Objectives
• List three etiologies of acute kidney injury.
• Identify the priorities of nursing management in acute kidney injury.
• Discuss the differences between hemodialysis and continuous renal replacement therapy.
• Explain the differences between a living-donor and a deceased-donor kidney transplant.
• Describe the priorities of nursing management for the kidney transplant recipient.
Be sure to check out the bonus material, including free self-assessment exercises, on the Evolve web site at http://evolve.elsevier.com/Urden/priorities/.
Acute Kidney Injury
Acute kidney injury (AKI) describes the spectrum of acute-onset kidney failure that can occur with critical illness; it replaces the traditional terms acute renal failure (ARF)1 and acute tubular necrosis (ATN). Severe AKI is characterized by a sudden decline in glomerular filtration rate (GFR), with subsequent retention of products in the blood that are normally excreted by the kidneys; this disrupts electrolyte balance, acid-base homeostasis, and fluid volume equilibrium.1,2 A transition to greater use of the word kidney rather than renal reflects a trend in the nephrology literature that emphasizes the vulnerability of the kidney during critical illness.
Critical Illness and Acute Kidney Injury
The estimated incidence of AKI is between 2000 and 3000 cases per 1 million people per year.3 Researchers estimate that AKI accounts for 1% of acute hospital admissions and complicates more than 7% of inpatient episodes, especially for older individuals and those with preexistent chronic kidney disease.2
Critical care patients with AKI have a longer length of hospital stay and more complications.3 After AKI has occurred in the critically ill patient, the risk of death rises dramatically.3,4 The mortality rate ranges from 38% to 80%.5 One of the reasons for the high mortality rate is that critical care patients often have coexisting nonrenal health problems that increase their susceptibility to the development of AKI. High-risk conditions include heart failure, shock, respiratory failure, and sepsis, and this situation has altered the spectrum of AKI.4,5 An observational study that examined the incidence and course of severe AKI that resulted in ARF in six academic medical centers in the United States found that AKI was accompanied by multiorgan failure in most patients, even those who did not require dialysis.4 In this study of 618 patients with ARF, 64% of patients required dialysis, the in-hospital mortality rate was 37%, and the permanent loss of kidney function or death was 50%.4 The clinicians’ conclusion is that death of the critically ill patient with AKI-related ARF is related to the severity of coexisting nonrenal diseases.4 Mortality rates exceeded 50% when four or more body systems had failed.4
Cohort studies suggest that the incidence of AKI is increasing, whereas the mortality rate is declining.6 Patients with AKI often have associated multiple organ dysfunction syndrome (MODS) and have more complex illnesses and comorbidities than patients 40 years ago, and more critical care patients are receiving dialysis therapies in the critical care unit.
Typically, a patient is not admitted to the critical care unit with a diagnosis of AKI alone; there is always coexisting hemodynamic, cardiac, pulmonary, or neurologic compromise. Many individuals come into the hospital with underlying changes in kidney function, such as an elevated serum creatinine (Cr) level, although the patient is not symptomatic and is often unaware of the compromised kidney.7 The lack of kidney reserve places the patient at increased risk for AKI if complications occur in any of the other major organ systems. As a result, the picture of AKI in the modern critical care unit has changed to encompass patients with kidney injury who also have multisystem dysfunction that complicates their clinical course.3
Definitions of Acute Kidney Injury and Acute Renal Failure
One of the challenges of estimating the incidence of AKI or ARF in the critical care unit has been the wide variation in definitions that have been used.8 Measurement of kidney function is necessarily indirect, and the diagnosis of AKI is predominantly derived from changes in urine output (UO) and serum creatine level, with the assumption that changes in these values reflect changes in the GFR.8 Urine output is sometimes a problematic measure to use because diuretics artificially increase the urine output but do not alter the course of kidney failure. The clinical insult may have direct effects on the kidney, such as the inflammation associated with sepsis, which accounts for 50% of the AKI seen in critical care units.9
RIFLE Criteria
The risk of development of AKI in critically ill patients has been classified by a multinational group of nephrologists.10 The classification uses the acronym RIFLE (risk, injury, failure, loss, and end-stage kidney disease [ESKD]).11 The RIFLE system classifies AKI into three categories of increasing severity (R, I, F) and two outcome criteria (L, E) based on GFR status reflected by the change in urine output or loss of kidney function11 (Table 20-1). If AKI is superimposed on a kidney that is already compromised, the term chronic is added to the RIFLE criteria to denote the cause as acute-on-chronic kidney failure.11
TABLE 20-1
RIFLE CRITERIA FOR ACUTE KIDNEY DYSFUNCTION
RIFLE CRITERIA | SERUM CREATININE CRITERIA* | URINE OUTPUT CRITERIA |
Risk | Serum Cr increased 1.5 times above normal or Serum Cr increase ≥0.3 mg/dL |
UO <0.5 mL/kg/hr for 6 hr |
Injury | Serum Cr increased 2 times above normal | UO <0.5 mL/kg/hr for 12 hr |
Failure | Serum Cr increased 3 times above normal or Serum Cr ≥4 mg/dL or Serum Cr acute rise ≥0.5 mg/dL |
UO <0.3 mL/kg/hr for 24 hr or anuria for 12 hr (oliguria) |
Loss | Persistent AKI = complete loss of kidney function for >4 wk | |
ESKD | End-stage kidney disease |
*All serum creatinine references are based on changes from baseline.
Data from Kellum JA, Bellomo R, Ronco C: Definition and classification of acute kidney injury, Nephron Clin Pract 109(4):c182, 2008.
Acute Kidney Injury Network Criteria
The Acute Kidney Injury Network (AKIN) criteria are listed in Box 20-1. These criteria are similar to those proposed by the RIFLE group, and both groups intend to make the point that in the acutely ill patient, small changes in the serum creatinine level and urine output may signal important declines in the GFR and kidney function. A conceptual model that combines the features of the RIFLE criteria and AKIN criteria are shown in Figure 20-1.11
Etiology of Acute Kidney Injury
Previously, AKI was predominantly classified by the location of the insult relative to the kidney: prerenal (before), intrarenal (within), and postrenal (after) (Box 20-2). This remains a useful way to imagine the relationship between anatomy and functional insults to the kidney when one is learning about AKI, although deleterious effects on the kidney are unlikely to be restricted to only one anatomical section at a time.11
Prerenal Acute Kidney Injury
Any condition that decreases blood flow, blood pressure (BP), or kidney perfusion before arterial blood in the renal artery enters the kidney may be anatomically described as prerenal AKI. When arterial hypoperfusion due to low cardiac output, hemorrhage, vasodilation, thrombosis, or other cause reduces the blood flow to the kidney, glomerular filtration and, consequently, urine output decrease (see Box 20-2). This is a major reason that the critical care nurse monitors the urine output on an hourly basis. Initially, in prerenal states, the integrity of the kidney’s nephron structure and function may be preserved. If normal perfusion and cardiac output are restored quickly, the kidney will not suffer permanent injury. However, if the prerenal insult is not corrected, the GFR will decline, the blood urea nitrogen (BUN) concentration will rise (prerenal azotemia),9 oliguria will develop, and the patient will be at risk for significant kidney damage. Oliguria (urine output <400 mL/day) is a classic finding in AKI.5 Prerenal AKI is seen frequently in the critically ill. In a study of hospitalized older patients with kidney failure, prerenal AKI occurred in 58%.5 This compares with rates of 34% for intrarenal AKI and 8% for postrenal AKI in the same study.5
Intrarenal Acute Kidney Injury
Any condition that produces an ischemic or toxic insult directly at the site of the nephron places the patient at risk for development of intrarenal AKI (see Box 20-2).
Ischemic damage to the kidney can also damage internal structures from prolonged hypotension or low cardiac output. In the multicenter study of critical care patients with AKI by Mehta and colleagues,4 50% of the patients had ischemic injury, which was largely precipitated by hypotension (20%) and sepsis (19%).
Toxins that damage kidney tubular endothelium include medications that are administered to treat a coexisting condition12 as well as radiopaque (contrast) dye administered during an interventional or diagnostic radiological study. Complications from the contrast dye cause AKI in 9% to 14% of patients.4 In affected patients, the serum creatinine levels typically begin to rise 48 to 72 hours after the study, peak at 3 to 5 days, and return to baseline within another 3 to 5 days.13 Kidney dysfunction can persist up to 3 weeks after the procedure. Although patients with normal kidney function are not considered to be at risk, those with elevated serum creatinine levels, diabetes, or microvascular disease are highly vulnerable.13
Postrenal Acute Kidney Injury
Any obstruction that hinders the flow of urine from beyond the kidney through the remainder of the urinary tract may lead to postrenal AKI. This is not a common cause of kidney failure in the critically ill. When monitoring of the urine output reveals a sudden decrease in the patient’s urine output from the urinary catheter, a blockage may be responsible. Sudden development of anuria (urine output <100 mL/24 hr) should prompt verification that the urinary catheter is not occluded.
Azotemia
The term azotemia is used to describe an acute rise in the BUN level.9 Uremia is another term used to describe an elevated BUN value.
Phases of Acute Kidney Injury
AKI can result from nephrotoxic or ischemic injury that damages the kidney tubular epithelium. In severe cases, the injury extends to the collagen of the basement membrane (Box 20-3).
Onset Phase
The onset (initiating) phase of AKI is the period from the initial insult until cell injury occurs. Ischemic injury is evolving during this time. The GFR is decreased because of impaired blood flow to the kidney and decreased glomerular ultrafiltration pressure. The GFR decrease disrupts the integrity of the tubular epithelium. This phase lasts hours to days, depending on the severity of the injury. If treatment is initiated during this time, irreversible damage may be alleviated. A longer course of recovery reflects the presence of more extensive tubular injury.
Oliguric or Anuric Phase
The oliguric or anuric phase, the second phase of AKI, lasts 5 to 8 days in the nonoliguric patient and 10 to 16 days in the oliguric patient.14 The accumulation of necrotic cellular debris in the tubular space blocks the flow of urine and causes damage to the tubular wall and basement membranes. Backleak occurs because damage in the tubular wall causes the glomerular filtrate to flow passively into the kidney tissue rather than to be passed out as urine through the ureters and bladder. Oliguria is encountered more often in ischemic damage and is a sign that the damage is extensive and severe. During the oliguric or anuric phase, the GFR is greatly reduced, leading to increased levels of BUN (azotemia), elevated serum creatinine values, electrolyte abnormalities (hyperkalemia, hyperphosphatemia, hypocalcemia), and metabolic acidosis.
Diuretic Phase
The third phase, the diuretic phase of AKI, may last 7 to 14 days and is characterized by an increase in the GFR. During the diuretic phase, the tubular obstruction has passed, but edema and scarring remain. In this situation, the GFR returns and the kidneys can clear fluid volume but not solutes.
Recovery Phase
Critically ill patients with AKI have a high mortality on long-term follow-up.15 Kidney function may return to normal, or near normal, with a GFR that is 70% to 80% of normal within 1 to 2 years.16 However, if significant kidney parenchymal damage has occurred, BUN and creatinine levels may never return to normal. Of the patients who survive AKI, approximately 62% eventually recover normal kidney function, 33% have residual kidney damage, and at least 5% require long-term hemodialysis.16
Laboratory Assessment
After acute kidney disease is suspected, the presence or degree or AKI is assessed using urinalysis and blood analysis. Table 20-2 lists the initial urinalysis findings for patients with AKI. Most serum electrolyte values become increasingly elevated as AKI develops (Tables 20-3 and 20-4). Normal and abnormal urinalysis findings are summarized in Chapter 19.
TABLE 20-2
INITIAL URINE LABORATORY ANALYSIS FINDINGS IN ACUTE KIDNEY INJURY*
ASSESSMENT | PRERENAL† | INTRARENAL‡ | POSTRENAL§ |
Urine volume | Normal | Oliguria or nonoliguria | Oliguria to anuria |
Urine specific gravity | >1.020 | 1.010 | 1.000-1.010 |
Urine osmolality (mOsm/kg) | >350 | <300 | 300-400 |
Urine sodium (mEq/L) | <20 | >30 | 20-40 |
FENa (%) | <1 | >2-3 | 1-3 |
BUN/Cr ratio | 20 : 1 | Ischemic: 20 : 1 Toxic: 10 : 1 |
10 : 1 |
Urine microscopy (sediment) | Normal | AKI: dark granular casts, hyaline casts, kidney epithelial cells | Normal |
Anuria, urine volume less than 100 mL/24 hr; oliguria, urine volume of 100-400 mL/24 hr.
*Results of urine laboratory tests are valid only in the absence of diuretics.
†Urine in prerenal failure is concentrated, with low sodium.
‡Urine in intrarenal failure shows kidney damage because the nephron cannot concentrate urine or conserve sodium, and evidence of kidney damage (casts) is seen.
§Urine test results in postrenal failure vary because the findings initially depend on the hydration status of the patient rather than the status of the kidney.
TABLE 20-3
NORMAL SERUM ELECTROLYTE VALUES
ELECTROLYTE | NORMAL VALUE |
Sodium | 135-145 mEq/L |
Potassium | 3.5-4.5 mEq/L |
Chloride | 98-108 mEq/L |
Calcium | 8.5-10.5 mg/dL or 4.5-5.8 mEq/L |
Phosphorus | 2.7-4.5 mg/dL |
Magnesium | 1.5-2.5 mEq/L |
Bicarbonate | 24-28 mEq/L |
TABLE 20-4
SERUM ELECTROLYTES IN ACUTE KIDNEY FAILURE
Acidosis
Acidosis (pH <7.35) is one of the trademarks of severe AKI.17 Metabolic acidosis occurs from accumulated metabolic waste products. The acid waste products consist of strong negative ions (anions), elevated serum phosphorus values (hyperphosphatemia), and other normally unmeasured ions (e.g., sulfate, urate, lactate) that decrease the serum pH.17 A low serum albumin concentration, which often occurs in AKI, has a slight alkalinizing effect, but it is not enough to offset the metabolic acidosis.17 Even respiratory compensation and mechanical ventilatory support are rarely sufficient to reverse the metabolic acidosis. Information on acidosis and arterial blood gas interpretation is found in Chapter 14. Anion gap measurement is discussed in Chapter 19.
Blood Urea Nitrogen
The BUN level is not a reliable indicator of kidney damage.9,18 The BUN concentration is changed by protein intake, blood in the gastrointestinal (GI) tract, and cell catabolism, and it is diluted by fluid administration. An elevated BUN-to-creatinine ratio may signal early AKI. The BUN-to-creatinine ratio is most useful in diagnosing prerenal AKI (often described as prerenal azotemia), in which the BUN value is greatly elevated relative to the serum creatinine value.
Serum Creatinine
Creatinine is a by-product of muscle metabolism that is formed from nonenzymatic dehydration of creatine in the liver18; 98% of creatine is in the muscles,18 and it is almost totally excreted by the kidney tubules. If the kidneys are not working, the serum creatinine level will increase. When the serum creatinine values doubles (e.g., from 0.75 to 1.5 mg/dL), the increase reflects a decrease of approximately 50% in the GFR.18 Serum creatinine level is assessed daily to follow the trend of kidney function and to determine whether the kidney function is stable, getting better, or getting worse.18
Creatinine Clearance
If the patient is making sufficient urine, the urinary creatinine clearance can be measured. A normal urinary creatinine clearance rate is 120 mL/min, but this value decreases with kidney failure. Critical care patients with severe AKI are oliguric, and the urinary creatinine clearance rate is infrequently measured.
Fractional Excretion of Sodium
The fractional excretion of sodium (FENa) in the urine is measured early in the AKI course to differentiate between a prerenal condition and AKI (intrarenal). A FENa value below 1% (in the absence of diuretics) suggests prerenal compromise, because resorption of almost all the filtered sodium is an appropriate response to decreased perfusion to the kidneys. If diuretics are being administered, however, results of the test would be meaningless. A FENa value above 2% implies that the kidney cannot concentrate the sodium and that AKI has occurred.
Urinary sodium is measured in milliequivalents per liter (mEq/L). The interpretation of results is similar to that for FENa. A urinary sodium concentration less than 10 mEq/L (low) suggests prerenal AKI. A urinary sodium level greater than 40 mEq/L (with an elevated serum creatinine in the absence of a high salt load) suggests that intrarenal damage has occurred. As with other urinalysis tests, the use of diuretics invalidates any results.
At-Risk Disease States and Acute Kidney Injury
Many patients come into the critical care unit with disease states that predispose them to the development of AKI. Many others already have kidney damage but are unaware of this condition.7
Underlying Chronic Kidney Disease
The incidence of chronic kidney disease (CKD) in the United States is estimated to be 11% (19.2 million adults).19 Clinical practice guidelines for the management of end-stage kidney disease (ESKD) categorizes kidney dysfunction in five stages.20 Because of the large numbers of adults with kidney dysfunction (diagnosed or not), kidney function must be assessed in all critically ill patients at risk for fluid and electrolyte imbalance. The GFR associated with each stage and the numeric population estimates for each stage of kidney dysfunction are shown in Table 20-5.
TABLE 20-5
DECREASED KIDNEY FUNCTION BY STAGE IN ADULT U.S. POPULATION
STAGE* | POPULATION AFFECTED* | GFR AND DIAGNOSIS* | PERCENTAGE WHO KNOW THEY HAVE KIDNEY DYSFUNCTION (%)† |
1 | 9 million (3.3%) | Normal; persistent albuminuria | 40.5 |
2 | 5.3 million (3.0%) | 60-89; persistent albuminuria | 29.3 |
3 | 7.6 million (4.3%) | 30-59 | 22.0 |
4 | 400,000 (0.2%) | 15-29 | 44.5 |
5 | 300,000 (0.2%) | <15: ESKD | 100 |
*Data from Coresh J et al: Prevalence of chronic kidney disease and decreased kidney function in the adult US population: Third National Health and Nutrition Examination Survey, Am J Kidney Dis 41(1):1-12, 2003. GFR is measured as mL/min/1.73 m2 of body surface area.
†Data from Nickolas TL et al: Awareness of kidney disease in the US population: findings from the National Health and Nutrition Examination Survey (NHANES) 1999 to 2000, Am J Kidney Dis 44(2):185-197, 2004.
Most people in the early stages of kidney disease are unaware of their condition.7 A national health survey queried individuals about whether they had ever been told by their physician that they had ‘weak or failing kidneys’. The answer to this question was correlated with each patient’s GFR and the presence of albumin in the urine was used to stratify the patients according to the five stages of CKD (see Table 20-5). The results showed that more than one half of the respondents were unaware that they had kidney dysfunction until their disease had reached stage 5 or ESKD, when they would become dialysis dependent.7 The results categorized by stage of CKD are listed in Table 20-5.
Older Age and Acute Kidney Injury
Older age appears to be a risk factor for CKD, because 11% of individuals older than 65 years without hypertension or diabetes have stage 3 or worse CKD.19 In the presence of diabetes or hypertension, the risk for CKD increases substantially.
Heart Failure and Acute Kidney Injury
There is a strong association between kidney failure and cardiovascular disease. In studies of critically ill patients with acute kidney failure, 54%4 to 63%5 have acute kidney failure and heart failure. Hypertension, a major contributor to the development of heart failure, is also a major risk factor for the development of CKD.21 Unfortunately, people with hypertension and diabetes are at increased risk for CKD and premature death.21 As a patient’s GFR declines, the risks of cardiovascular disease, myocardial infarction, and death increase, especially for the patient with stage 3 or worse CKD.22,23
Respiratory Failure and Acute Kidney Injury
There is a significant association between respiratory failure and kidney failure. In studies of critically ill patients with kidney failure, 54% to 88% have respiratory failure.4,5 The range in values reflects how the kidney failure was classified. For example, in one study, 57% had respiratory and kidney failure but were not treated with dialysis,4 and in another study, 88% had respiratory and kidney failure and were given dialysis treatment with continuous renal replacement therapy (discussed later).5
The process of mechanical ventilation affects the kidney, although it is not known whether the effect is deleterious.24 Positive-pressure ventilation reduces blood flow to the kidney, lowers the GFR, and decreases urine output.24 These effects are intensified with the addition of positive end-expiratory pressure (PEEP).24 AKI increases inflammation, causes the lung vasculature to become more permeable, and contributes to the development of acute respiratory failure.25 Prolonged mechanical ventilation in critical illness is associated with an increased incidence of AKI and dialysis.26
Sepsis and Acute Kidney Injury
Sepsis is the most common cause of AKI in the critically ill.9 Sepsis and septic shock create hemodynamic instability and reduce perfusion to the kidney. Immunologic, toxic, and inflammatory factors may alter the function of the kidney microvasculature and tubular cells.27 Sepsis caused 19% of AKI in one study4 and 55% of AKI in a population of older hospitalized patients.5 Clinical guidelines for hemodynamic support in sepsis emphasize the need for adequate fluid resuscitation, because in 40% to 50% of cases, reversal of hypotension and restoration of hemodynamic stability can be achieved with fluids alone.28 Unfortunately, in severely septic patients, inflammation increases vascular permeability, and much of the fluid may move into the third space (interstitial space). If the blood pressure remains low, the use of vasopressors is recommended to raise refractory low blood pressure after volume resuscitation.28 Vasopressors raise blood pressure and increase systemic vascular resistance (SVR), but they also may raise the vascular resistance within the kidney microvasculature. Other practices aimed at reversing the deleterious effects of sepsis include maintaining the patient’s hemoglobin level at 7 to 9 g/dL and blood glucose level below 150 mg/dL and ensuring optimal hydration as evidenced by a central venous pressure (CVP) above 8 mm Hg.28
Trauma and Acute Kidney Injury
Trauma Admissions
Trauma patients have different demographics from those of other critical care populations. They are always emergency admissions, are younger, are more often male, and have fewer coexisting illnesses.29 A 5-year retrospective study of 9449 trauma admissions to critical care units in Australia and New Zealand used the RIFLE criteria to determine incidence of AKI in the first 24 hours after admission; AKI developed in 18% of trauma patients.29 However, if patients were older or had preexisting comorbid illnesses, their risk of AKI rose to 35%.29 Although these AKI numbers are high, they likely underestimate the true incidence because the study did not include patients in whom AKI developed more than 24 hours after admission to the critical care unit.29
Rhabdomyolysis
Trauma patients with major crush injuries have an elevated risk of kidney failure because of the release of creatine and myoglobin from damaged muscle cells, a condition called rhabdomyolysis.30 Myoglobin in large quantities is toxic to the kidney. Overall survival from rhabdomyolysis is 77%.30
The level of creatine kinase (CK), a marker of systemic muscle damage, increases in patients with rhabdomyolysis. One trauma service reported that of 2083 trauma patients admitted to critical care, 85% had elevated CK values, and acute kidney failure resulting from rhabdomyolysis developed in 10%.31 A CK level of 5000 units/L was the lowest abnormal value in patients in whom AKI associated with rhabdomyolysis developed.31
Volume resuscitation is the primary treatment for preservation of adequate kidney function and prevention of AKI. In many hospitals, the intravenous (IV) fluids are alkalinized by the addition of sodium bicarbonate, and the urine output is increased by intravenous administration of the diuretic mannitol.30 A bicarbonate and mannitol regimen is instituted to prevent acidosis and hyperkalemia, because both are frequent complications of rhabdomyolysis. Close attention is paid to urine output, CK levels, increases in serum creatinine levels, and any signs of compartment syndrome in all patients admitted with this diagnosis.
Contrast-Induced Acute Kidney Injury
More than one million radiologic studies or procedures that involve use of intravenous radiopaque contrast are performed every year.13 Approximately 1% of the patients undergoing those studies require dialysis as a result of contrast-induced nephrotoxicity,32 with prolongation of the hospital stay to an average of 17 days.13 Patients at risk are those with chronic kidney dysfunction, baseline serum creatinine levels more than 1.5 mg/dL, and known diabetes, heart failure, or volume depletion.33,34 A clinical definition of contrast-induced nephrotoxicity is an increase in serum creatinine concentration of 0.5 mg/dL or more or a 25% increase from the patient’s baseline value within 48 to 72 hours of contrast medium exposure.13,33 The effects of reversible, contrast-induced AKI may not be limited to the immediate hospitalization; it has been linked to a higher mortality in the 5-year period after the reversible AKI than in similar patients who did not have kidney injury.34
Limit Radiopaque Contrast
High-molecular-weight contrast medium is a potential cause of nephrotoxicity.13,35 Kidney-protection strategies include identification of patients at high risk and use of alternative imaging modalities that do not involve contrast. If radiopaque contrast use is inevitable, smaller contrast volumes should be used for each study, and low-osmolar, or iso-osmolar (iohexol), contrast media that are less nephrotoxic should be selected.35,36
Promote Hydration and Avoid Dehydration
The best method of prevention is aggressive hydration with intravenous normal saline during and after the procedure.37,38 After some diagnostic intravascular catheterization procedures, the alert patient is asked to drink several liters of water over a 12-hour period to protect the kidney. Avoiding dehydration is vital. In research studies, the addition of sodium bicarbonate or N-acetylcysteine did not confer additional protection to the vulnerable kidney beyond hydration with normal saline only.37–39
Medications
Several medications have been investigated to mitigate the risk of AKI in at-risk patients who undergo diagnostic studies involving radiopaque contrast agents. The agents include oral N-acetylcysteine, intravenous sodium bicarbonate, and an intravenous infusion of fenoldopam.37–40 In randomized, controlled trials, N-acetylcysteine has not lived up to its early promise.37–40 For sodium bicarbonate, the picture is mixed, with some studies reporting a benefit39,41 and other studies reporting no effect.37,38 Because N-acetylcysteine and sodium bicarbonate are inexpensive and have almost no side effects, many physicians prescribe them on an empirical basis, even though the research evidence is not yet conclusive.
In summary, the mainstay measures to protect the kidney from contrast-induced AKI are to use the smallest dose of low- or iso-osmolar contrast media possible, provide vigorous fluid volume expansion, stop all nephrotoxic drugs, and avoid repeat contrast media injections within 48 hours.42
Hemodynamic Monitoring and Fluid Balance
Hemodynamic monitoring is important for the analysis of fluid volume status in the critically ill patient with AKI.
Hemodynamic Monitoring
Hemodynamic monitoring includes surveillance of the CVP, pulmonary artery occlusion pressure, cardiac output, and cardiac index.43 A less high-tech method that is also important consists of a daily weight and focused physical assessment.
Daily Weight
The daily weight, combined with accurate intake and output monitoring, is a powerful indicator of fluid gains or losses over 24 hours. A 1-kg weight gain over 24 hours represents 1000 mL (1 liter) of additional fluid retention.
Physical Assessment
Physical signs and symptoms are used to assess fluid balance. Signs that suggest extracellular fluid (ECF) depletion include thirst, decreased skin turgor, and lethargy. Signs that imply intravascular fluid volume overload include pulmonary congestion, increasing heart failure, and rising blood pressure. The patient with untreated AKI is edematous. The following factors contribute to this state:
In critical illness, even though there is peripheral edema and the patient may have gained 8 L of fluid over his or her “dry-weight” baseline, the patient may remain “intravascularly dry” and hemodynamically unstable, because the retained fluid is not inside a vascular compartment and cannot contribute to maintenance of hemodynamic stability. The patient with AKI is assessed frequently for pitting edema over bony prominences and in dependent body areas.
Electrolyte Balance
Potassium
Electrolyte levels require frequent observation, especially in the critical phases of AKI (Table 20-4). Potassium may quickly reach levels of 6.0 mEq/L or higher. Specific electrocardiographic changes are associated with hyperkalemia: peaked T waves, a widening of the QRS interval, and ultimately, ventricular tachycardia or fibrillation. If hyperkalemia is identified, all potassium supplements are stopped.44 If the patient is producing urine, intravenous diuretics can be administered. Acute hyperkalemia can be treated temporarily by IV administration of insulin and glucose. An infusion of 50 mL of 50% dextrose accompanied by 10 units of regular insulin forces potassium out of the serum and into the cells.
Sodium polystyrene sulfonate (Kayexalate), a cation-exchange resin, is mixed in water and sorbitol and given orally, rectally, or through a nasogastric tube. The resin binds potassium in the bowel, which eliminates it in the feces. Kayexalate and dialysis are the only permanent methods of potassium removal.44
Sodium
Dilutional hyponatremia, associated with kidney failure, is an expected finding in AKI (see Table 20-4). It can be corrected over a few days with fluid restriction. Sodium levels may be raised more rapidly during dialysis by changing the amount of sodium in the dialysate bath.
Calcium and Phosphorus
Serum calcium levels are reduced (hypocalcemia) in kidney failure (see Table 20-4). This reduction results from multiple factors, including hyperphosphatemia. Long-term elevations of serum phosphorus (>5.5 to 6.5 mEq/L) are associated with higher mortality rates for patients in kidney failure.20,45,46 Calcium and phosphorus are regulated in part by parathyroid hormone (PTH). Normally, PTH helps calcium be resorbed back into the bloodstream at the proximal tubule and distal nephron, and it promotes excretion of phosphorus by the kidney to maintain homeostasis. In kidney failure, this mechanism is nonfunctional; the serum phosphorus level rises, and the serum calcium level falls.
Calcium Replacement
Most calcium in the bloodstream is bound to protein. Calcium levels can be measured in two ways: total calcium (tCa) or ionized calcium (iCa).46 Unfortunately, protein-calcium binding confounds the measurement of accurate calcium levels. In the past, calculations were used to estimate the amounts of protein-bound versus unbound calcium, but these calculations have been shown to produce inaccurate results.47 The metabolically active, non–protein-bound portion is known as the ionized calcium and is the preferred method of measurement.47 Without adequate levels of serum calcium, a compensatory mechanism ‘steals’ calcium from the bones, making the patient with kidney failure more vulnerable to fractures.48 Maintaining adequate calcium stores in the body is important and is achieved by administration of calcium supplements, vitamin D preparations, and synthetic calcitriol.49
Dietary Phosphorus–Binding Drugs
A second method used in tandem with calcium supplements to achieve normal calcium levels is to lower the level of phosphorus in the bloodstream.46 Phosphorus occurs in many foods, and eliminating all phosphorus-containing foods from the diet would make it unpalatable.50 Foods that contain particularly high levels of phosphorus include dairy products, processed meats, some carbonated drinks, and nuts.50 After these foods are eaten, free phosphorus passes from the GI tract into the bloodstream and raises the serum level. Medications that bind dietary phosphorus in the gastrointestinal tract are administered orally or by nasogastric tube. The binding agent must be taken at the same time as a meal.50 After the dietary phosphorus is bound to the binding substance in the bowel, it is eliminated from the intestine with stool. This lowers the serum phosphorus level.
The types of dietary-phosphorus binders used have changed over the years. The original binders were aluminum salts (aluminum hydroxide) that bound dietary phosphorus effectively in the gastrointestinal tract but conferred aluminum toxicity because some of the aluminum metal was also absorbed.50 For this reason, aluminum binders have largely been abandoned.50
The second generation of dietary phosphorus–binding agents that are most widely prescribed use calcium salts (calcium carbonate; calcium acetate [PhosLo]) to bind dietary phosphorus in the gastrointestinal tract. Calcium-based drugs are safer, but elevated serum calcium values and calcium deposits in other areas of the body (extraosseous calcification) are a problem.50 This category of drugs remains in widespread use.
A third generation of dietary phosphorus–binding medications is available. They are not aluminum- or calcium-based. They include sevelamer hydrochloride (RenaGel) and lanthanum carbonate (Fosrenol).50
Medical Management
Treatment Goals
Treatment goals for patients with AKI focus on prevention, compensation for the deterioration of kidney function, and regeneration of the remaining kidney functional capacity. Over the past 4 decades, the mortality rate for AKI has remained at more than 50%.4,5 Key areas that are evaluated include prevention strategies, fluid balance, anemia, medications, and electrolyte imbalance.
Prevention
The only truly effective remedy for AKI is prevention. Effective prevention requires assessment of the patient’s risk for AKI. Knowledge of the most frequent causes of AKI in the critically ill is essential if prevention strategies are to be enacted. The critical care team collaborates closely with the clinical pharmacist to avoid drugs with nephrotoxic side effects in patients with AKI or CKD.19,20 Nonsteroidal anti-inflammatory drugs (NSAIDs) for pain relief are avoided in patients with elevated creatinine values.2 The use of intravascular contrast dye is preferably delayed until the patient is fully rehydrated.42
Fluid Resuscitation
Prerenal failure is caused by decreased perfusion and flow to the kidney. It is often associated with trauma, hemorrhage, hypotension, and major fluid losses. If contrast dye is used, aggressive fluid resuscitation with normal saline (NaCl) is recommended. Fluid replacement is the only treatment shown to prevent kidney tubular injury.51 The objectives of volume replacement are to replace fluid and electrolyte losses and to prevent ongoing loss. Maintenance intravenous fluid therapy is initiated when oral (PO) fluid intake is inadvisable. Maintenance fluids are calculated with consideration for individual body surface area. Adults require approximately 1500 mL/m2/24 hr; fever, burns, and trauma significantly increase fluid requirements. Other important criteria in the calculation of fluid volume replacement include baseline metabolism, environmental temperature, and humidity. The rate of replacement depends on cardiopulmonary reserve, adequacy of kidney function, urine output, fluid balance, ongoing loss, and type of fluid replaced.
Crystalloids and Colloids
Crystalloids and colloids are two different types of intravenous fluids used for volume management in critically ill patients. These intravenous solutions are used on all types of patients, not just those with acute kidney failure. Adequacy of intravenous fluid replacement depends on strict, ongoing evaluation and frequent adjustment. Frequent monitoring of serum electrolyte levels is required, and strictly regulated intake and output are correlated with daily weight records. In septic shock, hemodynamic readings are frequently undertaken. After a fluid challenge, a merely minimal increase in CVP implies that additional fluid replacement is required. Continued decreases in CVP, pulmonary artery occlusion pressure, and the cardiac index indicate ongoing volume losses.
Which intravenous fluid to select to most successfully resuscitate hemodynamically unstable patients has been a controversial topic in critical care. The major debate centers on the differences between crystalloid and colloid solutions.
Crystalloids
Crystalloid solutions, which are balanced salt solutions, are in widespread use for maintenance infusion and replacement therapy. Crystalloid fluids include normal saline solution (0.9 NaCl), half-strength saline solution (0.45 NaCl), and lactated Ringer’s (LR) solution (Table 20-6). LR solution usually is avoided in patients with kidney failure because it contains potassium. A noncrystalloid solution that may be infused is dextrose (5% or 10%) in water (D5W, D10W).
TABLE 20-6
Frequently Used Intravenous Solutions
SOLUTION | ELECTROLYTE COMPONENTS, PH, OXMOLALITY | INDICATIONS |
Crystalloids* | ||
Dextrose in water (D5W), isotonic | ||
Normal saline solution (0.9% NaCl) | ||
Half-strength saline solution (0.45% NaCl) | ||
Lactated Ringer’s solution | ||
Colloids | ||
5% Albumin (Albumisol) | ||
25% Albumin (salt-poor) | ||
Hetastarch | ||
Low-molecular-weight dextran (LMWD) | ||
High-molecular-weight dextran (HMWD) |
*For crystalloid solutions that contain electrolytes, specific concentrations of electrolytes and pH vary according to the manufacturer.
Colloids
Colloids are solutions containing oncotically active particles that are used to expand intravascular volume to achieve and maintain hemodynamic stability. Albumin (5% and 25%) and hetastarch are colloid solutions (see Table 20-6). Colloids expand intravascular volume, and the effect can last as long as 24 hours. The goal is to optimize the pulmonary artery occlusion pressure (PAOP), raise mean arterial pressure (MAP), and increase cardiac output and the cardiac index to a therapeutic level.
The controversy over whether colloids or crystalloid intravenous fluids are most effective for volume resuscitation appears to have been put to rest by a series of randomized clinical trials and meta-analytic reviews. The SAFE study (saline versus albumin fluid evaluation) was a randomized, prospective, double-blind trial that examined whether the selection of resuscitation fluid in the critical care unit affected survival at 28 days. This was a huge study, with almost 7000 critical care patients randomly assigned to two similar treatment groups. One group received 4% albumin, and the other group received 0.9 normal saline (NaCl) for fluid resuscitation. The patients in the two groups were similar in terms of organ dysfunction, mechanical ventilator support (64% of patients), and renal replacement therapy (1% of patients). The SAFE results showed that there was no difference in the mortality rate, time in the critical care unit, ventilator days, or renal replacement therapy days between the treatment groups. The researchers concluded that albumin and saline should be considered clinically equivalent treatments for intravascular volume expansion in critically ill patients.52 The exception was for patients with traumatic brain injury (TBI), in whom albumin was associated with a higher mortality rate.53
The findings of the SAFE study investigators have been validated by a systematic review of randomized trials of crystalloids versus colloids that reported no difference in mortality rates for the critically ill and injured based on resuscitation fluid.54 Consequently, colloids are not favored because of their higher cost, and crystalloids are the recommended fluid to use for resuscitation in critical care.
Fluid Restriction
Fluid restriction constitutes a large part of the medical treatment for acute kidney failure. Fluid restriction is used to prevent circulatory overload and the development of interstitial edema when the kidneys cannot remove excess volume. The fluid requirements are calculated on the basis of daily urine volumes and insensible losses. Obtaining daily weight measurements and keeping accurate intake and output records is essential. Patients with kidney failure are usually restricted to 1 L of fluid per 24 hours if the urine output is 500 mL or less. Insensible losses range from 500 to 750 mL/day.
Fluid Removal
Acute kidney failure promotes increased amounts of water, solutes, and potential toxins in the circulation, and prompt measures are needed to decrease their levels. Diuretics are used to stimulate the urine output. However, renal replacement therapy (hemodialysis or hemofiltration) is the treatment of choice, particularly if volume overload exacerbates acute lung injury and heart failure.
Pharmacologic Management
The first step is to eliminate all nephrotoxic medications. Second, if drugs are eliminated through the kidneys, it is important to decrease the frequency of administration (e.g., from every 6 hours to every 12 or 24 hours) or to decrease the dose and to monitor the serum concentration by measuring serum drug levels.55
Diuretics
Diuretics are used to stimulate urinary output in the fluid overloaded patient with functioning kidneys. Care must be taken in their use to avoid the creation of secondary electrolyte abnormalities (Table 20-7). Diuretics reduce volume overload and are helpful for symptoms such as pulmonary edema, but they have not been shown to prevent AKI.56 Diuretics are used in many patient populations other than those with incipient kidney failure.
TABLE 20-7
PHARMACOLOGIC MANAGEMENT: KIDNEY-RELATED MEDICATIONS
Loop Diuretics
The loop diuretic furosemide (Lasix) is the most frequently used diuretic in critical care patients. It may be prescribed as a bolus dose or as a continuous infusion.56 Electrolytic abnormalities are frequently encountered with the use of furosemide, and close monitoring of serum potassium, magnesium, and sodium values is essential.57,58
The optimal use of furosemide and other diuretics in critical illness is being actively investigated. Mehta and colleagues published an observational study showing that for critically ill patients with established AKI and oliguria, loop diuretics increased the mortality rate and delayed kidney recovery.59 A subsequent study found that furosemide helped maintain urine output but had no more impact on survival and recovery of kidney function than a placebo.60 A third research trial found that furosemide neither helped nor worsened AKI in the critically ill.61 The debate and research studies will continue, but for now, it appears that loop diuretics increase the urine output and reduce volume overload, but have no impact on the outcome of established oliguric AKI.56,62 Studies are under way to determine the optimal use of furosemide in critical illness.63
Thiazide Diuretics
Diuretics may be prescribed in combination. A thiazide diuretic such as chlorothiazide (Diuril), hydrochlorothiazide (HydroDiuril), or the thiazine-like diuretic or metolazone (Zaroxolyn) may be administered and followed by a loop diuretic to take advantage of the fact that these drugs work on different parts of the nephron. The diuretic efficacy of chlorothiazide and hydrochlorothiazide is reduced when creatine clearance is less than 30 mL/minute.
Osmotic Diuretics
Osmotic diuretics (e.g., mannitol) are used to decrease fluid overload and improve urine output. It is important to use an in-line 5-micron filter when administering this drug. Mannitol is frequently administered to patients with brain injury and increased intracranial pressure (ICP). More information on the use of mannitol in this population can be found in Chapter 17.
Heart Failure
For patients with heart failure, two of the natriuretic peptides (atrial natriuretic peptide [ANP] and brain natriuretic peptide [BNP], which acts on the ventricles) may be prescribed. These drugs work by stimulating natriuretic receptors in the atrium (ANP) and ventricular myocardium (BNP). The potassium-sparing diuretic Aldactone (spironolactone) is also used in heart failure management, not as a diuretic but primarily as an aldosterone antagonist to lower aldosterone levels. Eplerenone (INSPRA) is a newer aldosterone antagonist used to treat chronic heart failure. Most heart failure patients and hypertensive patients require loop diuretics as part of their medication regimen to control fluid volume overload. More information on the use of diuretics in heart failure management is provided in Chapters 12 and 13.
Dopamine
Low-dose dopamine (2 to 3 mcg/kg/min), previously known as renal-dose dopamine, is infused to stimulate blood flow to the kidney. Dopamine is effective in increasing urine output in the short term, but tolerance of the dopamine renal receptor to the drug is theorized to develop in the critically ill patients who are most at risk for AKI.64 A meta-analysis of related research studies determined that renal-dose dopamine did not prevent onset of AKI and did not decrease the need for dialysis or reduce mortality.65 At this point, the support for routine use of low-dose dopamine for the prevention of AKI remains anecdotal only.66 See Table 13-16 and the Priority Medications Box: Dopamine in Chapter 13 for more information on dopamine.
Acetylcysteine
N-Acetylcysteine (Mucomyst, Mucosil) is an N-acetyl derivative of the amino acid L-cysteine. It has been used for many years as a mucolytic agent to assist with expectoration of thick pulmonary secretions. It is also frequently prescribed for patients with mildly elevated serum creatinine values before a radiologic study using contrast dye.67 In research trials, the addition of N-acetylcysteine to normal saline hydration has not showed a reduction in the incidence of contrast-induced AKI.37,39,67 In clinical trials enrolling cardiac surgery patients, prophylactic use of N-acetylcysteine did not decease the rate of AKI.67,68 Most studies suggest that N-acetylcysteine does not prevent AKI during radiologic procedures or after cardiac surgery.
Fenoldopam
Fenoldopam mesylate (Corlopam) is a dopamine 1 (D1) receptor agonist similar in structure to dopamine and dobutamine. It is used to lower blood pressure. Claims that this drug would prevent contrast-induced nephrotoxicity in high-risk patients have not been supported by clinical trial results.40
Dietary Phosphorus Binders
Many patients with kidney failure are prescribed a dietary phosphorus–binding medication (see earlier “Dietary-Phosphorus Binding Drugs”).50 Many dietary phosphorus–binding drugs are available, and some important issues concern all of them. The dietary phosphorus binder must be taken at the time of the meal. If it is taken 2 hours later, it will increase only the level of the binding substance (e.g., calcium) in the bloodstream and will not lower the serum phosphorus level. Related issues such as the quantity of phosphorus in the diet should be discussed with a clinical nutritionist (dietitian).
Nutrition
Diet or nutritional supplementation for the patient with AKI in the critical care unit is designed to account for the diminished excretory capacity of the kidney. The recommended energy intake is between 20 and 30 kilocalories/kg per day, with 1.2 to 1.5 g/kg of protein per day to control azotemia (increased BUN level).69 Oral nutrition is preferred, and if the patient cannot eat, enteral nutrition is recommended over parenteral (intravenous) nutrition. Fluids are limited, and tight glucose control is recommended.69 The electrolytes potassium, sodium, and phosphorus are strictly limited.
Nursing Management
Nursing management of the patient with AKI involves a variety of nursing diagnoses (see Nursing Diagnosis Priorities box on Acute Kidney Dysfunction). ‘Prevention is the best cure’ is an old saying that captures the role of the critical care nurse, who evaluates all patients for level of kidney function, risk of infection, fluid imbalance, electrolyte disturbances, anemia, readiness to learn, and need for education.
Risk Factors for Acute Kidney Injury
Some individuals are at increased risk for AKI as a complication during hospitalization, and the alert critical care nurse recognizes potential risk factors and acts as a patient advocate.19,20 Patients at risk include older persons because their GFR may be decreased,5 dehydrated patients with kidney hypoperfusion, patients who have increased creatinine values before hospitalization, and patients undergoing a radiologic procedure involving contrast dye.
Infectious Complications
The critical care patient with infectious complications is at risk for AKI. Signs of infection such as an increased white blood cell (WBC) count, redness at a wound or intravenous line site, or increased temperature are always a cause for concern. A urinary catheter is inserted to facilitate accurate urine measurement and patient comfort. However, any indwelling catheter is a potential source for infection. When the patient no longer makes large quantities of urine and is hemodynamically stable, the catheter must be removed promptly. If the patient cannot void urine spontaneously, a scheduled straight catheterization is performed to minimize the risk of infection from an indwelling catheter and drainage system. This method allows the patient’s bladder to be emptied, but the urinary catheter does not remain in place.
Fluid Balance
Intravascular fluid balance is often assessed on an hourly basis for the critically ill patient in whom hemodynamic lines have been inserted. Hemodynamic values (heart rate, blood pressure, CVP, PAOP, cardiac output, and cardiac index) and daily weight measurements are correlated with the intake and output. Urine output is measured hourly by means of a urinary catheter and drainage bag throughout all phases of AKI, particularly in response to diuretics. Any fluid removed with dialysis is included in the daily fluid balance. Recognition of the clinical signs and symptoms of fluid overload is important. Excess fluid moves from the vascular system into the peripheral tissues (dependent edema), abdomen (ascites), and lungs (crackles, pulmonary edema, and pulmonary effusions); around the heart (pericardial effusions); and into the brain (increased intracranial swelling).
Electrolyte Imbalance
Hyperkalemia, hypocalcemia, hyponatremia, hyperphosphatemia, and acid-base imbalances occur during AKI (see Table 20-4). Clinical manifestations of these electrolyte imbalances must be prevented and their associated side effects controlled. The more likely imbalances are hyperkalemia and hypocalcemia, which can result in life-threatening cardiac dysrhythmias. Dilutional hyponatremia may develop as fluid overload worsens in the patient with oliguria. Monitoring the serum sodium level is important to prevent this complication. Hyperphosphatemia results in severe pruritus. Nursing care is directed at soothing the itching by performing frequent skin care with emollients, discouraging scratching, and administering phosphate-binding medications.50 The acid-base imbalances that occur with AKI are monitored by arterial blood gas (ABG) analyses. The goal of treatment is to maintain the pH within the normal range.
Preventing Anemia
Anemia is an expected side effect of kidney failure that occurs because the kidney no longer produces the hormone erythropoietin.70 As a result, the bone marrow is not stimulated to produce red blood cells (RBCs). Decreased RBC production by the bone marrow also occurs as a consequence of critical illness.71 Care is taken to prevent blood loss in the patient with AKI, and blood withdrawal is minimized as much as possible. Irritation of the GI tract from metabolic waste accumulation is expected, and stress ulcer prophylaxis must be prescribed. Gastrointestinal bleeding remains a possibility. Stool, nasogastric tube drainage, and emesis are routinely tested for occult blood. Anemia may be treated pharmacologically by the administration of recombinant human erythropoietin (rhEPO), epoetin alfa (Procrit, Epogen), to stimulate erythrocyte production by the bone marrow and if required by RBC transfusion. Treatment of anemia early in the course of AKI (before dialysis) appears to slow the progression of the kidney failure and delays the initiation or renal replacement therapies.72 Even in anemic, critically ill patients without kidney failure, administration of rhEPO weekly significantly decreases the number of blood transfusions.73 However, this therapy is not recommended for anemia related to sepsis in the critically ill.28
Patient Education
Accurate and uncomplicated information must be provided to the patient and family about AKI, including its prognosis, treatment, and possible complications.7 Education of the patient can be challenging because elevations of BUN and creatinine can negatively affect the level of consciousness. Sleep-rest disorders and emotional upset often occur as complications of AKI and can disrupt short-term memory. Encouraging the patient and family to voice concerns, frustrations, or fears and allowing the patient to control aspects of the acute care environment and treatment are essential.
Renal Replacement Therapy: Dialysis
Two types of renal replacement therapy are available for the treatment of AKI. They are intermittent hemodialysis (IHD) therapy and continuous renal replacement therapy (CRRT).
Hemodialysis
Hemodialysis roughly translates as ‘separating from the blood.’ Indications and contraindications for hemodialysis are listed in Box 20-4. As a treatment, hemodialysis separates and removes from the blood the excess electrolytes, fluids, and toxins by means of a hemodialyzer (Figure 20-2). Although hemodialysis is efficient in removing solutes, it does not remove all metabolites. Levels of electrolytes, toxins, and fluids increase between treatments, necessitating hemodialysis on a regular basis. Hemodialysis therapy is always intermittent; each dialysis treatment takes 3 to 4 hours. In the acute phases of kidney failure, dialysis is performed daily.74 The dialysis frequency gradually decreases to three times per week as the patient moves into a more chronic phase of kidney failure.
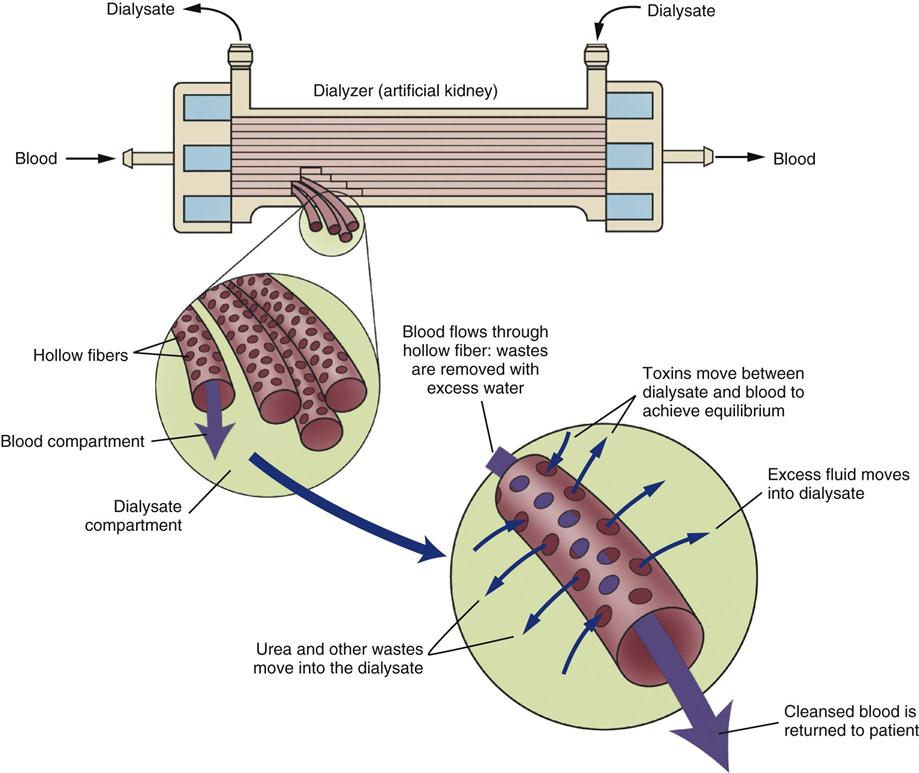
Hemodialyzer
Hemodialysis works by circulating blood outside the body through synthetic tubing to a dialyzer, which consists of hollow-fiber tubes. The dialyzer is sometimes described as an artificial kidney (Figure 20-3). While the blood flows through the membranes, which are semipermeable, a fluid (dialysate bath) bathes the membranes and, through osmosis and diffusion, performs exchanges of fluid, electrolytes, and toxins from the blood to the bath, where toxins and dialysate then pass out of the artificial kidney. The blood and the dialysate bath are shunted in opposite directions (countercurrent flow) through the dialyzer to match the osmotic and chemical gradients at the most efficient level for effective dialysis.
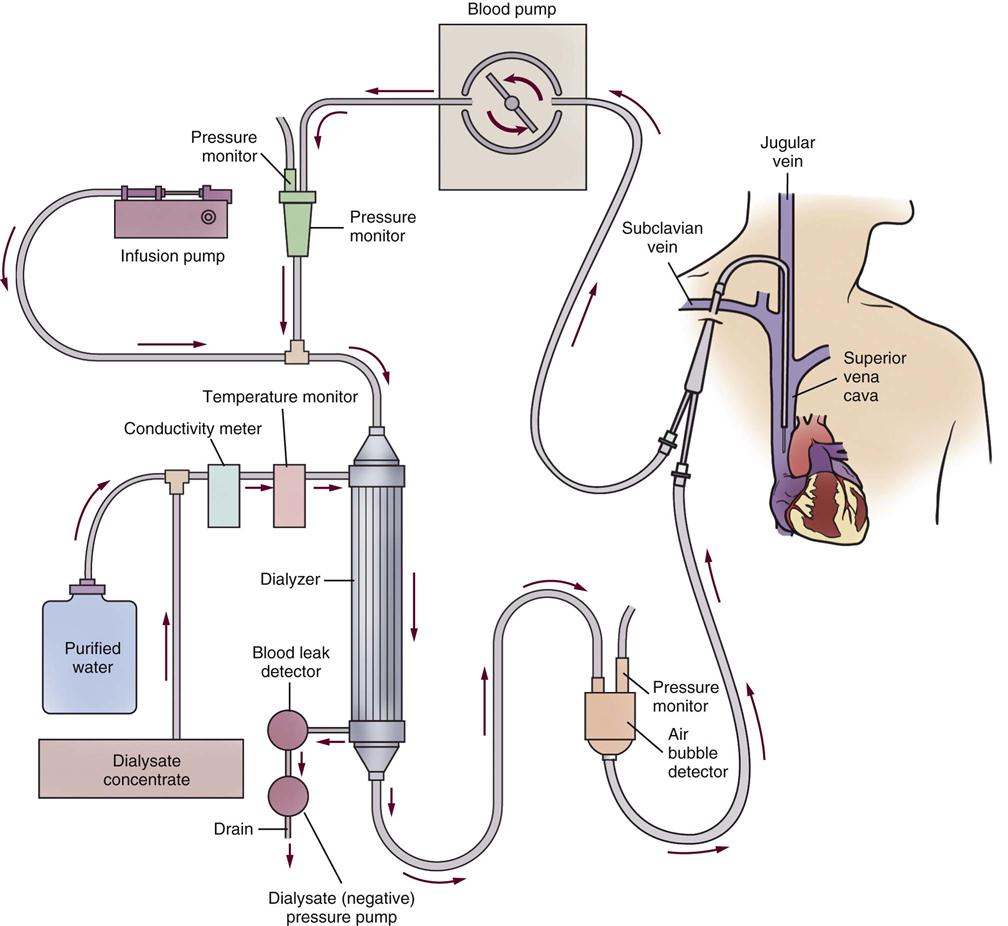
Ultrafiltration
To remove fluid, a positive hydrostatic pressure is applied to the blood, and a negative hydrostatic pressure is applied to the dialysate bath. The two forces together, called transmembrane pressure, pull and squeeze the excess fluid from the blood. The difference between the two values (expressed in millimeters of mercury [mm Hg]) represents the transmembrane pressure and results in fluid extraction, known as ultrafiltration, from the vascular space.
Anticoagulation
Heparin or sodium citrate is added to the system just before the blood enters the dialyzer to anticoagulate the blood within the dialysis tubing. Without an anticoagulant, the blood clots because its passage through the foreign tubular substances of the dialysis machine activates the clotting mechanism. Heparin can be administered by bolus injection or intermittent infusion. It has a short half-life, and its effects subside within 2 to 4 hours. If necessary, the effects of heparin are easily reversed with the antidote protamine sulfate. When there is concern about the development of heparin-induced thrombocytopenia (HIT),75 alternative anticoagulants can be used. Citrate (trisodium citrate) can be infused as an anticoagulant by intermittent bolus or continuous infusion.76
Vascular Access
Hemodialysis requires access to the bloodstream. Various types of temporary and permanent devices are in clinical use. It is important for patient safety that the nurse be able to recognize these different vascular access devices and properly care for them. The following section discusses temporary vascular access catheters used in the acute care hospital environment and permanent methods used for long-term hemodialysis.
Temporary Acute Access
Subclavian and femoral veins are catheterized when short-term access is required or when vascular access is nonfunctional in a patient requiring immediate hemodialysis. Subclavian and femoral catheters are routinely inserted at the bedside. Most temporary catheters are venous lines only. Blood flows out toward the dialyzer and flows back to the patient through the same vein. A dual-lumen venous catheter is most commonly used. It has a central partition running the length of the catheter. The outflow catheter section pulls the blood flow through openings that are proximal to the inflow openings on the opposite side (Figure 20-4). This design helps prevent dialyzing the same blood just returned to the area (recirculation), which would severely reduce the procedure’s efficiency. A silicone rubber, dual-lumen catheter with a polyester cuff designed to decrease catheter-related infections is also available.
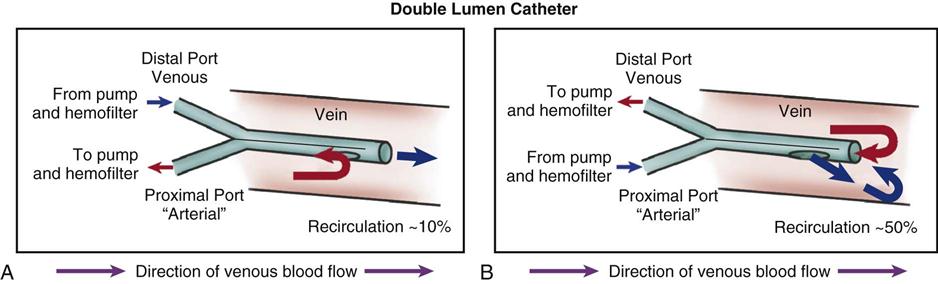
Permanent Vascular Access
The common denominator in permanent vascular access devices is a connection to the arterial circulation and a return conduit to the venous circulation.
Arteriovenous Fistula
The arteriovenous fistula is created by surgically exposing a peripheral artery and vein, creating a side-by-side opening in the artery and the vein that joins the two vessels together. The high arterial flow creates a swelling of the vein, or a pseudoaneurysm, at which point (when healed) a large-bore needle can be inserted to obtain arterial outflow to the dialyzer. Inflow is accomplished through a second large-bore needle inserted into a peripheral vein distal to the fistula (Figure 20-5). If the patient’s vessels are adequate, fistulas are the preferred mode of access because of the durability of blood vessels, relatively few complications, and less need for revision in comparison with other access methods.20 An initial disadvantage of a fistula concerns the time required for development of sufficient arterial flow to enlarge the new access. The minimum reported length of time before a fistula can be cannulated for dialysis is 14 days,77 but the time lag for many patients is longer, as much as weeks or months.78
In the care of a patient with a fistula, some important nursing priorities ensure the ongoing viability of the vascular access and safety of the limb (Table 20-8). The critical care nurse frequently assesses the quality of blood flow through the fistula. A patent fistula has a thrill when palpated gently with the fingers and has a bruit if auscultated with a stethoscope. The extremity should be pink and warm to the touch. No blood pressure measurements, intravenous infusions, or laboratory phlebotomy procedures are performed on the arm with the fistula.79
TABLE 20-8
COMPLICATIONS AND NURSING MANAGEMENT OF ARTERIOVENOUS FISTULA OR GRAFT
TYPE | COMPLICATIONS | NURSING MANAGEMENT |
Fistula | ||
Graft |
The arteriovenous fistula is the preferred long-term access for hemodialysis.20 However, in the United States, only 27% of hemodialysis patients have an arteriovenous fistula; 47% have a synthetic graft; and 23% have a tunneled hemodialysis catheter.80 An arteriovenous fistula provides the most favorable long-term patency for hemodialysis access and is recommended if patients require long-term hemodialysis.20
Arteriovenous Grafts
Arteriovenous grafts are vascular access devices for treating chronic kidney failure. The graft is a tube made of synthetic material that is surgically implanted inside the limb. The area is surgically opened, and an artery and a vein are located. A tunnel is created in the tissue where the graft is placed. Anastomoses are made with the graft ends connected to the artery and vein. The blood is allowed to flow through the graft, and the surgical area is closed. The graft creates a raised area that looks like a large peripheral vein just under the skin and peripheral tissue layers (see Figure 20-5). Two large-bore needles are used for outflow from and inflow to the graft. For grafts and fistulas, after needle removal at the end of the hemodialysis treatment, firm pressure must be applied to stop any bleeding (see Table 20-8).
Tunneled Catheters
While waiting for the fistula or graft to mature to be ready for access, some patients with CKD may have a tunneled, cuffed catheter placed.81 The cuff and tunneling are physical barriers to reduce central venous catheter infections. Modern catheters are made of silicone or polyurethane, tunneled under the skin, and inserted through the jugular or subclavian vein into the superior vena cava.77,81
Medical Management
Medical management involves the decision to place a vascular access device and then to choose the most appropriate type and location for each patient. Patients in the critical care setting who require vascular access for hemodialysis typically use a temporary hemodialysis catheter. The exact quantity of fluid and solute removal to be achieved by hemodialysis is determined individually for each patient by clinical examination and review of all relevant laboratory results.
Nursing Management
A noncritical care nurse who is specially trained in dialysis manages the IHD. The dialysis nurse typically comes to the patient’s bedside with the hemodialysis machine. During the acute phase of treatment, hemodialysis occurs daily. The frequency is reduced to 3 days per week as the patient becomes hemodynamically stable. The essential role of the critical care nurse during dialysis is to monitor the patient’s hemodynamic status and ensure that the patient remains hemodynamically stable. The AKI patient undergoing hemodialysis depends on a viable venous access catheter. When not in use, the catheter is ‘heparin-locked’ to preserve patency. The critical care nurse provides education about the disease process and treatment plan to patient and family.
Continuous Renal Replacement Therapy
CRRT is a newer mode of dialysis that has many similarities to traditional hemodialysis. CRRT is a continuous therapy that is monitored by the critical care nurse, and it may continue over many days. The venous blood is circulated through a highly porous hemofilter. As with traditional hemodialysis, access and return of blood are achieved through a large venous catheter (venovenous). The CRRT system allows the continuous removal of fluid from the plasma. The patient’s blood flow is 100 to 200 mL/min, and the dialysate flow is 17 to 40 mL/min.82 The fluid removal rate varies depending on the particular CRRT method used and removal of solutes (urea, creatinine, and electrolytes), as listed in Table 20-9. The removed fluid is described as ultrafiltrate. In an ideal situation, the hydrostatic pressure exerted by an MAP greater than 70 mm Hg would propel a continuous flow of blood through the hemofilter to remove fluid and solute. However, because many critically ill patients are hypotensive and cannot provide adequate flow through the hemofilter, an electric roller pump ‘milks’ the tubing to augment flow. If large amounts of fluid are to be removed, intravenous replacement solutions are infused. Indications and contraindications for CRRT are described in Box 20-5.
TABLE 20-9
COMPARISON OF CONTINUOUS RENAL REPLACEMENT THERAPY METHODS
TYPE | ULTRAFILTRATION RATE | FLUID REPLACEMENT | METHOD OF SOLUTE REMOVAL | INDICATION |
SCUF | 100-300 mL/hr | None | None | Fluid removal |
CVVH | 500-800 mL/hr | Predilution or postdilution, calculating hourly net loss | Convection | Fluid removal, moderate solute removal |
CVVHD | 500-800 mL/hr | Predilution or postdilution, subtracting dialysate, then calculating hourly net loss | Diffusion | Fluid removal, maximum solute removal |
CVVHDF | Predilution or postdilution, subtracting dialysate, then calculating hourly net loss | Convection and diffusion | Maximal fluid removal, maximal solute removal |
Controversy exists about when CRRT should be started, what the optimal dialysis dose is, which patients can derive the greatest benefit, and when CRRT should be discontinued.83–86 The debate over the optimal “dose” of dialysis is likely to continue because the multicenter Veterans Administration and National Institutes of Health trial showed no difference in mortality between critically ill patients receiving intensive and those receiving nonintensive dialysis regimens.87 Other studies have confirmed that a high CRRT “dose” does not improve mortality.88
Because controlled removal and replacement of fluid are possible over many hours or days with CRRT, hemodynamic stability is maintained. This makes CRRT highly advantageous for use in the hemodynamically unstable patient with multisystem problems. The following modes of CRRT are used in critical care units85,86:
• Slow continuous ultrafiltration (SCUF)
• Continuous venovenous hemofiltration (CVVH)
The decision about which type of therapy to initiate is based on clinical assessment, metabolic status, severity of uremia, whether a particular treatment modality is available at that institution, and other factors.
Terminology for Continuous Renal Replacement Therapy
In CRRT, solutes are removed from the blood by diffusion or convection. Both processes remove fluid, and the two methods remove molecules of different sizes.
Diffusion
Diffusion describes the movement of solutes along a concentration gradient from a high concentration to a low concentration across a semipermeable membrane. This is the main mechanism used in hemodialysis. Solutes such as creatinine and urea cross the dialysis membrane from the blood to the dialysis fluid compartment.
Convection
Convection occurs when a pressure gradient is set up so that the water is pushed or pumped across the dialysis filter and carries the solutes from the bloodstream with it. This method of solute removal is known as solvent drag, and it is commonly employed in CRRT.
Absorption
The filter attracts solute, and molecules attach (adsorb) to the dialysis filter. The size of solute molecules is measured in daltons. The different sizes of molecules that can be removed by convection or diffusion methods are shown in Table 20-10. Tiny molecules such as urea and creatinine are removed by diffusion and convection (all methods). As the molecular size increases above 500 daltons, convection is the more efficient method.
TABLE 20-10
SIZE OF MOLECULES CLEARED BY CONTINUOUS RENAL REPLACEMENT THERAPY
TYPE OF MOLECULE | SIZE OF MOLECULE | SOLUTES | SOLUTE REMOVAL METHOD |
Small | <500 daltons | Urea, creatinine | Convection, diffusion |
Middle | 500-5000 daltons | Vancomycin | Convection better than diffusion |
Low-molecular-weight (small) proteins | 5000-50,000 daltons | Cytokines, complement | Convection or absorption onto hemofilter |
Large proteins | >50,000 daltons | Albumin | Minimal removal |
Ultrafiltrate Volume
The fluid that is removed each hour is not called urine; it is known as ultrafiltrate.
Replacement Fluid
Typically, some of the ultrafiltrate is replaced through the CRRT circuit by a sterile replacement fluid. The replacement fluid can be added before the filter (prefilter dilution) or after the filter (postfilter dilution). The purpose is to increase the volume of fluid passing through the hemofilter and improve convection of solute.
Anticoagulation
Because the blood outside the body is in contact with artificial tubing and filters, the coagulation cascade and complement cascades are activated. To prevent the hemofilter from becoming obstructed by clotting, or clotting off, low-dose anticoagulation must be used.89 The dose should be low enough to have no effect on patient anticoagulation parameters. Systemic anticoagulation is not the goal. Typical anticoagulant choices include unfractionated heparin (UFH) and sodium citrate.76,89 Citrate is an effective prefilter anticoagulant, which has the side effect that it chelates (binds to and removes) calcium from the blood. Consequently, ionized calcium levels are verified, and calcium is replaced per protocol when sodium citrate is the anticoagulant.
Methods of Continuous Renal Replacement
Because of the design of the CRRT machine, it is not possible to look at the outside and follow the flow of blood and, if used, dialysate. Each of the CRRT methods is described here, and diagrams are employed to clarify the mode of CRRT that is used (Figure 20-6, A-D).
Slow Continuous Ultrafiltration
SCUF slowly removes fluid (100 to 300 mL/hr) through a process of ultrafiltration (see Figure 20-6, A). It consists of a movement of fluid across a semipermeable membrane. SCUF has minimal impact on solute removal. Because this process removes small amounts of fluid, it was initially hoped that it would be a suitable choice for edematous patients with acute heart failure and diminished kidney perfusion. However, SCUF is an infrequent clinical choice because it requires both arterial and venous access and is more likely to thrombose (clot off) than other CRRT methods that use higher flows.
Continuous Venovenous Hemofiltration
CVVH is indicated when the patient’s clinical condition warrants removal of significant volumes of fluid and solutes (see Figure 20-6, B). Fluid is removed by ultrafiltration in volumes of 5 to 20 mL/min or up to 7 to 30 L/24 hr. Removal of solutes such as urea, creatinine, and other small non-protein-bound toxins is accomplished by convection. The replacement fluid rate of flow through the CRRT circuit can be altered to achieve desired fluid and solute removal without causing hemodynamic instability.
As with other CRRT systems, the blood outside the body is anticoagulated, and the ultrafiltrate is drained off by gravity or by the addition of negative-pressure suction into a large drainage bag. Because large volumes of fluid may be removed in CVVH, some of the removed ultrafiltrate volume must be replaced hourly with a continuous infusion (replacement fluid) to avoid intravascular dehydration. Replacement fluids may consist of standard solutions of bicarbonate, potassium-free LR solution, acetate, or dextrose. Electrolytes such as potassium, sodium, calcium chloride, magnesium sulfate, and sodium bicarbonate may be added. The formula used to calculate the volume removed from the patient follows with an example:
< ?xml:namespace prefix = "mml" />
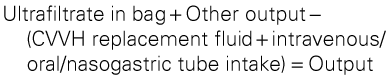

Continuous Venovenous Hemodialysis
CVVHD is technically like traditional hemodialysis, and it removes solute by diffusion because of a slow (15 to 30 mL/min) countercurrent drainage flow on the membrane side of the hemofilter (see Figure 20-6, C). Blood and fluid move by countercurrent flow through the hemofilter. Countercurrent means the blood flows in one direction and the dialysate flows in the opposite direction. As with other types of CRRT and hemodialysis, although arterial access is always possible, venovenous vascular access is the most common choice.
CVVHD is indicated for patients who require large-volume removal for severe uremia or critical acid-base imbalances or for those who are resistant to diuretics. An MAP of at least 70 mm Hg is desirable for effective volume removal and dialysis, and it is most effective when used over days, not hours. The use of replacement fluid is optional and depends on the patient’s clinical condition and plan of care. The critical care nurse is responsible for calculating the hourly intake and output, identifying fluid trends, and replacing excessive losses. This therapy is ideal for hemodynamically unstable patients in the critical care setting because they do not experience the abrupt fluid and solute changes that can accompany standard hemodialysis treatments.
Continuous Venovenous Hemodiafiltration
Another CRRT option is CVVHDF, which combines two of the previously described methods (CVVH and CVVHD) to achieve maximal fluid and solute removal (see Figure 20-6, D). A strong transmembrane pressure is applied to the hemofilter to push water across the filter, and a negative pressure is applied at the other side to pull fluid across the membrane and produce large volumes of ultrafiltrate and to create a “solvent drag” (CVVH method). The blood and the dialysate are circulated in a countercurrent flow pattern to remove fluid and solutes by diffusion (hemodialysis method). CVVHDF can remove large volumes of fluid and solute because it employs diffusion gradients and convection.
Complications
Potential problems associated with CRRT and appropriate nursing interventions are listed in Table 20-11. Complications are often related to the rate of flow through the system. If the patient becomes hypotensive or the access lines remain kinked, the ultrafiltration rate will decrease. This can lead to increased clot formation within the hemofilter. As the surface of the hemofilter becomes more clotted, it will not provide effective fluid or solute clearance, and CRRT will be stopped; a new CRRT circuit must then be set up. The critical care nurse keeps track of the pressures displayed on the CRRT machine screen to monitor the positive pressure of fluid going into the hemofilter (inflow) and the pressures coming out of the hemofilter to ensure that that resistance to the negative-pressure pull of the fluid across the hemofilter membrane has not developed. Other patient-related complications include fluid and electrolyte alterations, bleeding because of anticoagulation, and problems with the access site, such as dislodgement and infection.
TABLE 20-11
COMPLICATIONS ASSOCIATED WITH CONTINUOUS RENAL REPLACEMENT THERAPY
PROBLEM | CAUSE | CLINICAL MANIFESTATIONS | NURSING MANAGEMENT |
Decreased ultrafiltration rate | |||
Filter clotting | |||
Hypotension | |||
Fluid and electrolyte changes | |||
Bleeding | |||
Access dislodgement or infection |
ACT, activated coagulation time; ECG, electrocardiogram; ↑, increased; ↓, decreased.
Medical Management
The choice of the method of blood purification to use to treat AKI is a medical decision. There is no clear clinical or research consensus about whether IHD or CRRT is the most beneficial. Age, gender, and preexisting chronic conditions are of little help in determining the need for hemofiltration or hemodialysis. Often, the acute clinical diagnosis, physician’s preference, availability of the CRRT machine, and knowledgeable physicians and nurses at the hospital are the deciding factors. Infectious complications are associated with a grave prognosis. Dialysis is prescribed for almost anyone who develops severe AKI, unless the patient is clearly dying.
IHD or CCRT is usually begun before the BUN level exceeds 90 mg/dL or the creatinine level exceeds 9 mg/dL. In many hospitals, the threshold to begin treatment is considerably lower. Whether daily treatment is more effective than treatment every other day is controversial. The patient’s serum creatinine concentration, BUN level, and fluid volume status are the deciding factors. CCRT is often prescribed when the BUN level is approximately 60 mg/dL. CRRT is more effective in the early stages of AKI. If the patient has severe electrolyte imbalance or fluid overload, even earlier intervention may be required.
Nursing Management
Critical care nurses play a vital role in monitoring the patient receiving CRRT. In many critical care units, the CRRT system is set up by the dialysis staff but is run on a 24-hour basis by critical care nurses with additional training. Complications may be related to the CRRT circuit, the CRRT pump, or to the patient, as shown in Box 20-6. The critical care nurse monitors fluid intake and output, prevents and detects potential complications (e.g., bleeding, hypotension), identifies trends in electrolyte laboratory values, supervises safe operation of the CRRT equipment, and provides patient and family education about the patient’s condition and the use of CRRT.
Kidney Transplantation
When the kidney to be transplanted is procured from the donor, whether living or cadaveric, the ureter, renal vein, and renal artery are dissected, leaving as much length as possible.
Living Donor Surgery
Living kidney donors are typically related to the person who will receive the kidney. The procurement can be a laparoscopic procedure or an open procedure. After the kidney is secured, it is flushed with a cold electrolyte preservative solution until the venous return is clear.90 This usually requires 100 to 200 mL of solution.90 The kidney is then transported to the operating room to be transplanted.
Deceased Donor Surgery
If the kidney is from a deceased donor, it is flushed with a cold electrolyte preservative solution and simultaneously cooled externally as quickly as possible. It can be transported on a kidney perfusion machine or packed in an iced preservation solution. After it is procured and placed in the hypothermic solution, it can be maintained for 48 to 72 hours before it must be transplanted.90 Most transplant centers attempt to transplant an organ within 24 hours after procurement, to minimize the risk of kidney injury.90 At the time of procurement, the kidney is assessed in situ for color, shape, and form. It is palpated to determine firmness, and often a biopsy specimen is taken to rule out undiagnosed kidney dysfunction.
Recipient Surgery
The patient is anesthetized in the usual manner, and a urinary catheter is placed. A curvilinear incision is made 3 to 4 cm above the symphysis pubis and extended to the iliac crest (Figure 20-7, A). The kidney will be placed in the extraperitoneal space of the right or left iliac fossa. The muscles and fascia are divided and retracted medially to expose the iliac vessels. The renal artery is anastomosed to the external iliac artery, using an end-to-side or an end-to-end anastomosis, and the vein is sutured to the common iliac vein in a similar manner (Figure 20-7, B).90
During the procedure, a CVP ranging from 8 to 16 mm Hg must be maintained, and a systolic BP at least as high as the patient’s baseline value should be maintained to ensure adequate perfusion of the transplanted kidney.
Postoperative Medical Management and Nursing Care
After the transplantation is completed and the patient is stable and ready for discharge from the recovery room, most transplant centers admit the patient directly to the organ transplantation unit or to the critical care unit. Serious complications can occur in the immediate postoperative period, and a sound knowledge base of medical/surgical nursing, kidney function, anatomy, and immunosuppressive medications is imperative.
Fluid Status
If the transplanted organ is working well, the patient’s fluid status, as monitored by observations of CVP, weight, and vital signs, must be regulated very closely. Adequate hydration is an absolute necessity for continued graft function in the immediate postoperative period. Hypovolemia can lead to compromised blood flow to the kidney, acute kidney injury, and possible graft failure. The new kidney will be producing large amounts of urine, and fluid replacement, usually maintained in a 1 : 1 ratio, must be sustained.
Electrolytes
Electrolyte balance is also of grave concern. Because of the large volumes of urine produced, the potential exists for hypokalemia, hypomagnesemia, and hypocalcemia, leading to possible cardiac compromise.91 Electrolytes must be monitored at least every 4 to 6 hours and replaced as necessary. Assessment of the BUN and the creatinine concentration also is necessary every 4 to 6 hours to monitor graft function and determine the need for dialysis.
Postoperative Complications
The complete blood count and platelet count should be monitored every 4 to 6 hours. Blood loss during the operation is minimal, usually 500 mL or less. Abrupt decreases or continuously falling counts may indicate hemorrhage at an anastomosis site, which requires a return to the operating room for repair. Even with minimal blood loss during surgery, transfusion of blood products after the surgery is often necessary. Frequent observation and assessment of the surgical incision are needed to evaluate for drainage and swelling. Urine output volume and color should be monitored at least every 30 minutes. The bladder anastomosis is fragile, and it is not uncommon for clots to occlude the catheter. The bladder must remain decompressed for several days to promote proper healing. If clots occlude the end of the catheter, gentle irrigation or aspiration may be necessary. If the clot cannot be dislodged or aspirated out, the catheter may have to be changed. Painful bladder spasms also can occur, and opiates, usually in the form of a belladonna and opium suppository, may be required to relax the bladder.
Immunosuppression
Initiation of induction immunosuppressant therapy begins at the time of transplantation, usually in the form of a polyclonal antithymocyte/antilymphocyte intravenous compound or an intravenous monoclonal antibody compound. These compounds remove the lymphocytes from the patient’s system, preventing rejection and suppressing the immune system until oral drugs can be safely administered and blood drug levels are sufficient to allow discontinuation of the intravenous agent. Because the patient is now immunocompromised, strict aseptic technique is required to prevent infection.
Infection Risk
Thorough hand washing, aseptic dressing changes, discontinuation of any unnecessary invasive lines, and limiting the number of visitors are necessary protective measures. Because of the patient’s immunocompromised status, subtle changes in the temperature, WBC count, or wound drainage can signal an active infection. Patients also are susceptible to infection by opportunistic native organisms such as Candida, Pneumocystis pneumonia, cytomegalovirus, Epstein-Barr virus, and herpes simplex virus.
The average length of stay in the hospital after uncomplicated kidney transplantation is 5 to 7 days.92 During the first few days, the patient must learn self-care related to the new organ. Medication regimens are very complicated, and most centers initiate a self-medication program at the patient’s bedside as a training tool. Patients are taught the signs and symptoms of infection and graft rejection, the protocols of the transplant clinic, and new dietary limitations. Frequent transplant clinic visits to check the functioning of the organ and to adjust down the doses of immunosuppressant medications are necessary for the first few months after transplantation.92
Rejection
Rejection of the transplanted kidney represents an ongoing concern for all transplant patients. The graft function is monitored closely, and if rejection is suspected, a biopsy is performed. If the biopsy reveals acute rejection, rescue therapy is initiated. This therapy can be given in the form of high-dose intravenous steroids for mild rejection or intravenous monoclonal antibody for moderate to severe rejection. If the biopsy reveals chronic rejection, the oral immunosuppressant medications are increased or returned to the higher doses used immediately after transplantation. No two patients’ immune systems are exactly alike, and the immunosuppressant medication regimen required to prevent rejection must be tailored to each patient individually. The goal is to create a balance among medications that allows the patient to fight off most infections but avoid rejection of the transplanted organ.
Patient adherence to the complicated medical regimen that is required to maintain a transplanted organ is of major concern. Adequate education of the patient and family as to the importance of taking the medications as instructed is of paramount importance. Patients are reluctant to take the medications appropriately if they are experiencing severe or disfiguring side effects. Decreasing the doses of the medications can often alleviate these side effects but may lead to a rejection episode. The goal is to create a balance so that medications allow the patient to fight off most infections and yet avoid rejection of the transplanted kidney.