CHAPTER 97 Reconstruction of the Mandible
Major advances in patient care generally occur through paradigm shifts (Fig. 97-1). For reconstruction of the mandible and maxilla, the first major paradigm shift occurred with the development of reliable free tissue transfer options along with advances in hardware devices. Before these advances, techniques involving nonvascularized bone grafts, vascularized pedicled bone grafts, and alloplastic materials were not reliably successful for primary reconstruction, especially in the typical defects that were caused by tumor-ablative surgery.1,2 Both advances in surgical techniques and improved hardware options were crucial for this first paradigm shift, which occurred circa 1990 for mandibular reconstruction and circa 2000 for maxillary reconstruction. Although current options have proved to be reliably successful for single-stage primary reconstruction in the mandible and maxilla, these procedures remain highly complex and challenging, and donor sites are still required. In addition, it is difficult to restore the intricate three-dimensional structure precisely in many defects with bone from distant sites because of differences between the size and shape of the bone graft and the defect configuration. Advances in biotechnology, specifically in the field of tissue engineering, will probably have an effect on mandibular and maxillary reconstruction and will cause another paradigm shift within the foreseeable future.3
Anatomy, Physiology, and Pathophysiology
The mandible is the strongest facial bone.4 It is a U-shaped bone with a horizontal portion (body), which carries an alveolar process with teeth, and two vertical portions (rami), which, through the temporomandibular joints, articulate with the skull bilaterally (Fig. 97-2). The mandible is attached to other facial bones by ligaments and muscles, and it articulates with the maxilla through occlusion of the teeth.
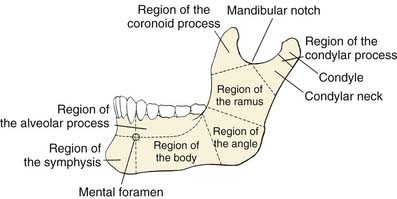
Figure 97-2. Anatomic regions of the mandible.
(From Dingman RO, Natvig P. Surgery of Facial Fractures. Philadelphia: WB Saunders; 1964.)
The body of the mandible has a dense cortical structure on the lateral and medial surfaces with a small core of spongiosa that contains nerves, blood vessels, and lymphatic vessels. The lower portion of the body contains heavy, thick bone that changes very little throughout life. The upper portion of the body, called the alveolar process, contains the dental sockets that support the teeth. Changes in the alveolar process occur throughout adult life, especially after dental extractions, which cause subsequent atrophy of this bony segment (Fig. 97-3). The mental foramen is midway between the inferior border and upper edge of the alveolar process, at the level of the second premolar tooth. The mental nerve branch of the inferior alveolar nerve and the associated vessels pass through this foramen. In an edentulous mandible, the mental foramen drifts to the upper border of the bone as a result of atrophy of the alveolar process (see Fig. 97-3).
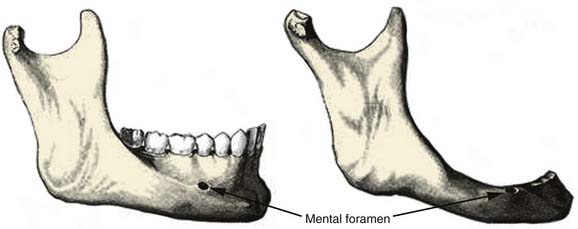
Figure 97-3. Comparison of the anatomy of a dentulous mandible (left) with an edentulous mandible (right).
(From Dingman RO, Natvig P. Surgery of Facial Fractures. Philadelphia: WB Saunders; 1964.)
The movement of the mandible is dependent primarily on two groups of muscles. The depressor-retractor group, which is anterior, is the weaker of the two groups and is composed of the geniohyoid and digastric muscles. These muscles insert into the inferior inner aspect of the symphyseal region and exert downward posterior force. The elevator group, which is posterior, is composed of the masseter, medial pterygoid, and temporalis muscles. The masseter muscle inserts along the outer aspect of the inferior edge of the angle. The medial pterygoid muscle inserts on the inner surface at the mandible angle, thus producing the masseteric-pterygoid sling. The masseter and medial pterygoid muscles always act simultaneously and hold each other in balance, but the angulation of the attachments at the angle is more favorable for the medial pterygoid. Thus, although the medial pterygoid muscle is weaker than the masseter in the intact mandible, it usually overpowers the masseter in segmental defects as a result of this more favorable angulation; this accounts for the typical displacement patterns that occur. Other muscles with more minor influence on the movements of the mandible include the lateral pterygoid and mylohyoid muscles. The lateral pterygoid muscle inserts mostly on the anterior inner aspect of the condylar neck and pulls primarily the neck medially and anteriorly. The mylohyoid muscle has minimal influence on movements of the intact mandible, inasmuch as most of its bundles insert into the muscle’s midline raphe rather than onto the mandible.4
The damage to the oral cavity after tumor extirpation was described by Conley in 1962.5 Options for bone or soft tissue reconstruction were limited at that time, but the serious effects of extirpative surgery on function and cosmesis were clearly recognized. Functions of the oral cavity structures include mastication, swallowing, maintenance of oral competence, articulation, taste, oral hygiene, and airway protection.6 Effective reconstruction of the oral cavity soft tissue components is crucial for functional restoration, especially in preserving mobility, position, and shape of the tongue, which is probably most critical for functional rehabilitation.6
There are two types of mandibulectomies: marginal and segmental (Fig. 97-4). In a marginal mandibulectomy, a rim of bone is resected, but continuity of the mandibular arch is maintained. When this type of mandibulectomy is performed, a curvilinear excision configuration must be used, and a minimal 1 cm height of inferior bone must be preserved, in order to avoid iatrogenic fracture.7,8 The bone defect in a marginal mandibulectomy does not necessitate any specific reconstruction except for soft tissue coverage, usually with a soft tissue flap or skin graft.9 The reconstructive plan in this setting is directed by the characteristics of the soft tissue defect and influenced mostly by the extent of soft tissue loss and whether the tissue has been previously irradiated.
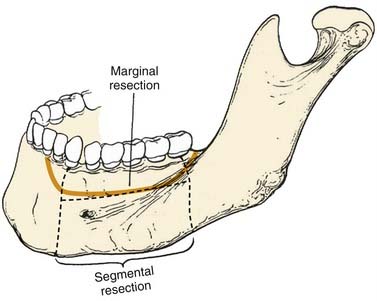
Figure 97-4. Techniques of mandibulectomy: marginal and segmental.
(From Silver CE, Rubin JS. Atlas of Head and Neck Surgery. 2nd ed. London: Churchill Livingstone; 2002.)
Segmental mandibulectomy involves resection of a full thickness of bone, which creates a discontinuity defect (see Fig. 97-4). Significant cosmetic and functional morbidity are associated with segmental defects. For defects involving the anterior mandible (between both mental foramina), the lateral segments act independently, and the displacement pattern is influenced by the remaining attached muscles (Fig. 97-5).10,11 The medial pterygoid muscle overpowers the masseter and pulls the segments upward, posteriorly, and medially. This movement brings the teeth out of alignment and disrupts mastication. There is complete loss of projection of the lower midline third of the face, which causes an overhanging upper jaw, the so-called Andy Gump appearance. The tongue loses anterior support, thus becoming malpositioned with posterior displacement. The oral sulci become obliterated. The upward movement of the larynx is lost with swallowing as a result of detachment of the suprahyoid musculature. The lower lip is numb as a result of resection of the mental nerves. All these changes lead to a cosmetically and functionally disabling situation for the patient. For defects involving the lateral mandible (posterior to the mental foramina), the remaining bone deviates toward the defect through the action of the remaining muscles of mastication, primarily the medial and lateral pterygoid muscles (Fig. 97-6).10,11 This causes malalignment of the teeth, which affects mastication. The deformity is accentuated by opening the mouth or chewing.
Classification of Defects
A combination of the extent of bone and soft tissue loss determines the reconstructive method. Classification systems that account for this fact are useful. Various classification schemes that have been described for segmental mandibulectomy defects can be used for documenting the defect components, which helps with planning the reconstruction (Fig. 97-7). In Boyd and colleagues’12 classification, H defects are lateral defects of any length up to the midline that include the condyle; L defects are lateral defects that exclude the condyle; and C defects involve the central segment containing the four incisors and two canines. The three lowercase letters in this classification system describe the associated soft tissue components: o (no skin or mucosal component), s (skin), m (mucosa), and sm (skin plus mucosa). Boyd and colleagues recognized the reconstructive and functional problems posed by the absence of the condyle and mentum; thus, their system is similar to Pavlov’s classification system, described before the era of free tissue transfer.12 With loss of the condyle, reconstruction of an articular surface is difficult. Loss of the central segment poses problems with restoration of stomal competence, restoration of an anterior gingivolabial sulcus to fit prosthetics for dental rehabilitation, and restoring the lip height for cosmesis.
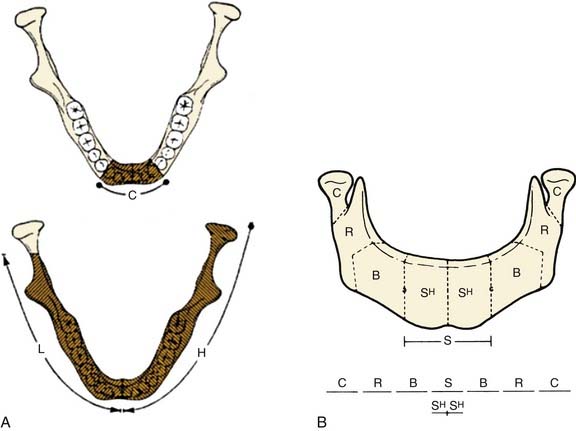
Figure 97-7. Systems of classification of mandible defects described by Boyd and colleagues12 (A) and Urken and associates13 (B).
(A, From Boyd JB, Gullane PJ, Rotstein LE, et al. Classification of mandibular defects. Plast Reconstr Surg. 1993;92:1266-1275. B, From Urken ML, Weinberg H, Vickery C, et al. Oromandibular reconstruction using microvascular composite free flaps. Report of 71 cases and a new classification scheme for bony, soft tissue and neurologic defects. Arch Otolaryngol Head Neck Surg. 1991;117:733-744.)
Another classification scheme, described by Urken and associates,13 is similarly based on functional considerations caused by the detachment of different muscle groups and difficulties with cosmetic restoration. This reconstruction scheme has similar anatomic designations, including separate components of the condyle (C), ramus (R), body (B), total symphysis (S), hemisymphysis (SH), and palate (P). Urken and associates’ classification system also includes a detailed description of soft tissue and neurologic deficits.
Neither of these two classification systems describes the absolute length of the defect. Before the use of free tissue transfer, the chance of healing and bony union depended more significantly on size when nonvascularized bone grafts were used. When osseous free flaps are used, outcomes are less dependent on the length of the graft required to bridge the defect.12
Goals of Mandibular Reconstruction
The primary objective of tumor resections that necessitate mandibulectomy is cure, but the functional and cosmetic rehabilitation are crucial for the physiologic and psychologic well-being of patients. The ideal reconstruction for a segmental mandibulectomy (1) restores oral competency, (2) maintains occlusal relationships with remaining teeth, (3) allows for prosthetic dental restoration, (4) restores bone continuity, and (5) restores facial symmetry and contour to the lower third of the face.14 In addition, wound closure should be immediate and complete in order to maximize early functional recovery, avoid orocutaneous fistula, avoid infection, create a safe wound that can undergo postoperative radiation 6 weeks later, and facilitate early hospital discharge. To achieve these goals, basic principles of reconstructive surgery dictate that the simplest method to achieve the desired results should be chosen for each individual patient.
Background and History
Before the development of advances in surgical techniques and improvements in hardware, reconstructive options were limited and yielded disappointing results. The available options for bone replacement at that time included nonvascularized autogenous bone grafts, allogeneic bone grafts, and vascularized pedicled bone grafts.11 The failure rate of vascularized pedicled bone grafts was as high as 50%.1 Nonvascularized bone grafts, which rely on neovascularization, were found to be prone to infection, extrusion, or resorption when placed into defects in which the oral mucosa was violated or in the setting of perioperative irradiation. Hardware options included alloplastic materials such as trays, prostheses, and Kirschner wires. These devices were designed to prevent displacement of the remaining mandibular segments, support the surrounding soft tissue, and minimize wound contracture.15 Unfortunately, these devices yielded disappointing results because of loosening, migration, exposure, and fracture.
Many studies, including those published by Adamo and Szal,2 Lawson and Biller,1 and Komisar,16 consistently revealed poor results of mandibular reconstruction with the available approaches and technologies. Mandible reconstruction usually proceeded in multiple stages fraught with complications. The management of these complications increased the number of hospitalizations by an average of three per patient.16 These complications sometimes delayed the postoperative treatment with radiation, which affected the ability to cure the cancer. Primary reconstruction yielded the best results but had the highest rate of complications. Secondary reconstruction produced fewer complications but suboptimal results.1,2,16,17 In the secondary setting, soft tissue contracture and fibrosis caused by prior irradiation would fixate the remaining bone segments in a malpositioned orientation, and the fibrosis of the remaining muscles of mastication accentuated this problem.
Even with the successful reconstructions, although most patients demonstrated cosmetic improvement, few experienced significant functional benefits.15–17 The functional limitations resulted from misalignment of the mandible, trismus caused by fibrosis, soft tissue losses, and failure of a bony segment capable of supporting a dental prosthesis. Gullane15