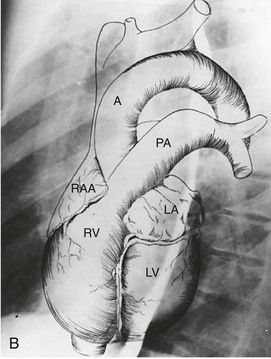
(From Van Houten FX, Adams DF, Abrams HL: Radiology of valvular heart disease. In Sonnenblick E, Lesch M [eds]: Valvular Heart Disease. New York, Grune & Stratton, 1974.)
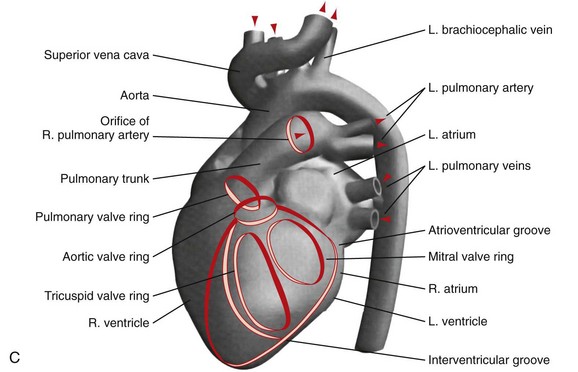
(From Steiner RM: Radiology of the heart and great vessels. In Braunwald E, Zipes PD, Libby P [eds]: Heart Disease: A Textbook of Cardiovascular Medicine, 6th ed. Philadelphia, Saunders, 2001.)
TABLE 5-1 Cardiovascular and Pulmonary Devices and Other Appliances in the ICU Patient
CHAPTER 5 Radiology of the Heart
Plain Film Imaging and Diagnosis
INTRODUCTION
All of these newer modalities yield anatomically detailed images of the heart and great vessels not possible with plain film radiography alone. However, the chest radiograph continues to provide valuable and often unique information concerning the structure and function of the cardiovascular system. The chest radiograph presents an opportunity to recognize subtle and/or overlooked abnormalities such as cardiac chamber enlargement, clinically important calcifications, and evidence of right and left heart dysfunction. Because the pulmonary arteries and veins are visualized in exquisite detail on a well-performed posterior-anterior (PA) and lateral chest radiograph, over and under pulmonary circulation as well as the findings of pulmonary arterial and venous hypertension can be appreciated. Adult onset congenital heart disease, often misdiagnosed or overlooked clinically, can often be identified on a plain film chest radiograph.1,2
The portable chest radiograph, usually performed in the anterior-posterior (AP) supine or erect position is the imaging modality of choice for the evaluation and monitoring of patients with cardiopulmonary disease in the intensive care unit (ICU) including postoperative cardiac patients and in those with implanted cardiovascular devices.3,4
NORMAL AND ABNORMAL CARDIAC BORDERS
Chest Radiograph
In a well-positioned PA or frontal chest radiograph, the cardiac silhouette and other vascular structures are predictably outlined against the lung as a series of bulges and indentations along the right and left mediastinal borders (Fig. 5-1).
Left Mediastinal Border
In the normal patient, the most superior border-forming structure along the left side of the mediastinum is the left subclavian artery (LSCA). Positioned just above the aortic arch and below the left clavicle, it usually forms a concave border with the lung and lies medial to the aortic arch (see Fig. 5-1). When blood flow through the LSCA is increased, as in a postductal coarctation of the aorta, or when the vessel is tortuous because of atherosclerosis, it will lie at or lateral to the aortic knob or arch—an important clue to the diagnosis of disease. A vertical, straight, or convex, double shadow parallel to the LSCA border is found when a left superior vena cava is present (Fig. 5-2). A cervical aortic arch or the elongated aortic arch of a pseudocoarctation of the aorta may also obscure the normal LSCA border.
Aortic Arch
The aortic arch or “knob” forms a convex segment along the left mediastinal border below the LSCA border and above the main pulmonary artery border. The aortic convexity is the distal posterior portion of the arch measuring 2.0 ± 1 cm in the young adult and 2.5 to 3.5 cm in the normal middle-aged individual. A clue to the location of the medial border of the aortic arch is the right-sided displacement of the tracheal airway at the same level as the arch. Although the aortic arch border usually has smooth margins, a rounded 2 to 3 mm extension or “nipple” may be present. This structure, seen in up to 10% of normal individuals, is the left superior intercostal vein (LSIV) (Fig. 5-3). An enlarged LSIV will suggest obstruction to blood flow in the deep mediastinal venous system such as superior vena caval obstruction. A dilated LSIV is similar in significance to a dilated azygos vein.
The left side of the descending thoracic aorta forms a clearly visualized interface with the left lung behind the left heart border on a well-penetrated frontal chest film (see Fig. 5-4). Pleural effusion and pulmonary masses, left lower lobe pneumonia, and other soft tissue opacities may obliterate the descending aortic border.
When the aortic arch border on the left side of the mediastinum is not visible and when the trachea is displaced to the left, the presence of an aortic arch on the right side of the mediastinum will suggest the diagnosis of right aortic arch (RAA) (Fig. 5-4). It is associated in most cases with an aberrant left subclavian artery; however, RAA may occur without an associated aberrant subclavian artery in mirror image RAA with or without associated cyanotic heart disease.
Main Pulmonary Artery Border (see Fig. 5-1)
The normally convex main left pulmonary artery (LPA) is situated immediately below the aorticopulmonary window and extends posteriorly as it arches over the left bronchus. It will enlarge because of increased pulmonary blood flow due to a left-to-right shunt, increased pulmonary pressure in patients with obliterative lung disease or Eisenmenger physiology, pulmonary valve stenosis, or weakness of the pulmonary arterial wall in an arteritis (Fig. 5-5). The main pulmonary artery (MPA) and LPA will bulge to the left and the pulmonary artery branches will converge at the lateral border of the enlarged vessel. Alternatively, if the pulmonary artery border is flat or concave, the MPA may be absent or stenotic when truncus arteriosus, tetralogy of Fallot, pulmonary atresia, or transposition of the great vessels is present.
Left Atrial Border (LAA)
The LAA lies below the left main-stem bronchus in the frontal projection and is border forming with the lung. The LAA forms a smooth and slightly concave portion of the left heart border (see Fig. 5-1). Atrial enlargement should be suspected when the LAA is convex and prominent (Fig. 5-6) but the LA may enlarge significantly, even when the LAA remains small, so that enlargement of the LA may be suspected by other signs of enlargement including a double right atrial-left atrial border on the right side of the mediastinum, elevation and widening of the carinal angle, and a prominent LA bulge on the lateral projection. Nonvascular pathology may simulate enlargement of the left atrial appendage. For example, a pericardial cyst, lymphoma, thymoma, or other mediastinal or pleural neoplasms may appear as a convexity of the left atrial border (Fig. 5-7). Bulging of the LAA border may also be caused by partial absence of the pericardium.
Left Ventricular Border (LV)
The left ventricular border continues downward seamlessly from the left atrial border. The LV is usually mildly convex in relation to the nearby lung. When the LV is hypertrophic as a result of aortic stenosis or hypertrophic obstructive cardiomyopathy, it may be round and slightly enlarged. When the left ventricle is dilated, as may occur with aortic regurgitation or other causes of decompensation, the apex is displaced downward and laterally (Fig. 5-8). An enlarged right ventricle (RV) may displace the LV border downward, thereby simulating LV dilation or aneurysm.
When the LV dilates because of volume overload as in patients with mitral regurgitation, the dimensions of the LV chamber increase markedly, assuming a globular or water flask appearance similar to a large pericardial effusion. The LV border may extend to the left and can reach the lateral ribs in massive LV dilation. As the LV enlarges, the LA border is obscured. In such circumstances, the left anterior oblique (LAO) projection is helpful to separate the two chambers so that their relative sizes can be discerned (Fig. 5-9). Separating the left heart chambers assumes importance when the differential diagnosis lies between ischemic cardiomyopathy (in which case the left ventricle is larger than the left atrium) and mitral regurgitation (in which case the left atrium may be larger than the left ventricle).
Right Mediastinal Border
The right atrial (RA) border is convex in its relationship to the medial segment of the right middle lobe. In the frontal projection, the superior vena cava (SVC) border above the RA is usually straight, and in a good inspiratory film, it can be clearly separated from the RA. The outline of the LA may be visible deep to the RA border as an additional convex shadow and the confluence of the right pulmonary veins directed toward the mid-point of the LA border may also be appreciated. In a well-penetrated film, the LA is also clearly visualized deep to the RA border because of intrusion of lung between the anterior portion of the LA and the more posterior border of the RA. If the LA is markedly enlarged, its border may actually be lateral to the RA. The borders of the right and left atria can be differentiated because the inferior border of the RA blends with the inferior vena cava, whereas the LA crosses the mid-line toward the left side of the heart (Fig. 5-10) The upper right atrial convexity blends superiorly with the SVC, which forms a straight interface with the adjacent lung as it continues into the neck. The RA is considered enlarged when it bulges more than 5.5 cm to the right of the mid-line.
Azygos Vein
The azygos vein forms an elliptical or teardrop structure at the right tracheobronchial angle (see Fig. 5-1A). It ascends in the right paravertebral sulcus and arches forward over the right mainstem bronchus to enter the back of the SVC. The azygos vein and its left-sided equivalent, the hemiazygos vein, receive intercostal veins and act as an important collateral pathway when the deep mediastinal veins are obstructed. Normally measuring 0.7 ± 0.3 cm across in the erect and 1.0 ± 0.3 cm in the supine position, the azygos vein is a good indicator of changing cardiovascular dynamics. It is enlarged in SVC and IVC obstruction, in the absence of the intrahepatic portion of the inferior vena cava, in portal vein obstruction, and in both left- and right-sided cardiac failure. A change in diameter of the azygos vein from film to film will parallel changes in pulmonary venous pressure, which makes it a useful guide to the development of congestive heart failure on plain film radiographs. An azygos fissure can be found in 3% of the population. When an azygos fissure is present, the azygos vein is displaced laterally and superiorly and will dilate under the same conditions as a normally positioned azygos vein.