregnancy are still not completely clear, but the primary factor is the increased RPF and decreased renal vascular resistance.32,91,144 These changes are stimulated by relaxin (which up-regulates vascular gelatinase activity and leads to activation of endothelin receptors causing vasodilation), increased GFR, and relaxation of the small renal arteries. This process is mediated by NO, which is a potent renal vasodilator.41,91 Other factors that may play a role are prostacyclin, ANF, human placental lactogen, and human chorionic gonadotropin, which stimulates relaxin secretion.32,33,41
During pregnancy, 24-hour urine volumes are higher due to the increased GFR. The degree to which renal handling of substances is altered during pregnancy depends on the renal process involved (see Figure 11-1). For example, because urea and creatinine are processed only by glomerular filtration, the increased GFR leads to a significant decrease in serum urea and creatinine levels.91,113 The increased GFR also alters renal excretion of drugs (see Chapter 7).9
Alterations in tubular function
Renal glucose excretion increases soon after conception and remains high to term. Urinary glucose values may be 10- to 100-fold greater than the nonpregnant values of 20 to 100 mg per 24 hours due to alterations in reabsorption of glucose in the loop of Henle and collecting ducts.40,113 Glycosuria is more common during pregnancy and can vary from day to day and within any 24-hour period. Glycosuria is discussed in Evaluation of Renal Function during Pregnancy.
Excretion of amino acids, urea, and protein increases in pregnancy. Protein excretion rises from less than 100 mg per 24 hours to up to 250 to 300 mg per 24 hours, with marked day-to-day variation.40,66 The primary amino acids whose excretion is markedly increased throughout pregnancy are alanine, glycine, histidine, serine, and threonine; excretion of cysteine, leucine, lysine, phenylalanine, taurine, and tyrosine increases in early pregnancy, but decreases later.113 Increased urea clearance leads to decreased plasma urea nitrogen levels by 8 to 10 weeks.40 Plasma urea levels may be only 63% of nonpregnant values by the third trimester.
Proteinuria occurs more frequently during pregnancy with increases in both total protein and urinary albumin.78 The filtered load of amino acids during pregnancy may exceed tubular reabsorptive capacity with small amounts of protein lost in the urine. Values of 1+ protein on dipsticks are common and do not necessarily indicate the presence of glomerular pathology or preeclampsia.5,160 Urinary protein excretion during pregnancy is not considered abnormal until values exceed 300 mg per 24 hours.68,78,113,125 Protein excretion does not correlate with the severity of renal disease, and increased protein excretion in a pregnant woman with known renal disease does not necessarily indicate progression of the disease.40 However, proteinuria associated with hypertension in the pregnant woman is associated with a greater risk of an adverse pregnancy outcome.68,162
Uric acid is normally handled by filtration, secretion, and reabsorption (see Box 11-1 on 358), so less than 10% of the filtered load appears in urine. During pregnancy, filtration of uric acid increases up to 30% in the first 16 weeks, and net reabsorption is decreased and secretion enhanced.163 As a result, serum uric acid levels decrease up to 25% to 35%, beginning as early as 8 weeks, to a nadir of 2 to 3 mg/dL by 24 weeks.78,113 Levels gradually increase toward nonpregnant values after that point as tubular reabsorption of uric acid increases. This increase may also be a result of the rise in RPF that alters the filtration fraction at this stage of gestation.40,113 In women with preeclampsia, uric acid clearance is reduced with higher serum levels.78
Potassium excretion is decreased, with retention of an additional 300 to 350 mEq that is thought to be due to increased proximal tubular reabsorption.91,113 Serum potassium levels do not rise, because the additional potassium is used for maternal tissues and by the fetus. The mechanisms for increased potassium reabsorption in pregnancy are not well documented. These changes occur despite the increase in aldosterone that normally would increase urinary potassium loss. Therefore the altered potassium excretion may be due to antagonistic action of progesterone on renal tubular actions of aldosterone.91
Renal acid-base balance is altered to compensate for the respiratory alkalosis that develops secondary to an increased loss of carbon dioxide from changes in ventilation during pregnancy (see Chapter 10). The respiratory alkalosis is compensated for by increased renal loss of bicarbonate. This is accomplished by renal retention of H+ ions and a decrease in serum bicarbonate. As a result, serum bicarbonate levels fall 4 to 5 mEq/L (mmol/L) during the first trimester to 20 to 22 mEq/L (mmol/L).66,125 This change may limit buffering capacity in the pregnant woman.78
Urinary calcium excretion is increased, possibly due to the increased GFR, and serum calcium and phosphorus levels decrease. This is balanced by increased intestinal absorption of calcium, so serum ionic calcium levels remain stable (see Chapter 17).113 To maintain homeostasis and meet fetal demands, women need 1200 mg of calcium per day in their diet. Excretion of water-soluble vitamins also increases, so maternal diet must be evaluated to ensure adequate supplies of vitamins B1, B2, B6, and C; folate; and niacin (see Chapter 12).
Fluid and electrolyte homeostasis
Sodium homeostasis.
The filtered load of sodium increases up to 50% as a result of the increased GFR. The nonpregnant woman filters approximately 20,000 mEq of sodium per day; the pregnant woman filters 30,000.41,113 To prevent excessive urinary sodium loss, tubular reabsorption of sodium also increases so that 99% of the filtered sodium is reabsorbed. As a result, there is a net retention of 900 to 950 mg (2 to 6 mEq/day) of sodium during pregnancy.40,41,78,113 Sodium retention occurs gradually with an increase in late pregnancy.41 Much of the sodium is used by the fetus and placenta; the rest is distributed in maternal blood and extracellular fluid (ECF) (Table 11-3).163
Table 11-3
Storage of Sodium during Pregnancy
STORAGE SITE | SODIUM (MEQ [MMOL/L]) |
Fetus | 290 |
Edema fluid | 240 |
Plasma | 140 |
Amniotic fluid | 100 |
Uterus | 80 |
Placenta | 57 |
Breasts | 35 |
Red cells | 5 |
Total | 947 |
From Sullivan, C.A. & Martin, J.N. (1994). Sodium and pregnancy. Clin Obstet Gynecol 37, 558.
Despite these alterations, the pregnant woman remains in sodium balance and responds normally to changes in both sodium and water balance. The specific mechanisms for sodium retention during pregnancy are unclear. The maintenance of sodium balance during pregnancy is multifactorial and related to a balance (Figure 11-2) between natriuretic factors favoring sodium excretion (increased GFR, decreased RVR, decreased plasma oncotic pressure, decreased serum albumin, vasodilating prostaglandins, increased ANF, and the diuretic-like and aldosterone-antagonistic actions of progesterone) and antinatriuretic factors favoring sodium conservation (increased renin, aldosterone, deoxycorticosterone, human chorionic somatomammotropin [human placental lactogen], and estrogen).113,161,163 ANF is released by the atrial endothelial lining and increases early in pregnancy. ANF opposes the action of progesterone and inhibits the renin-angiotensin-aldosterone system, thus stimulating sodium loss. Relaxin also mediates sodium balance during pregnancy. Relaxin is associated with osmoregulatory changes, vasodilation, and increased GFR.29,33,125 As a result of these changes, sodium retention in pregnancy is proportional to water accumulation and the woman remains in homeostatic balance.
Renin-angiotensin-aldosterone system
The renin-angiotensin-aldosterone system is important in fluid and electrolyte homeostasis and maintaining arterial blood pressure (Figure 11-3 and Box 11-2 on page 362). Pregnancy is characterized by increases in components of the renin-angiotensin-aldosterone system and decreased sensitivity to the pressor effects of angiotensin II (ANTII).11 Changes in most of these components peak at 30 to 32 weeks. The changes are mediated by estrogens, progesterone, prostaglandins, and alterations in renal processing of sodium.64 Although the renin-angiotensin-aldosterone system is altered during pregnancy, this system responds normally, but at a new set point, to interventions that alter volume such as salt restriction, diuretics, and positional changes.92
During pregnancy, major sites for renin production include the uterus, placenta, and fetus as well as the kidneys.26,75 Renin peaks at levels two to three times higher than normal during the first trimester and remains elevated, with a tendency to reach a plateau by about 32 weeks. The increase in renin is due primarily to increased inactive renin; however, both active renin and plasma renin activity (PRA) (a measure of the capacity of plasma to generate angiotensin I) also increase.64,148 PRA increases 4 to 10 fold during the first trimester, peaking at 12 weeks, and then remaining high to term with a possible decrease in the third trimester noted by some investigators.139,148
Renin release is stimulated by estrogens (which also increase concentrations of angiotensinogen), decreased blood pressure, increased levels of plasma and urinary PGE, and the aldosterone-antagonizing effects of progesterone.11,139,148 Increases in plasma renin are also seen during the later part of the secretory phase of the menstrual cycle, peaking at twice normal levels with the luteinizing hormone surge and persisting until midway into the luteal phase. These changes coincide with progesterone release.
Angiotensinogen (plasma renin substrate) levels double by 8 to 10 weeks, increase twofold to threefold by 20 weeks, and peak at 30 to 32 weeks.11,26 This increase is due to the effects of estrogen on the liver, which synthesizes this substrate.139 Low angiotensinogen levels are associated with spontaneous abortion and may reflect reduced placental estrogen production.
Angiotensin-converting enzyme (ACE) levels may be similar or slightly lower than nonpregnant values, although increases after 30 weeks have been reported.26,139 ACE levels are also high in the uterus and placenta.11 ANTII increases early in pregnancy and peaks at two to three times nonpregnant levels by 30 weeks.26,139 ANTII levels may fall in the third trimester but are still above nonpregnant values.
Plasma aldosterone levels are significantly increased by 8 weeks and reach levels 4 to 6 times higher than those in nonpregnant women by the third trimester.41,66,102,148 Aldosterone increases again later in gestation, peaking at about 36 weeks at levels 8 to 10 times higher than nonpregnant values. The increased aldosterone opposes the sodium-losing effects of progesterone and allows a progressive accumulation of sodium in maternal and fetal tissues.
Despite these changes, the pregnant woman remains responsive to both sodium depletion and loading, suggesting that a new equilibrium has been established.41,148 The expanded intravascular and ECF compartments are sensed as “normal” by the woman’s vascular and renal volume-regulating mechanisms. The elevated levels of aldosterone may be necessary to maintain the expanded extracellular volume. This new equilibrium is protected against further increases or depletion in a manner similar to that in nonpregnant individuals.66
ANTII is a potent vasopressor. Yet despite markedly elevated levels during pregnancy, the blood pressure does not rise and in fact actually decreases along with the peripheral vascular resistance (see Chapter 9).11 The basis for resistance of the pregnant woman to the pressor effects of ANTII and other vasoactive substances is unclear. This refractoriness may be due to decreased vascular smooth muscle responsiveness to ANTII, perhaps mediated by local action of vasodilating prostaglandins, such as PGI2, and progesterone.75,77,151 Other mechanisms include down-regulation of angiotensin receptors and the effects of endothelial-derived relaxing factors such as NO.151 The result is an estimated 60% decrease in sensitivity of the systemic vasculature to the pressor effects of ANTII during pregnancy. Diurnal variations in PRA, ANTII, aldosterone, and angiotensin sensitivity during pregnancy have been reported.42
Preeclampsia is associated with a suppression of the renin-angiotensin-aldosterone system.26,76,77 The increases in renin, aldosterone, and ANTII are smaller in women with preeclampsia than in normotensive women. The preeclamptic woman is highly sensitive to the vasopressor effects of ANTII. The angiotensin II type I receptor agonistic autoantibodies seen in preeclamptic women may play a role in the pathogenesis of preeclampsia (see Chapter 9).76,77
Volume homeostasis and regulation of osmolarity
Because ECF volume is determined by sodium, the accumulation of sodium in pregnancy is accompanied by accumulation of water. Both extracellular and intravascular volumes expand during pregnancy. The largest portion of this expansion is in the vascular component, favoring placental perfusion. The amount of water filtered by the kidneys increases 50% or more during pregnancy due to the increased GFR. Pregnant women accumulate 6 to 7 L of water (Table 11-4) to meet their needs and those of the fetoplacental unit.92,113 About 70% to 75% of maternal weight gain is due to increased body water in the extracellular spaces. Interstitial fluid volume increases 2 to 3 L beginning at 6 weeks and peaks at 24 to 30 weeks, with the greatest accumulation during the second half of pregnancy.41,163 Accumulation of greater than 1.5 L of interstitial fluid is associated with edema. Alterations in blood volume are discussed in Chapters 8 and 9.
Table 11-4
TOTAL WATER | EXTRACELLULAR | INTRACELLULAR | |
Fetusa | 2414 | 1400 | 1014 |
Placentab | 540 | 260 | 280 |
Amniotic fluidc | 792 | 792 | 0 |
Uterusd | 800 | 528 | 272 |
Mammary gland | 304 | 148 | 156 |
Plasma | 920 | 920 | 0 |
Red cells | 163 | 0 | 163 |
Total | 5933 | 4048 | 1885 |
For the purposes of the above estimates, the following assumptions have been made:
aExtracellular space = 41.2% of body weight.
d66% of water is extracellular.
From Davison, J.M. (1997). Edema in pregnancy. Kidney Int Suppl, 59, S90.
The exact mechanisms for water retention in pregnancy are still unclear with disagreement regarding the significance of these volume changes during pregnancy and how pregnant women “sense” these changes.91,92,99 Lindheimer noted: “All agree that normal pregnancy is characterized by an absolute increment in both extracellular and intravascular volume (by some 6–7 L, at that), but investigators disagree on the meaning of these changes. For some (‘under-fill’ theory) it is an incomplete response to both the systemic vasodilation and markedly increased arterial global compliance characteristic of normal pregnancy, and indeed, the lower blood pressure and markedly stimulated renin-angiotensin system and aldosterone levels that persist during normal pregnancy are consistent with this paradigm. For others, this is an absolute hypervolemia perhaps due to the higher levels of salt-retaining steroids that accompany gestation (‘over-fill’ theory), and to still others, there is a constant resetting of the ‘volumestat’ as pregnancy progresses (‘normal-fill’ theory), the gravida always acting as if her current volume status were ‘normal’. ”92, p. 1712
The increase in plasma volume occurs despite decreases in plasma osmolarity and colloid osmotic pressure, changes that would normally stimulate decreases in intravascular volume. Estrogen and progesterone may play a role through dilation of the venous capacitance vessels so that they can accommodate additional volume without stimulation of atrial baroreceptors to alter AVP and aldosterone release.40
Plasma osmolarity decreases from conception, reaching 8 to 10 mOsm/kg below nonpregnant values of 290 mOsm/kg by 10 weeks’ gestation and remaining low to term.41,66,78,113,125,137,151 This change is associated with changes in sodium, urea, and other ions and may arise from the decrease in PCO2 and subsequent compensatory adjustments in renal ion excretion. A decrease in plasma osmolarity of this magnitude in a nonpregnant person would significantly reduce the osmotic threshold for thirst, suppress AVP release, and lead to a massive water diuresis (as occurs in diabetes insipidus). However, the pregnant woman senses this change in osmolarity as normal. At this new baseline, she responds to water loading and deprivation and concentrates and dilutes urine in a manner similar to that in nonpregnant individuals.113 Because of these changes, the urine of pregnant women is concentrated at levels below nonpregnant values.
Arginine vasopressin.
AVP (also known as antidiuretic hormone) secretion and its effect on renal reabsorption of water are similar in pregnant and nonpregnant women, as is AVP secretion in response to changes in baseline plasma osmolarity.163 During pregnancy, the osmostat for AVP is reset, so the threshold at which the osmoreceptors signal the need for increased release is reduced from 280 to 270 mOsm/kg.27,41,66,125 The thirst threshold (usually 10 mOsm/L [mmol/L] above the osmostat) is also reset during pregnancy, falling from 290 to 280 mOsm/L (mmol/L).66,137
AVP metabolites increase in pregnancy, probably due to placental metabolism, but maternal circulating AVP levels do not change significantly. The threefold to fourfold increase in plasma AVP clearance, which peaks at 22 to 24 weeks, is secondary to placental vasopressinases.66,151 The posterior pituitary responds to this increased clearance by releasing more AVP to maintain normal plasma levels.66 These changes in osmoregulation parallel the progressive increase in vasopressin metabolism and placental vasopressinases. The increased plasma vasopressinase in multiple pregnancies may be a basis for polyuria in these women.137
Nonosmotic factors regulating AVP secretion in pregnancy are poorly understood. In nonpregnant individuals a decrease in arterial blood pressure stimulates AVP secretion. In pregnancy the fall in plasma osmolarity occurs weeks before the decline in blood pressure, and the lowered osmolarity is still sensed as normal at term when the blood pressure has returned to nonpregnant values. AVP release is also influenced by plasma volume (decreased plasma volume increases AVP release and increased plasma volume decreases AVP release). During pregnancy the threshold for the release of AVP is reset to accommodate the increase in extracellular volume at a lower baseline plasma osmolarity.40,66 Human chorionic gonadotropin may also have a role in resetting the thirst and osmostat receptors during pregnancy.66 The lowering of the osmotic threshold is probably due to the systemic arterial vasodilation leading to vasopressin simulation and up-regulation of aquaporin 2 water channels in the collecting ducts.137
Intrapartum period
Because the changes in renal function may also affect handling and excretion of drugs, drug doses and responses must be carefully monitored (see Chapter 7). General anesthesia decreases GFR, RPF, and sodium excretion and is associated with renal vasoconstriction, which may be magnified by the effects of stress with catecholamine release.127 Thus monitoring of fluid and electrolyte status is especially important following use of general anesthesia for cesarean birth or with nonobstetric surgery during pregnancy.
Some bladder and urethral trauma probably occurs in most women during the intrapartum period.106 With contractions, intravesicular pressure increases by about 5 cmH2O (0.49 kPa); however, bearing down increases pressure by up to 50 cmH2O (4.90 kPa). Therefore prolonged straining during the second stage of labor may be more important in causing injury to the bladder than are contractions.106
Postpartum period
RPF decreases markedly in the first 5 days after delivery.129 GFR remains elevated (by about 40%) during the first day postpartum and then decreases over the next 2 weeks but remains about 20% elevated.71 RPF, GFR, plasma creatinine, creatinine clearance, and BUN return to nonpregnant levels by 2 to 3 months postpartum.10,129 Urinary excretion of calcium, phosphate, vitamins, and other solutes generally returns to normal by the end of the first week, but hyperfiltration may be maintained for up to 4 weeks due to decreased glomerular oncotic pressure.148 Immediately after delivery, the creatinine clearance increases, but by 6 days is similar to nonpregnant levels.40 PRA and ANTII concentrations fall to nonpregnant values immediately after delivery and then rise again and remain elevated for up to 14 days.148 These changes may reflect the loss of renin from the fetoplacental unit, with subsequent “overshooting” by the maternal system.148 Urinary glucose excretion returns to nonpregnant patterns by 1 week postpartum, and pregnancy-associated proteinuria is resolved by 6 weeks.21,40 Plasma osmolality returns to nonpregnant levels by 2 weeks.151
The postpartum period is characterized by rapid and sustained natriuresis and diuresis, especially prominent on days 2 to 5, as the sodium and water retention of pregnancy is reversed.40 Fluid and electrolyte balance is generally restored to nonpregnant homeostasis by 21 days postpartum and often earlier. Persistence of more than a trace of edema after this time is indicative of sodium retention or a protein-losing state.
The decrease in oxytocin contributes to diuresis because oxytocin acts similarly to AVP in promoting reabsorption of free water. As oxytocin levels decrease, the diuresis becomes more pronounced, with up to 3000 mL of urine excreted per 24 hours on the second through fifth days after delivery.38,66,101 A normal voiding for the postpartum woman may be 500 to 1000 mL, several times greater than a nonpostpartum individual. Water may also be lost via night sweats.
The alterations in tone of the ureters and bladder during pregnancy do not permanently impair function of these structures in most women unless damage from infection has incurred.21,102,113 Morphologic changes in the urinary tract may last 3 to 4 months.66,113 In many women the dilation of the bladder, ureters, and renal pelvis has decreased significantly by the end of the first week, although the potential for distensibility of these structures may persist for several months. In most women these structures return to their nonpregnant state by 6 to 8 weeks; in some women these changes may persist for 12 to 16 weeks or longer.40,66 In up to 10% of women, the anatomic changes in the ureters and bladder persist.129
The decreased tone, edema, and mucosal hyperemia of the bladder can be aggravated immediately postpartum by prolonged labor, forceps delivery, analgesia, or anesthesia.21 These events may also lead to submucosal hemorrhages. Pressure of the fetal head on the bladder during labor can result in trauma and transient loss of bladder sensation in the first few days or weeks postpartum. This can lead to overdistention of the bladder, with incomplete emptying (in about 20%) and an inability to void.129 Stress incontinence is also seen postpartum, although it usually develops before delivery.152 Altered sphincter tone may increase the frequency of incontinence with events such as coughing.
Decreased urine flow rates are seen after vaginal delivery, with an increased voided volume, total flow time, and time to peak flow on the first day postpartum returning to nonpregnant levels by 2 to 3 days.128 Urinary retention is reported in 1.7% to 17.9% of women and is more common after the first vaginal delivery, epidural anesthesia, and catheterization before delivery.134 Retention is a result of the continuing bladder hypotonia after delivery without the weight of the pregnant uterus to limit its capacity.134
Clinical implications for the pregnant woman and her fetus
Urinary frequency, incontinence, and nocturia
Urinary frequency (more than 7 daytime voidings) occurs in about 60% of women.106 Urinary frequency is progressive and maximum at term.152 Frequency begins in the first trimester before the uterus is large enough to put significant pressure on the bladder.152 Throughout most of pregnancy, urinary frequency is primarily due to the effects of hormonal changes, hypervolemia, and the increased RBF and GFR.53,152 Pressure of the pregnant uterus probably influences urinary frequency during the third trimester. Alterations in bladder sensation postpartum can lead to overdistention with incomplete emptying and overflow incontinence.21
During pregnancy, 30% to 50% of women (versus about 8% of nonpregnant women) experience incontinence.106 Urinary incontinence can begin in any trimester, but once it begins, an increase in severity until delivery is noted.106,142,152 An increase is seen in both stress incontinence and urge incontinence.53 Stress incontinence is reported in up to 41% of pregnant women, urge incontinence in 3% to 15%.53,55 Urinary incontinence regresses after delivery in the majority of women, but often returns in subsequent pregnancies.106 Onset of stress incontinence after delivery is associated with continued symptoms at 1 year postpartum in 24% of women.55
Nocturia results from increased sodium excretion, with an obligatory, concomitant loss of water. During the day, water and sodium are trapped in the lower extremities because of venous stasis and pressure of the uterus on the iliac vein and inferior vena cava. At night, when the pregnant woman lies down, pressure on the iliac vein and inferior vena cava is reduced, promoting increased venous return, cardiac output, RBF, and glomerular filtration with subsequent increase in urine output. However, since pregnant women excrete large amounts of sodium at night, diurnal differences in sodium, and therefore water, excretion may be the primary cause of nocturia.53,106 Nursing interventions for women experiencing urinary frequency and nocturia are summarized in Table 11-5.
Table 11-5
Nursing Interventions for Common Problems during Pregnancy Related to the Renal System
PROBLEM | NURSING INTERVENTIONS |
Urinary frequency |
Avoid the upright position for extended periods.
Rest in the left lateral recumbent position with legs slightly elevated.
Elevate the legs and feet at regular intervals and when sitting.
Use support hose or elastic stockings.
Avoid tight clothing on lower extremities (e.g., tight pants, socks, girdles, garter belts, knee-high stockings).
Restrict intake of high-salt foods and beverages.
Assess for signs of preeclampsia (e.g., increased blood pressure, proteinuria, generalized edema).
Dependent edema
Dependent edema is seen in up to 70% of pregnant women and is more common as pregnancy progresses.41 Edema is more common in obese women and is associated with larger babies.41 The forces resulting in the movement of fluid out of the vascular space include capillary hydrostatic pressure and colloid osmotic (plasma oncotic) pressure, which is generated primarily by albumin.41 Compression of the iliac vein and inferior vena cava by the growing uterus increases capillary hydrostatic pressure below the uterus, with filtration of fluid into the interstitial spaces of the lower extremities. The net reduction in plasma albumin during pregnancy reduces plasma colloid osmotic pressure, interfering with return of fluid to the vascular compartment. However, Theunissen and colleagues suggest that the increased interstitial fluid is not due to the decreased plasma oncotic pressure, because interstitial oncotic pressure is reduced to an even greater degree by increased flow of protein into the lymphatic system to maintain the transcapillary osmotic gradient.151 They suggest the basis of the edema is alterations in capillary permeability and changes in interstitial ground substance. These changes reduce the margin of safety against edema, but increase the margin of safety for vascular engorgement and provide a transcapillary pool of fluid that can be mobilized with delivery.151
Dependent edema is more likely to develop in women who are in supine or upright positions for prolonged periods. The development of edema in pregnancy is associated with the amount of water accumulation in maternal tissues. Women with no visible edema have an accumulation of approximately 1.5 L. Pedal edema is associated with an accumulation of 2 L or more; women with generalized edema have accumulated 4 to 5 L or more of water. Edema in the lower legs increases the risk of varicosities and thromboembolic complications (see Chapter 8). Nursing interventions are summarized in Table 11-5 and include leg elevation, increased fluids, activity changes, and water immersion.41 Leg elevation uses gravity to decrease capillary hydrostatic pressure and thus reabsorption of interstitial fluid into the vascular space.41 Water immersion also changes hydrostatic pressure to help move water back into the vascular space. Increased urine flow and diuresis are noted after immersion.
Effects of position on renal function
Position can markedly alter renal function during pregnancy, especially during the third trimester. These postural effects are magnified in women with preeclampsia and hypertension. As pregnancy progresses, there is pooling of blood in the pelvis and lower extremities while sitting, lying supine, or standing. The pooling of blood leads to a relative hypovolemia and decreased cardiac output. In order to compensate and maintain adequate perfusion of vital organs such as the heart and brain, blood vessels supplying less vital organs such as the kidneys are constricted.22 Renal plasma flow is maximal in the left lateral position.113
During the second half of pregnancy, the supine and upright positions are associated with a reduction in GFR and in urine output.113 As a result, pregnant women excrete water poorly and have a reduced urine volume when lying supine and, to a lesser extent, when upright.113 For example, renal excretion of a water load while in the supine position may be decreased by 40% in late pregnancy. Water excretion is enhanced by the lateral recumbent position. However, this position interferes with the ability of the woman to concentrate urine, possibly because of mobilization of fluid from the lower extremities with increased intravascular volume and subsequent suppression of AVP.
Renal handling of sodium is also affected by postural changes. Moving from a lateral recumbent to a supine or sitting position is associated with sodium retention and, in some cases, with an increase in plasma renin and aldosterone levels.163 Sodium excretion may be decreased in the supine and upright positions. This sodium retention is associated with weight gain and occasional ankle swelling that comes and goes rapidly depending on the woman’s activity patterns and position and does not necessarily indicate pathology. Therefore in evaluating sudden weight gain and edema in the extremities during pregnancy, data regarding recent activity patterns are essential.
Inability to void postpartum
Postpartum urinary retention is defined as the “lack of spontaneous micturition 6 hours after a vaginal delivery or removal of an indwelling catheter.”53 Urinary retention is reported in 0.45% to 17.9% of postpartum women, with an increased risk after first labors, instrument delivery, cesarean birth, epidurals, labor longer than 13 to 14 hours, and perineal lacerations.53 Immediately after delivery, the woman has a hypertonic bladder with an increased capacity and decreased sensation, leading to incomplete emptying. Women should void within 6 to 8 hours of delivery. Some women experience an inability to void after delivery, which is related to the following factors: (1) trauma to the bladder from pressure of the presenting part during labor, with transient loss of bladder sensation; (2) edema of the urethra, vulva, and meatus and spasm of the sphincter from the forces of delivery; (3) decreased intraabdominal pressure immediately postpartum due to continuing distention of the abdominal wall; (4) decreased sensation of the bladder due to regional anesthesia and catheter use; and (5) hematomas of the genital tract.101 Nursing interventions are summarized in Table 11-5.
Risk of urinary tract infection
UTI occurs with increased frequency during pregnancy and is related to anatomic changes in the renal system. Asymptomatic bacteriuria (ASB) occurs in 2% to 10% of pregnant women, which is similar to rates in sexually active nonpregnant women.106,162 However, during pregnancy, 12.5% to 30% of women with untreated ASB develop pyelonephritis.162
Dilation of the urinary tract, along with partial obstruction of the ureters from ureteral compression at the pelvic brim, results in urinary stasis. Large volumes of urine may be sequestered in the ureters and hypotonic bladder during pregnancy. These static pools increase the risk of ASB, especially because the urine may contain glucose, protein, and amino acids, which provide additional substrates for bacterial growth. Edema and hyperemia of the bladder mucosa also increase susceptibility to infection. The static column of urine in the hypoactive ureters also facilitates ascending bacterial migration, increasing the risk of pyelonephritis.106 Women with sickle cell anemia and trait are at higher risk for UTI. UTI has been associated with preterm labor (see Chapters 4 and 13).162
UTIs are also more common during the postpartum period. Factors that increase the risk of UTIs postpartum include pregnancy-induced changes in the bladder (hypotonia, edema, and mucosal hyperemia) that may be aggravated by the trauma of labor and delivery. Decreased bladder sensation from pressure of the fetal head during labor leads to incomplete emptying and urinary stasis, further predisposing to UTI for the first few postpartum weeks. Recommendations to reduce the risk of UTI during pregnancy and the postpartum period are summarized in Table 11-5.
Fluid needs in labor
Fluid needs of women in labor are controversial; the routine use of intravenous (IV) infusions has been questioned and even discontinued in some practices.82,98,119,155 Before use of general anesthesia for delivery, food and fluid intake was maintained during labor. With introduction of general anesthesia, food and fluid were prohibited and IV administration of fluids and glucose became routine to provide fluid and calories to prevent dehydration and ketosis.98,100,155 Routine IV use has continued in many settings even with the decline in use of general anesthesia. There is little documentation supporting either the benefits of this therapy or the risk of oral intake for most women.87,122,143,155 A recent Cochrane review of 5 studies (n=3130) found no benefit or harm of oral fluids in labor and concluded that “there is no justification for the restriction of fluids and food in labor for women at low risk for complications.”14 Current guidelines from professional groups support oral intake during labor in low-risk women.7,8,84
IV infusions may be needed for anesthesia or medication administration and must be carefully monitored. However, there is little evidence to support routine use of IV infusions in otherwise healthy women not receiving these agents. O’Sullivan notes the following: “Intravenous therapy is seldom necessary during the first 12 hours of labor, irrespective of the finding of ketonuria; when it is prescribed, the indication for its use should be clearly documented and fluid balance charts should be meticulously maintained. In fact, the judicious use of oral fluids should mitigate the need for intravenous fluids in many patients.”124,p.39 In addition, it may be harder for the woman to change her position during IV therapy, leading to increased use of the supine position. IV therapy is also linked to increased use of medications because there is an accessible line.82 Volume loading with colloids prior to or during can blunt the hemodynamic alterations with spinal anesthesia in pregnant women.37,140 Crystalloids administered 20 to 30 minutes before spinal anesthesia have been found to have no advantage over administration during the preocedure.150 Complications such as infection, phlebitis, hyponatremia, and fluid overload can occur with IV therapy.87,119
Acute hydration can lead to hypervolemia, with a greater risk in pregnant women who already have expanded body water and plasma volume. The use of hypertonic glucose infusions can lead to elevations in maternal blood glucose, which can in turn result in fetal hyperglycemia and hyperinsulinemia and eventually neonatal hypoglycemia.19,82,98,112,119,121,155 Lower cord blood sodium values and increased neonatal weight loss in the first 48 hours have been reported following the administration of 5% dextrose solutions to laboring women.38
Although rare, the pregnant woman is at increased risk for water intoxication.111 Oxytocin infusions have been associated with water intoxication and maternal and fetal hyponatremia.87,111 The risk of water intoxication is increased when oxytocin is administered with large volumes of hypertonic dextrose solution.87,111 The hypertonic solution pulls fluid into the vascular compartment from the interstitial space, resulting in hemodilution. However, the additional fluid cannot be readily excreted due to the antidiuretic effects of oxytocin. Water intoxication results in electrolyte imbalances such as hyponatremia, which can affect both mother and fetus and in severe cases can lead to seizures and hypoxia.19,87,121 Thus not only must oxytocin infusions be carefully monitored, but maternal electrolyte and fluid status and urine output must also be carefully evaluated for any woman receiving this type of infusion.
Maternal-fetal fluid and electrolyte homeostasis
Fetal fluid and electrolyte balance is dependent on maternal homeostasis and placental function. Because serum osmolarity is similar in the fetus and the mother, changes in maternal or fetal osmolarity will lead to transfer of water from the opposite compartment to achieve homeostasis. Water is continuously exchanged between mother and fetus, with a net flux in favor of the fetus, placenta, and amniotic fluid. Factors that influence the rate and direction of water exchange between the mother and the fetus include maternal and fetal blood flow to and from the placenta, osmotic and hydrostatic pressure gradients across the placenta, and availability of cellular transport mechanisms.91
Fetal balance is affected by any maternal or fetal conditions that alter the supply, demand, and transfer of water. Maternal events such as altered nutrition, electrolyte imbalance, diabetes, hypertension, or the excessive use of diuretics are associated with alterations in fetal fluid and electrolyte status, amniotic fluid volume, and fetal growth.24 For example, fetal urine flow can be increased by volume loading of maternal blood or administration of diuretics, because acute changes in maternal plasma osmolarity induce parallel changes in the fetus due to transplacental movement of fluid, with decreased urine flow and increased AVP.24 In addition, because fetal free water is derived from the mother, net movement of water to the fetus is decreased when maternal osmolarity increases; this leads to decreased fetal urine flow and increased tubular reabsorption of water. The fluid and electrolyte status of the infant reflects maternal balance during labor.151 A reduction in fetal plasma volume with increased fetal osmolarity occurs in most infants during labor. These changes are more pronounced following prolonged labor, with administration of hyperosmolar glucose solutions to the mother, or during fetal hypoxia because fluid is redistributed from the extracellular fluid (ECF) to intracellular fluid (ICF) compartments. Maternal electrolyte imbalances result in similar alterations in the fetus. Administration of hypotonic fluid to the mother can, by increasing the maternal ECF volume, lead to decreases in fetal osmotic pressure and serum sodium and has resulted in maternal and neonatal hyponatremia and an increased risk of neonatal complications.49 If this therapy is used, the fluid and electrolyte status of both mother and newborn infant must be carefully monitored.
Evaluation of renal function during pregnancy
The marked increase in GFR during pregnancy significantly reduces serum blood urea nitrogen (BUN), plasma urea, uric acid, and creatinine by the end of the first trimester. As a result, values that are normal for nonpregnant individuals may actually be elevations for the pregnant woman and reflect pathologic alterations in renal function. Thus it is critical for health care providers to know the normal values for these parameters during pregnancy (see Table 11-2) so that early signs of renal impairment are not missed. In a pregnant woman, serum creatinine decreases in each trimester an average 0.5 mg/dL versus nonpregnant values of 0.80 mg/dL.78 Serum creatinine levels greater than 0.80 mg per 100 mL, plasma urea nitrogen levels greater than 14 mg per 100 mL (6 mmol/L), or plasma BUN and creatinine levels that do not decrease to expected values by mid-gestation may indicate a significant reduction in renal function and require further investigation.40,66,162,163
GFR is best measured by endogenous creatinine clearance (Ccr) during pregnancy as other measures have been demonstrated to underestimate GFR.68,145 The 24-hour Ccr is generally a good index of GFR in both pregnant and nonpregnant women but is less valid in women with severe renal impairment or diminished urine production.91 In the latter case, the amount of creatinine secreted by the proximal tubule can markedly alter urinary creatinine values.40 The Ccr rises approximately 45% by 4 weeks postconception and remains elevated to or near term, when it may begin to fall.40,91
Dilation of the urinary tract with stasis and retention of large volumes of urine can lead to collection errors. Accuracy of clearance measures in pregnancy can be improved by using 24-hour collections to avoid “washout” from diurnal changes in urine flow, ensuring that the woman is well hydrated to ensure a high urine flow rate, discarding the first morning specimen, and having the woman assume a lateral recumbent position for 1 hour before the start and 1 hour before the end of the collection. Dietary intake has to be evaluated in the timing of blood samples during a clearance period in that recent ingestion of cooked meat can increase plasma creatinine levels up to 0.18 mg per 100 mL (1.59 μmol/L). Plasma creatinine levels, used to estimate GFR, are influenced by age, height, weight, and gender. In the pregnant woman, body size and weight may not accurately reflect kidney size.40
Interpretation of diagnostic studies such as ultrasound or IV pyelography in the pregnant woman must be done in light of the normal structural changes.162 Because structural changes in the urinary system may persist for several months after delivery, these alterations also need to be considered when evaluating postpartum renal function. Evaluation of renal function is considered if the GFR during the postpartum period decreases more than 25% to 30% from predelivery values or if the serum creatinine rises above nonpregnant levels.18
Glycosuria
Chemically detectable glycosuria is found in more than 50% of pregnant women.5,28,163 Glucose excretion may be 10 to 100 times greater than the nonpregnant levels of 20 to 100 mg per 24 hours.113 About 70% of pregnant women excrete more than 100 mg of glucose per 24 hours; in up to 50%, glucose excretion is greater than 150 mg per 24 hours.18,40,163 Large day-to-day variation in glucose excretion is reported, with little correlation between excretion rate, plasma glucose levels, and the stage of pregnancy.40,163 Few women with glycosuria have abnormal glucose tolerance test results. Thus glycosuria in pregnancy does not reflect alterations in carbohydrate metabolism but rather alterations in renal function.113,163
The basis for glycosuria in pregnancy is secondary to the increases in GFR and tubular flow rate that exceeds the maximal tubular reabsorptive capacity for glucose (200 to 240 mg/dL).5,78,113 As the GFR increases during pregnancy, renal reabsorptive capacity for glucose also increases but not to the same extent. Thus as the filtered load of glucose increases, more glucose is excreted, leading to glycosuria at normal plasma glucose levels.163 When glucose excretion increases, alterations in reabsorption seem to occur primarily in that portion of the glucose load that escapes reabsorption in the proximal tubules (usually 5% of the filtered glucose) and is normally reabsorbed in the loops of Henle and collecting duct.40,78,113
Glycosuria during pregnancy has not been associated with alterations in perinatal mortality or morbidity or with subsequent development of diabetes or renal disorders.5,28 However, Davison suggests that women with greater than the usual degree of glycosuria during pregnancy may have sustained renal tubular damage from earlier untreated UTIs.40 How this alters renal handling of glucose is unclear. Infection is known to cause a temporary impairment of distal tubular function. In some women, sites for glucose reabsorption may not be completely healed and thus are unable to deal with the stresses imposed by the increased filtered load of glucose during pregnancy.
Because of the high incidence of glycosuria in normal pregnant women, glycosuria is not useful in screening for pregnancy-related glucose intolerance.78 In addition, urinary glucose may not be a reliable indicator of plasma glucose control in pregnant diabetics, thus decreasing the usefulness of urinary glucose concentrations for monitoring these women.40 In the pregnant diabetic, any elevation of blood glucose results in a greater urinary loss of glucose than in the nonpregnant state. Because water and electrolytes are normally lost along with glucose, volume depletion and polydipsia occur sooner in the pregnant than in the nonpregnant diabetic woman (see Chapter 16).
Hypertension and the renal system
The kidneys play a critical role in the regulation of blood pressure; therefore alterations in renal function often lead to hypertension. During the perinatal period, renal disorders associated with hypertension often have a poorer outcome for both mother and infant. Preeclampsia involves specific lesions and functional alterations in the renal system (see Table 9-5). The renal lesion usually seen in preeclampsia is glomerular capillary endotheliosis, which decreases the diameter of the glomerular capillary lumina, resulting in decreased GFR and RPF.68,86 In women with preeclampsia, GFR and RPF are decreased from values seen in healthy pregnant women.86 The renin-angiotensin-aldosterone system is altered in preeclampsia (see p. 362). An exaggerated response to ANTII, which antedates the onset of clinically detectable hypertension, has also been noted in women with preeclampsia.148 Sodium excretion is reduced in these women due to the decreased GFR, increased vascular resistance, decreased RPF (with decreased perfusion of the peritubular capillaries), alterations in plasma and blood volume, and possibly inadequate tubular reabsorptive mechanisms.68,76,77 Further discussion of vascular changes associated with pregnancy, preeclampsia, and the impact of preeclampsia and chronic hypertension can be found in Chapter 9.
Renal disease and pregnancy
Regardless of the specific disorder, as the severity of renal disease and reduction in renal function increase, the ability to conceive and sustain a pregnancy also decreases.39,40,125,162 In general, normotensive women with mild renal disease before pregnancy do well during pregnancy, although the risk of preeclampsia, preterm birth and fetal growth restriction increases, and their renal prognosis is not significantly altered by pregnancy.125,145 Women with moderate to severe disease have a greater risk of worsening renal function, hypertension, preeclampsia, preterm birth, or other pregnancy complications.125,145,162 A normal pregnancy is rare if, before conception, the woman has a plasma creatinine greater than 2.5 mg/dL (220 μmol/L) or a urea nitrogen above 30 mg/dL (10.72 mmol/L).125 Table 11-6 summarizes the effects of pregnancy on chronic renal problems. Management of women with chronic renal problems includes careful monitoring of maternal functional status and signs of increasing severity of the disease and fetal assessment.
Table 11-6
Chronic Renal Disease and Pregnancy
RENAL DISEASE | EFFECTS |
Chronic glomerulonephritis and focal glomerular sclerosis (FGS) | Incidence of high blood pressure late in gestation is increased, but there is usually no adverse effect if renal function is preserved and hypertension is absent before gestation. Some disagree, believing coagulation changes in pregnancy exacerbate disease, especially immunoglobulin A (IgA) nephropathy, membranoproliferative glomerulonephritis, and FGS. |
IgA nephropathy | Some cite risks of sudden escalating or uncontrolled hypertension and renal deterioration. Most note good outcome when renal function is preserved. |
Chronic pyelonephritis (infectious tubular disease) | Bacteriuria occurs in pregnancy and may lead to exacerbation. |
Reflux nephropathy | In the past, some emphasized risks of sudden escalating hypertension and worsening of renal function. Consensus now is that results are satisfactory when preconception function is only mildly affected and hypertension is absent. Vigilant screening for urinary tract infection is necessary. |
Urolithiasis | Ureteral dilation and stasis do not seem to affect natural history, but infections can be more frequent. Stents have been successfully placed, and sonographically controlled ureterostomy has been performed during gestation. |
Polycystic kidney disease | Functional impairment and hypertension usually minimal in childbearing years. |
Diabetic nephropathy | There are no adverse effects of the renal lesion. Frequencies of infection, edema, and preeclampsia increase. |
Systemic lupus erythematosus | Prognosis is most favorable if disease is in remission 6 or more months prior to conception. Some authorities increase steroid dosage in immediate postpartum period. |
Periarteritis nodosa | Fetal prognosis is poor. Associated with maternal death. Therapeutic abortion should be considered. |
Scleroderma | For onset during pregnancy, there can be rapid overall deterioration. Reactivation of quiescent scleroderma can occur during pregnancy and after delivery. |
Previous urologic surgery | Depending on original reason for surgery, there may be other malformations of urogenital tract. Urinary tract infection is common during pregnancy, and renal function may undergo reversible decrease. No significant obstructive problem but cesarean section may be necessary in case of abnormal presentation or to avoid disruption of the continence mechanisms if artificial sphincters or neourethras are present. |
After nephrectomy, solitary and pelvic kidneys | Pregnancy is well tolerated. Condition may be associated with other malformations of the urogenital tract. Dystocia rarely occurs with a pelvic kidney. |
From Williams, D.J. & Davison, J.M. (2009). Renal disorders. In R.K. Creasy, et al. (Eds.), Creasy & Resnik’s Maternal-fetal medicine: Principles and practice (6th ed.). Philadelphia: Saunders, p. 916.
Pregnancy following renal transplantation
Having a transplant improves fertility within 1 to 12 months in women who have been on dialysis.80,125,162 Many women have conceived following renal transplantation. Overall 71% to 76% of pregnancies result in a live birth.35 Of those women who carried the pregnancy beyond the first trimester, more than 90% have had a successful pregnancy outcome, although pregnancy complications such as hypertension, preterm birth, preeclampsia, and fetal growth restriction were increased.35,46,79,80,89,125,162 The transplanted kidney undergoes the usual renal changes seen in pregnancy. Renal hemodynamics often improve with pregnancy, but some women have impairment of renal function during pregnancy that may persist.35,66 However, a recent review did not find any negative effects of pregnancy on either maternal survival or the transplanted kidney.79 Pregnancy is generally not recommended for at least 1 year following transplantation in stable women to ensure that the transplant is successful and able to function under the increased demands of pregnancy.80,104 Optimal immunosuppressant therapy during pregnancy is unknown but the woman may need to be switched to safer drugs as a few agents have been found to be associated with an increased risk of fetal anomalies.79,125 Long-term follow-up data are still sparse, especially for the newer drugs.162
Summary
The renal system is critical to maintenance of fluid and electrolyte homeostasis within the body. Relatively small changes in renal function can significantly alter this homeostasis, leading to a variety of volume and electrolyte disorders. Renal function and many related laboratory parameters are altered significantly during pregnancy to levels that would be of concern in a nonpregnant individual. In most cases the pregnant woman readily adapts to these changes and establishes a new equilibrium for volume and electrolyte homeostasis. At this new equilibrium, she responds to alterations in fluid and electrolyte intake in a manner similar to that of a nonpregnant individual. Pathophysiologic conditions that alter renal function or volume homeostasis affect the health of both the pregnant woman and her infant. Monitoring and health counseling related to fluid and electrolyte status are essential during pregnancy. Clinical recommendations for nurses working with pregnant women based on alterations in the renal system and fluid and electrolyte balance are summarized in Table 11-7.
Table 11-7
Recognize the usual changes in the renal system during pregnancy and postpartum (pp. 356-365 and Table 11-1).
Recognize the usual values for renal function tests and patterns of change during pregnancy and postpartum (pp. 359-360, 368 and Table 11-2).
Recognize that individual laboratory values must be evaluated in light of clinical findings and previous values (pp. 359-360, 368).
Teach women to recognize and reduce the risk of urinary tract infection during pregnancy and postpartum (pp. 366-367).
Recognize the effects of altered renal function on pharmacokinetics of drug elimination by glomerular filtration (pp. 359-360, 364 and Chapter 7).
Monitor and evaluate maternal responses to drugs for evidence of subtherapeutic doses (pp. 359-360, 364 and Chapter 7).
Assess maternal nutritional status in relation to calcium and water-soluble vitamins and provide nutritional counseling (p. 360 and Chapters 12 and 17).
Know the influences of position on renal function (pp. 365-366).
Counsel women regarding appropriate positions and activity patterns (pp. 365-366).
Assess activity patterns and position in evaluating changes in weight gain and edema (p. 365).
Monitor fluid and electrolyte status and renal function following use of general anesthetics (pp. 364, 367).
Monitor oxytocin and intravenous infusions during labor and delivery to avoid overload (pp. 364-367).
Use fluids, ice chips, and other alternatives to use of intravenous infusions with women with uncomplicated labors (p. 367).
Know the benefits and risks for the mother, fetus, and neonate of different types of intravenous fluids (pp. 367-368).
Avoid use of hypertonic solutions during labor (pp. 367-368).
Know indications for intrapartum intravenous therapy (pp. 367-368).
Monitor maternal fluid and electrolyte status and urine output (especially in women receiving an oxytocin infusion or with preeclampsia or compromised renal function) during labor for development of water intoxication (pp. 364, 367-368).
Teach women the common experiences associated with changes in the renal system (urinary frequency, dependent edema, nocturia) and implement appropriate interventions (pp. 365-367 and Table 11-5).
Monitor women with dependent edema for varicosities and thromboembolism (p. 365 and Chapter 8).
Know usual values and monitor for glycosuria and proteinuria in the pregnant woman (pp. 360, 368-369).
Monitor fluid and electrolyte status of women with preeclampsia, chronic hypertension, and diabetes, recognizing that alterations may occur more rapidly than in other pregnant women (pp. 368-369).
Monitor fluid and electrolyte status, renal function, amniotic fluid volume, and fetal growth in women on diuretics (pp. 367-368).
Counsel women with renal disorders regarding the impact of their disorder on pregnancy and of pregnancy on their disorder (p. 369 and Table 11-6).
Monitor women with chronic renal problems for signs of initiation of preterm labor, fetal growth restriction, hypertension, and alterations in maternal renal function (p. 369 and Table 11-6).
Evaluate bladder function and voiding postpartum (pp. 366, 364-365).
Implement interventions to encourage postpartum voiding (p. 366, Table 11-5).
Observe for signs of pulmonary edema and congestive heart failure post birth in women with preeclampsia and impaired renal function (p. 364).
Recognize that structural changes in the urinary system may persist for 6 months or more following birth (pp. 364-365).