Renal system and fluid and electrolyte homeostasis
The kidneys are critical organs in maintaining body homeostasis by regulation of water and electrolyte balance, excretion of metabolic waste products and foreign substances, regulation of vitamin D activity and erythrocyte production (via erythropoietin), and gluconeogenesis.161 The kidneys also have an important role in control of arterial blood pressure through the renin-angiotensin system and regulation of sodium balance. This chapter examines the alterations in basic renal processes and regulation of fluids and electrolytes observed in the pregnant woman, fetus, and neonate, and discusses the implications of these changes for clinical practice. Basic renal processes are summarized in Figure 11-1 and Box 11-1 on page 358.
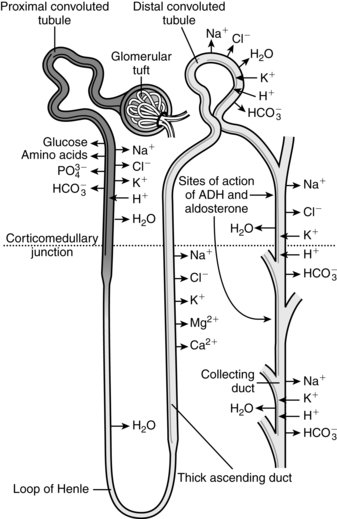
Maternal physiologic adaptations
Antepartum period
The renal system must handle the effects of increased maternal intravascular and extracellular volume and metabolic waste products as well as serve as the primary excretory organ for fetal wastes. Changes in the renal system are related to hormonal effects (particularly the influence of progesterone on smooth muscle), pressure from the enlarging uterus, effects of position and activity, and alterations in the cardiovascular system and vasoactive substances. Cardiovascular system changes that interact with alterations in renal hemodynamics include increased cardiac output, increased blood and plasma volume, and alterations in the venous system and plasma proteins (see Chapters 8 and 9). The predominant structural change in the renal system during pregnancy is dilation of the renal pelvis and ureters; functional changes include alterations in hemodynamics, glomerular filtration, and tubular handling of certain substances. Changes in fluid and electrolyte homeostasis result from changes in renal handling of water and sodium and alterations in the renin-angiotensin system. Table 11-1 summarizes changes in the renal system during pregnancy and their clinical implications. Even with these changes, renal reserve is maintained during pregnancy, so that the pregnant woman has the capacity for further vasodilation and filtration above baseline if needed.
Table 11-1
Changes in the Renal System During Pregnancy
PARAMETER | ALTERATION | SIGNIFICANCE |
Renal calyces, pelvis, and ureters |
Structural changes
Pregnancy is characterized by physiologic hydroureter and hydronephrosis. Significant dilation of the renal calyces, pelvis, and ureters beginning as early as the seventh week, is seen in up to 80% of women.21,53,78,113,125 The mean kidney length increases by approximately 1 cm due to the increased renal blood flow (RBF), renal vascular and volume, and renal hypertrophy.66,113 Renal volume increases by 30%.78,113 The diameter of the ureteral lumen increases with hypertonicity and hypomotility of the ureteral musculature. Hypomotility and reduced peristaltic movements of the ureters may be mediated by prostaglandin E2 (PGE2).21,54
The ureters elongate and become more tortuous, especially during the last half of pregnancy as they are laterally displaced by the growing uterus.21 These changes are seen within the renal pelvis and the upper portion of the ureters to the pelvic brim. The portion of the ureters below the pelvic brim (linea terminalis) is usually not enlarged. The ureters may contain as much as 300 mL of urine by the third trimester.54 This reservoir of urine leads to stasis and can interfere with evaluation of glomerular filtration rate (GFR), tubular function, and influence the accuracy of 24-hour urine collections.113,125 The stasis increases the risk of ascending urinary tract infection (UTI), nephrolithiasis, and pyelonephrosis.53,68,70,113,125
The etiology of physiologic hydroureter is unclear. Dilation begins before the uterus reaches the pelvic brim, so initial changes are probably hormonally mediated. Hormonal influences, particularly progesterone, may induce hypertrophy of the longitudinal smooth muscles surrounding distal portions of the ureters and hyperplasia of periurethral connective tissue.21,54,113 This may lead to a temporary stenosis and mild dilation of the upper portion of the ureters. These findings are similar to those seen in women on oral contraceptives and in postmenopausal women given estrogen and progesterone.54
The major contributing factor to hydronephrosis in later pregnancy is probably external compression of the ureters at the pelvic brim. As pregnancy progresses, the ureters are compressed at the pelvic brim by the iliac arteries, enlarging ovarian vein complexes, and the growing uterus, leading to further marked dilation and urinary stasis.51 Dilation is more prominent in the primipara, whose firmer abdominal wall may increase resistance and pressure on the ureters.21
In most women, the right ureter is dilated to a greater extent than the left.51,78,113 These differences become more prominent after midgestation.51 The right ureter makes a right-angle turn as it crosses the iliac and ovarian veins at the pelvic brim; the turn of the left ureter is less acute, and it parallels rather than crosses the left ovarian vein. The iliac vessels are more rigid on the right than on the left, thus further compressing the ureters. Compression is maximal by around 30 weeks’ gestation, with no further significant changes to term. The sigmoid colon contributes to dextroversion of the uterus and may increase ureteral compression on the contralateral side during the last trimester.21,54,78,113 The position of the fetus does not seem to influence ureteral dilation. The site of placental attachment may increase venous flow on that side with subsequent compression of the ureters by the dilated vessels.54
Bladder tone decreases as a result of the effects of progesterone on smooth muscle. Bladder capacity doubles by term. The bladder becomes displaced anteriorly and superiorly by the end of the second trimester. Under the influence of estrogen, the trigone undergoes hyperplasia with hypertrophy of the bladder musculature. The bladder mucosa becomes hyperemic with increased size and tortuosity of the blood vessels. The mucosa becomes more edematous and vulnerable to trauma or infection after engagement of the presenting part.54
The baseline intravesical pressure doubles due to the enlarged uterus.106 The decreased bladder tone and flaccidity may lead to incompetence of the vesicoureteral valve and reflux of urine. Vesicoureteral reflux is seen in up to 3.5% of pregnant women, especially in the third trimester.106 Predisposing factors for this reflux include hypertrophy and hyperplasia of the ureteral wall, increased elasticity of the ureters, increased bladder pressure, and decreased peristalsis in the distal ureter.106 Alterations in bladder placement by the growing uterus stretch the trigone and displace the intravesical portion of the ureters laterally. This shortens the terminal ureter, decreasing intravesical pressure. If intravesical pressure subsequently increases with micturition, urine regurgitates into the ureters.54
Urodynamic changes
Urine output increases from a mean of 1475 to 1919 mL per 24 hours primarily because of changes in sodium excretion.106 Mean flow rate decreases in the second and third trimesters with an increase in flow time and time to maximal flow throughout pregnancy.106 The number of voids per day and mean daily urine output increase throughout gestation.152 Studies of changes in bladder capacity during pregnancy have reported variable findings. Increased bladder capacity due to the decreased tone has been reported by some investigators, especially in primiparas.10 Others have found a mean bladder capacity similar to that of the nonpregnant state in the first two trimesters, but decreased capacity in the third trimester due to elevation of the trigone and the size of the presenting part.142 Another study reported that the pregnant woman’s bladder has a larger capacity with a lower pressure per volume.134 In nonpregnant women the first urge to void was at a bladder capacity of 150 to 200 mL and maximum at 450 to 550 mL with an intravesicular pressure of 20 cmH2O (1.96 kPa); in pregnant women the first urge to void was at a bladder capacity of 250 to 400 mL (intravesical pressure of 4 to 8 cmH2O [0.39 to 0.78 kPa]) and often did not reach maximum until 1000 to 1200 mL (intravesicular pressure of 12 to 15 cmH2O [1.17 to 1.47 kPa]).134
Changes in renal hemodynamics
Significant hemodynamic changes occur within the kidneys beginning early in pregnancy in conjunction with systemic vasodilation (see Chapter 9). RBF and GFR increase. Increases in these parameters, although not to the degree seen in pregnancy, also occur during the luteal phase of the menstrual cycle.78
RBF increases up to 50% to 80% by the mid–second trimester and then slowly decreases to term.91,113 The higher values are seen in the left lateral recumbent position.33 This change is accompanied by increased glomerular filtration rate (GFR), decreased renal vascular resistance (RVR), and activation of the renin-angiotensin-aldosterone system. Renal hemodynamic changes begin before significant expansion of plasma volume and are thought to be primarily related to the decrease in systemic vascular resistance (SVR), which may stimulate decreased RVR leading to increased RBF and GFR, and by sodium retention. These changes may be mediated by nitric oxide (NO), prostacyclin (PGI2), atrial natriuretic factor (ANF), progesterone, endothelin B, and relaxin, possibly via their effects on GFR. Increased flow is enhanced by vasodilation of preglomerular and postglomerular capillaries.33,78,113,125 Another measure of renal hemodynamics is the effective renal plasma flow (ERPF), which increases 80% by midpregnancy.40,113,153 ERPF then gradually decreases during the third trimester to values 50% greater than nonpregnant values.66,113
Changes in glomerular filtration
The GFR increases 40% to 60% during pregnancy.29,34,66,91,113 The rise begins as early as 6 weeks and precedes plasma volume expansion and cardiac output (see Chapter 9).29,91 Changes in GFR are detectable 3 to 4 weeks after conception, with 25% of the increase occurring by 2 weeks postconception, peak at 9 to 16 weeks, then remain relatively stable to near term.113 Values for GFR in pregnancy, as measured by creatinine clearance, average 110 to 150 mL/min.113 Differences in reported values for RBF and GFR during pregnancy vary with the method of measurement. The increased GFR is related to the increased glomerular blood flow (and glomerular capillary hydrostatic pressure) and decreased colloid osmotic (plasma oncotic) pressure due to a reduction in the concentration of plasma proteins.71,91 Failure of the GFR to increase early in pregnancy has been associated with pregnancy loss.163
The increases in renal plasma flow (RPF) and GFR parallel each other, although RPF changes are slightly greater than GFR changes. This alters the filtration fraction (GFR/RPF), the portion of the RBF that is filtered.70,78,113 The filtration fraction is decreased in the first half of gestation and increased in late gestation as ERPF decreases.78,91 As a result, renal excretion of amino acids, glucose, protein, electrolytes, and vitamins increases, whereas serum urea, creatinine, blood urea nitrogen (BUN), and uric acid levels decrease (Table 11-2).
Table 11-2
Changes in Laboratory Values Associated with Renal Function during Pregnancy
VARIABLE | NONPREGNANT VALUES | VALUES DURING PREGNANCY | VALUES REQUIRING FURTHER INVESTIGATION |
Creatinine clearance | 85-120 mL/min | 110-150 mL/min | |
Plasma creatinine | 0.65 ± 0.14 mg/dL | 0.46 ± 0.13 mg/dL | >0.80 mg/dL |
Blood urea nitrogen | 13 ± 3 mg/dL | 8.7 ± 1.5 mg/dL | >14 mg/dL |
Urinary protein | <100-150 mg/24 hours | <250-300 mg/24 hours | >300 mg/24 hours |
Urinary glucose | 20-100 mg/24 hours | >100 mg/24 hours (up to 10 g/24 hours) | |
Plasma urate | 4-6 mg/dL | 2.5-4 mg/dL | >5.8 mg/dL |
Urinary amino acids | Up to 2 g/24 hours | >2 g/24 hours |
The causes of the increased GFR during pregnancy are still not completely clear, but the primary factor is the increased RPF and decreased renal vascular resistance.32,91,144 These changes are stimulated by relaxin (which up-regulates vascular gelatinase activity and leads to activation of endothelin receptors causing vasodilation), increased GFR, and relaxation of the small renal arteries. This process is mediated by NO, which is a potent renal vasodilator.41,91 Other factors that may play a role are prostacyclin, ANF, human placental lactogen, and human chorionic gonadotropin, which stimulates relaxin secretion.32,33,41
During pregnancy, 24-hour urine volumes are higher due to the increased GFR. The degree to which renal handling of substances is altered during pregnancy depends on the renal process involved (see Figure 11-1). For example, because urea and creatinine are processed only by glomerular filtration, the increased GFR leads to a significant decrease in serum urea and creatinine levels.91,113 The increased GFR also alters renal excretion of drugs (see Chapter 7).9
Alterations in tubular function
Renal glucose excretion increases soon after conception and remains high to term. Urinary glucose values may be 10- to 100-fold greater than the nonpregnant values of 20 to 100 mg per 24 hours due to alterations in reabsorption of glucose in the loop of Henle and collecting ducts.40,113 Glycosuria is more common during pregnancy and can vary from day to day and within any 24-hour period. Glycosuria is discussed in Evaluation of Renal Function during Pregnancy.
Excretion of amino acids, urea, and protein increases in pregnancy. Protein excretion rises from less than 100 mg per 24 hours to up to 250 to 300 mg per 24 hours, with marked day-to-day variation.40,66 The primary amino acids whose excretion is markedly increased throughout pregnancy are alanine, glycine, histidine, serine, and threonine; excretion of cysteine, leucine, lysine, phenylalanine, taurine, and tyrosine increases in early pregnancy, but decreases later.113 Increased urea clearance leads to decreased plasma urea nitrogen levels by 8 to 10 weeks.40 Plasma urea levels may be only 63% of nonpregnant values by the third trimester.
Proteinuria occurs more frequently during pregnancy with increases in both total protein and urinary albumin.78 The filtered load of amino acids during pregnancy may exceed tubular reabsorptive capacity with small amounts of protein lost in the urine. Values of 1+ protein on dipsticks are common and do not necessarily indicate the presence of glomerular pathology or preeclampsia.5,160 Urinary protein excretion during pregnancy is not considered abnormal until values exceed 300 mg per 24 hours.68,78,113,125 Protein excretion does not correlate with the severity of renal disease, and increased protein excretion in a pregnant woman with known renal disease does not necessarily indicate progression of the disease.40 However, proteinuria associated with hypertension in the pregnant woman is associated with a greater risk of an adverse pregnancy outcome.68,162
Uric acid is normally handled by filtration, secretion, and reabsorption (see Box 11-1 on 358), so less than 10% of the filtered load appears in urine. During pregnancy, filtration of uric acid increases up to 30% in the first 16 weeks, and net reabsorption is decreased and secretion enhanced.163 As a result, serum uric acid levels decrease up to 25% to 35%, beginning as early as 8 weeks, to a nadir of 2 to 3 mg/dL by 24 weeks.78,113 Levels gradually increase toward nonpregnant values after that point as tubular reabsorption of uric acid increases. This increase may also be a result of the rise in RPF that alters the filtration fraction at this stage of gestation.40,113 In women with preeclampsia, uric acid clearance is reduced with higher serum levels.78
Potassium excretion is decreased, with retention of an additional 300 to 350 mEq that is thought to be due to increased proximal tubular reabsorption.91,113 Serum potassium levels do not rise, because the additional potassium is used for maternal tissues and by the fetus. The mechanisms for increased potassium reabsorption in pregnancy are not well documented. These changes occur despite the increase in aldosterone that normally would increase urinary potassium loss. Therefore the altered potassium excretion may be due to antagonistic action of progesterone on renal tubular actions of aldosterone.91
Renal acid-base balance is altered to compensate for the respiratory alkalosis that develops secondary to an increased loss of carbon dioxide from changes in ventilation during pregnancy (see Chapter 10). The respiratory alkalosis is compensated for by increased renal loss of bicarbonate. This is accomplished by renal retention of H+ ions and a decrease in serum bicarbonate. As a result, serum bicarbonate levels fall 4 to 5 mEq/L (mmol/L) during the first trimester to 20 to 22 mEq/L (mmol/L).66,125 This change may limit buffering capacity in the pregnant woman.78
Urinary calcium excretion is increased, possibly due to the increased GFR, and serum calcium and phosphorus levels decrease. This is balanced by increased intestinal absorption of calcium, so serum ionic calcium levels remain stable (see Chapter 17).113 To maintain homeostasis and meet fetal demands, women need 1200 mg of calcium per day in their diet. Excretion of water-soluble vitamins also increases, so maternal diet must be evaluated to ensure adequate supplies of vitamins B1, B2, B6, and C; folate; and niacin (see Chapter 12).
Fluid and electrolyte homeostasis
Sodium homeostasis.
The filtered load of sodium increases up to 50% as a result of the increased GFR. The nonpregnant woman filters approximately 20,000 mEq of sodium per day; the pregnant woman filters 30,000.41,113 To prevent excessive urinary sodium loss, tubular reabsorption of sodium also increases so that 99% of the filtered sodium is reabsorbed. As a result, there is a net retention of 900 to 950 mg (2 to 6 mEq/day) of sodium during pregnancy.40,41,78,113 Sodium retention occurs gradually with an increase in late pregnancy.41 Much of the sodium is used by the fetus and placenta; the rest is distributed in maternal blood and extracellular fluid (ECF) (Table 11-3).163
Table 11-3
Storage of Sodium during Pregnancy
STORAGE SITE | SODIUM (MEQ [MMOL/L]) |
Fetus | 290 |
Edema fluid | 240 |
Plasma | 140 |
Amniotic fluid | 100 |
Uterus | 80 |
Placenta | 57 |
Breasts | 35 |
Red cells | 5 |
Total | 947 |
From Sullivan, C.A. & Martin, J.N. (1994). Sodium and pregnancy. Clin Obstet Gynecol 37, 558.