CHAPTER 73 Rationale for Surgical Interventions in Movement Disorders
Neuroscience research has led to major insights into the structure and function of the basal ganglia and the pathophysiologic basis of basal ganglia disorders such as Parkinson’s disease (PD).1–4 Several factors have contributed to progress in this field, specifically, the availability of effective animal models, improved methods of physiologic and pharmacologic investigation, and the development of powerful brain imaging methods for studies of patients and animal models of these disorders.
Overview of Anatomy and Function of the Basal Ganglia
PD and other movement disorders are increasingly being recognized as “circuit disorders” that involve not only the basal ganglia but also related nodes in the thalamus and the cerebral cortex. This chapter focuses on a description of these larger cortical-subcortical circuits. The anatomic organization of the basal ganglia is discussed in detail in Chapter 74.
Anatomic and physiologic studies have demonstrated that the basal ganglia are components of a family of parallel reentrant brain circuits in which cortical information is sent to the basal ganglia, processed, sent to the thalamus, and then returned to the cerebral cortex.5–9 The different circuits are segregated but share anatomic features, thus supporting the view that the basal ganglia and cortex interact in a modular fashion in which similar processing steps are carried out in each module even though the different modules are engaged in different functions. Depending on the presumed function of the cortical region that is involved in these different circuits, the circuits are commonly designated as “motor,” “oculomotor,” “prefrontal,” and “limbic” (Fig. 73-1). As shown in Figure 73-1, dysfunction within the individual circuits may be associated with specific disease states.
Movement disorders prominently affect the “motor” circuit, which takes origin in the frontal cortical precentral and postcentral sensorimotor areas, including the primary motor cortex (M1), the supplementary motor area (SMA), the premotor cortex (PMC), the cingulate motor area (CMA), and interconnected sensory cortical areas, and involves specific “motor” portions in each of the basal ganglia structures and thalamus. Similar to the other transbasal ganglia circuits, the motor circuit is at least partially closed, with thalamocortical projections terminating in the same frontal cortical regions from which the circuit originates. The anatomy of the motor circuit is shown in greater detail in Figure 73-2A.
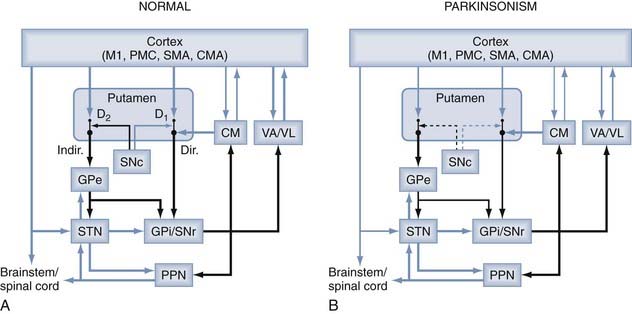
FIGURE 73-2 Parkinsonism-related changes in overall activity in the basal ganglia–thalamocortical motor circuit. Black arrows indicate inhibitory connections; blue arrows indicate excitatory connections. The thickness of the arrows corresponds to their presumed activity. CM, centromedian nucleus; Dir., direct pathway; D1, D2, dopamine receptor subtypes; Indir., indirect pathway; PPN, pedunculopontine nucleus; SNc, substantia nigra pars compacta; STN, subthalamic nucleus. For other abbreviations, see the legend to Figure 73-1.
(From Galvan A, Wichmann T. Pathophysiology of parkinsonism. Clin Neurophysiol. 2008;119:1459.)
The striatum and STN are the main entry points for cortical input, whereas the GPi and SNr provide basal ganglia output to the thalamus. The corticostriatal projections are topographically organized.5–7,10,11 Movement-related cortical input terminates in the postcommissural putamen, and nonmotor projections terminate in other areas of the striatum. Thus, prefrontal cortical areas project to the caudate nucleus and the precommissural putamen, and projections from limbic cortices, amygdala, and hippocampus terminate preferentially in the ventral striatum. The cortical-subthalamic projections are also topographically arranged.12,13 Afferents from M1 reach the dorsolateral STN, whereas afferents from the PMC and SMA innervate mainly the mediodorsal third of the nucleus.14
The striatum is linked to the basal ganglia output structures, GPi and SNr, via two distinct pathways, the so-called direct and indirect pathways (Fig. 73-2).1,5,7 The direct pathway arises from sets of striatal medium spiny neurons (MSNs) that project monosynaptically to neurons in the GPi and SNr. These neurons also contain the neuropeptides substance P and dynorphin and preferentially express dopamine D1-like receptors. It is thought that MSNs in the direct pathway receive a larger share of the thalamostriatal pathway than do neurons in the indirect pathway. The indirect pathway arises from a set of striatal MSNs that project to the GPe.15,16 The striatal neurons that give rise to the indirect pathway preferentially express enkephalin and dopamine D2-like receptors.17,18 Although most authors emphasize the separation of these pathways, single-cell labeling of striatal MSNs has shown that at least some send collaterals to both segments of the globus pallidus (thus participating anatomically in both the direct and indirect pathways).19,20
Similar to the corticostriatal projections, the direct and indirect pathways are topographically organized. For instance, populations of GPe neurons within the sensorimotor, associative, or limbic territory are reciprocally connected with populations of neurons in the same functional territories of the STN, and neurons in each of these regions, in turn, innervate the same functional territory of the GPi.15,16
In terms of basal ganglia output, the caudolateral “motor” territory of the GPi projects almost exclusively to the posterior part of the ventrolateral (VL) nucleus, which sends projections toward the SMA,21,22 M1, and PMC.23 The outflow from pallidal motor areas directed at M1, PMC, and SMA appears to arise from separate populations of pallidothalamic neurons.23 These findings indicate that the larger “motor circuit” is composed of segregated subcircuits centered on the individual cortical motor areas.24,25 The more rostromedial associative areas of the GPi project preferentially to the parvocellular part of the ventral anterior (VA) and the dorsal VL nucleus (VLc in macaques),26,27 specifically to thalamic areas preferentially connected to the prefrontal cortex.28,29
Collaterals from the pallidofugal and nigrofugal projections also reach the intralaminar nuclei of the thalamus, the centromedian (CM) and parafascicular (PF) nuclei. These projections are part of a system of segregated basal ganglia–thalamostriatal feedback projections.27,30 In primates, the CM nucleus receives input from motor areas in the GPi and projects to the motor portions of the putamen and STN, whereas PF input and output are related to the associative and limbic territories of the basal ganglia.31,32 CM terminations are found mostly along the shafts of striatal direct pathway MSNs,33,34 separate from the sites of termination of cortical input and of dopaminergic synapses.30,35–41 Basal ganglia output also reaches the pedunculopontine nucleus (PPN),42,43 which in turn gives rise to ascending projections to the basal ganglia, thalamus, and basal forebrain and to descending projections to the pons, medulla, and spinal cord.44,45
Electrophysiologic and metabolic mapping studies in animals and functional imaging data in humans support the view that the anatomically and physiologically defined basal ganglia “motor” areas are indeed involved in movement.25,46–48 The model of the basal ganglia–thalamocortical motor circuit shown in Figure 73-2 is often used as the basis for speculations regarding the function of the basal ganglia in movement. The model poses that activation of MSNs that give rise to the direct pathway reduces inhibitory basal ganglia output from targeted neurons with subsequent disinhibition of related thalamocortical neurons.5,49,50 The net effect of this process is increased activity in appropriate cortical neurons that results in facilitation of the movement. By contrast, activation of MSNs that give rise to the indirect pathway would lead to increased (inhibitory) basal ganglia output on thalamocortical neurons and to suppression of movement. The balance between direct and indirect pathway activity may regulate the overall amount of movement, whereas specific activation patterns (for instance, a center-surround type of activation involving the direct and indirect pathways) may limit the extent or duration of ongoing movements.
Other views of basal ganglia function are currently evolving.51–55 Thus, synaptic processing and modification of synaptic strength in the striatum, or alteration of the level of interneuronal synchrony, may give the basal ganglia a role in the regulation of habit formation or procedural learning.51–5355
In models of basal ganglia function, the modulatory effects of dopamine on striatal transmission are highly important. Dopamine is released in the striatum from terminals of projections from the SNc and modulates the activity of the basal ganglia output neurons in the GPi and SNr by facilitating corticostriatal transmission on the direct pathway and inhibiting corticostriatal transmission on MSNs that give rise to the indirect pathway. These opposing actions are probably mediated by the two different sets of dopamine receptors (D1-like and D2-like receptors) that are differentially expressed in these pathways.56–59 Through the different effects of activation of the direct and indirect pathways, the net effect of striatal dopamine release appears to be reduction of basal ganglia output to the thalamus and other targets. Evidence indicates that dopamine also acts directly on receptors in the STN and pallidum to influence discharge patterns and rates in these structures.
Dopamine receptor activation may not only act to modulate the activity of the direct and indirect pathways but might also have a role in the proposed learning functions of the basal ganglia. Activation of dopamine receptors on MSNs has been shown to be involved in the induction of long-term potentiation and depression at glutamatergic (presumably corticostriatal) synapses.60–64 Recent research has elucidated complex interactions between dopaminergic transmission and transmission involving adenosine, glutamate receptors, and endocannabinoid receptors in this process.61,63–66
Parkinsonism
The cardinal features of PD—the triad of akinesia/bradykinesia, tremor at rest, and muscular rigidity—result from decreased dopaminergic transmission in the motor portions of the basal ganglia, in particular, the putamen, as a result of progressive loss of dopaminergic neurons in the SNc. Dopaminergic replacement therapies are highly effective in reversing these features of the disorder. These dopamine-responsive features are often accompanied by other issues that are poorly or entirely unresponsive to dopaminergic medications, such as depression, autonomic dysfunction, sleep disorders, cognitive impairment, and gait/balance problems. Although some of these problems may result, in part, from decreased dopamine within the nonmotor portions of the basal ganglia, widespread progressive pathologic changes in the brainstem, thalamus, and eventually the cerebral cortex appear to play a major role.67
Pathophysiology of Parkinsonism
The following paragraphs are focused on the motor aspects of PD that result from dopamine deficiency. Study of these changes has been facilitated by the development of animal models of dopamine depletion in which changes in the basal ganglia, thalamus, and cerebral cortex can be conveniently studied, including the 6-hydroxydopamine (6-OHDA)-treated rat and primates treated with 1-methyl-4-phenyl-1,2,3,6-tetrahydropyridine (MPTP).68,69
In early studies of MPTP-treated primates, alterations in activity of the striatopallidal pathways were strongly emphasized. Such changes were suggested by studies of metabolic activity in the basal ganglia that indicated increased synaptic activity in the GPe and GPi.70,71 Possible interpretations of this finding were that the activity of the striatal-GPe connection and the STN-GPi pathway is increased in parkinsonism or that the STN projections to both pallidal segments were overactive. Subsequent microelectrode recording studies in MPTP-treated primates showed a reduction in neuronal discharge in the GPe and increased firing in the STN, GPi, and SNr in comparison to normal controls,72–75 as well as high neuronal discharge rates in the GPi in PD patients undergoing functional neurosurgical procedures.76–78 These changes may be related to one another in that striatal dopamine depletion may lead to increased activity of striatal neurons in the indirect pathway and result in inhibition of the GPe and subsequent disinhibition of the STN and GPi/SNr (Fig 73-2B). A role of local dopamine loss in the extrastriatal structures (specifically in the STN, GPi, and SNr) may play a role as well, specifically with regard to the emergence of abnormal firing patterns in these nuclei (see later).
Other structures and feedback loops, such as those involving the PPN and CM, may contribute to the abnormalities in discharge that are found in the basal ganglia output nuclei. A series of studies have demonstrated that brainstem areas such as the PPN may also be involved in the development of parkinsonian signs. Lesioning of the PPN in normal monkeys was shown to lead to akinesia.79,80 At the cortical level, positron emission tomography (PET) studies in parkinsonian patients have consistently shown reduced activation of motor and premotor areas.46,81
The notion that rate changes in the basal ganglia underlie the development of parkinsonism (the so-called rate model, depicted in Fig. 73-2) is generally supported by studies that have shown that inactivation of the sensorimotor portions of the STN or GPi increases metabolic activity in the cortical motor areas and improves bradykinesia and tremor in parkinsonian subjects. Thus, lesions of the STN, GPi, or SNr in MPTP-treated primates reverse some or all signs of parkinsonism, presumably by reducing basal ganglia output,19,82,83 and GPi or STN lesions are highly effective antiparkinsonian treatments in patients with PD.84–90 However, the “rate model” has largely been rejected because several crucial observations contradict a purely rate-based view of basal ganglia–processing abnormalities. For instance, lesions of the VA/VL nuclei of the thalamus (which completely removes thalamic output) do not lead to parkinsonism and are, in fact, beneficial in the treatment of tremor and rigidity.91,92 Similarly, lesions of the GPi in the setting of parkinsonism improve the cardinal features of PD without producing dyskinesias or other obvious detrimental effects. In fact, they are highly effective in reducing drug-induced dyskinesias.86,87,93
Because of these discrepancies, the rate model has given way to the view that abnormalities in basal ganglia activity other than rate changes are more relevant to the pathophysiology of parkinsonism. Among the most discussed changes in discharge patterns in the basal ganglia of parkinsonian subjects (Fig. 73-3) is the development of oscillatory phenomena.94,95 Abnormal oscillations have been identified in the activity of single neurons in the GPi, SNr, and STN in animals and patients and, more recently, in recordings of local field potentials (LFPs) in patients, and these oscillations may reflect the activity of more extensive networks of connections. The LFP recordings were made by using implanted DBS macroelectrodes as recording probes during the time immediately after the implantation surgery. Recordings in the STN and other basal ganglia areas with this method have demonstrated the presence of LFP oscillations in the 10- to 25-Hz (beta) range in the STN, GPi, and cortex in unmedicated PD patients. The studies have also shown that beta-range oscillations give way to oscillations in the 60- to 80-Hz (gamma) range when the patients are treated with levodopa or when the STN is stimulated at high frequencies.95,96 The prominence of beta-band activity is apparent throughout the basal ganglia–thalamocortical circuitry. For example, the desynchronization of electroencephalography (EEG) oscillations that normally precedes movement was shown to be abnormally small in parkinsonian patients, perhaps interfering with the initiation of movement.97–99
Another parkinsonism-related abnormality in spontaneous discharge is the emergence of abnormal synchrony between neurons. Under physiologic conditions, basal ganglia activity is highly specific in its relation to movement parameters and body part and appears to be segregated even at the cellular level, where neighboring neurons are rarely found to fire in synchrony.100 In parkinsonism, this level of segregation is lost, and the discharge of neighboring neurons is often found to be correlated and abnormally synchronized.95,101
Finally, the proportion of cells in the STN, GPi, and SNr that discharge in bursts (oscillatory or nonoscillatory) is greatly increased in parkinsonism.73,95,101–103 Oscillatory burst discharge patterns are also seen in conjunction with tremor, which may reflect tremor-related proprioceptive input or more active participation of basal ganglia in the generation of tremor.
Although spontaneous basal ganglia firing has been extensively studied in MPTP-treated monkeys, much less is known about the way information processing is altered in the basal ganglia. Studies of changes in neuronal firing patterns preceding or following active movements are difficult to perform and interpret in akinetic or bradykinetic animals. However, studies of neuronal responses to passive limb manipulation have been carried out and showed that such responses in the STN, GPi, and thalamus occur more often, are more pronounced, and have widened receptive fields after treatment with MPTP.72,73,101,104
The importance of specific electrophysiologic features in basal ganglia, thalamic, or cortical activity for development of the behavioral signs of parkinsonism remains unclear. Although burst-like, synchronized oscillatory activity in the basal ganglia–thalamocortical circuits is associated with parkinsonism,105 direct links between oscillatory activity and specific parkinsonian deficits have not been established. In fact, recent studies in monkeys that underwent a gradual MPTP treatment protocol in which parkinsonism was slowly induced have cast doubt on the notion that synchronous oscillatory firing contributes strongly to (early) parkinsonism.106 In these single-neuron recording studies, synchrony and oscillations in neuronal spiking activity were detected only after the development of bradykinesia and akinesia. Similarly, recent rodent experiments have suggested that abnormal oscillations in the basal ganglia do not result simply from (acute) lack of dopaminergic stimulation but may rather be due to the chronic absence of dopamine.107 Such “late” changes could be related to anatomic alterations secondary to dopamine depletion, such as loss of the dendritic spines of MSNs,108–110 which has been described as homeostatic pruning in response to altered glutamatergic transmission in the striatum.108,109
Rationale for Treating Parkinsonism by Surgical Interventions
Two general types of procedures are used to surgically treat patients with parkinsonism: lesioning and DBS. Unilateral lesioning of the sensorimotor territory of the GPi results in significant contralateral antiparkinsonian effects and significantly reduces drug-induced involuntary movements and motor fluctuations. For DBS, a stimulation electrode is introduced into the same targets in the GPi and STN, and high-frequency stimulation is applied by way of an implanted, externally programmable pulse generator. DBS alleviates parkinsonian motor signs and reduces the severity of “off” periods and the dyskinesias, dystonia, and motor fluctuations that result from long-term drug administration.111–113