Chapter 85C Radiofrequency ablation for liver tumors
Overview
Hepatic resection and transplantation are the only curative options for patients with liver metastases or primary liver tumors (see Chapter 80, Chapter 81A, Chapter 81B, Chapter 81C, Chapter 97A, Chapter 97B, Chapter 97C, Chapter 97D, Chapter 97E ). Unfortunately, resection is possible in only about 20% of patients; most hepatic malignancies are surgically inaccessible or are associated with a large tumor burden or inadequate hepatic reserve. Liver transplantation for hepatocellular carcinoma (HCC) is limited by a shortage of donor organs (see Chapter 97D). Patients with unresectable disease may be candidates for systemic therapy (see Chapter 88), local ablative techniques (percutaneous ethanol injection, microwave tumor coagulation, interstitial laser photocoagulation, cryosurgical ablation, or radiofrequency ablation [RFA]; see Chapter 85A, Chapter 85B, Chapter 85D ), or hepatic-directed therapy (hepatic artery ligation, chemoembolization, hepatic artery perfusion; see Chapters 83, 86, and 89).
Over the past decade, multiple clinical trials have evaluated RFA for the treatment of primary liver tumors and liver metastases. Animal experiments in the early 1990s determined the size and shape of ablations that could be achieved in the liver (Rossi et al, 1990). Rossi and colleagues (1996) then demonstrated the effectiveness of RFA for the treatment of HCC in humans. The Food and Drug Administration (FDA) approved RFA for generic tissue ablation in 1996 and for ablation of unresectable hepatic metastases in 2000. Since then, randomized trials have been performed in early HCC that demonstrate equivalent survival to resection (Lu et al, 2006), but prospective data in colorectal liver metastases are limited.
RFA destroys tumor by generating heat within a lesion. During RFA, a high-frequency alternating current changes the direction of ions around an alternating electrode charge. This creates frictional energy and heat conduction. As tissue temperature increases above 45° C, loss of cellular structure and protein denaturing result in tumor cell death. RFA can be performed in the operating room via celiotomy or laparoscopy, or it may be done in the radiology suite by a percutaneous approach. It can be used with other modalities of liver-directed therapy, such as resection and hepatic artery perfusion, and it can be used in conjunction with systemic therapy for other sites of metastatic disease. RFA continues to evolve with the introduction of new technology that increases the field of ablation and simplifies the technique. Long-term survival data have been reported in both HCC and colorectal hepatic metastases (Table 85C.1). A recently published position statement by the Society of Interventional Radiology (Gervais et al, 2009) describes four categories of patients that are preferred candidates for RFA: those with 1) inadequate liver function, 2) comorbid conditions, 3) anatomic distribution, and 4) for local tumor control as a bridge to transplantation.
Radiofrequency Ablation Technology
RFA destroys tissue by generating enough heat to denature cell protein and cause cell death (Fig. 85C.1), which can occur instantaneously at 60° C, a temperature achieved by all RFA devices. During RFA an electrical generator produces a current that alternates directions rapidly. Charged molecules in the tissue follow the direction of the changing current: this results in friction, which produces heat. The current around the ablation electrode creates a relatively uniform zone of conductive heat that radiates out from the electrode. If tissue impedance is low, an expanding spherical zone of ablated tissue is created. As the temperature rises, tissue charring and desiccation occur. Also, nitrogen gas forms that can be seen on ultrasound (US) as air bubbles in the tissue. Both of these events raise tissue impedance and inhibit heat conduction, thereby inhibiting further tissue ablation. The spherical size of the ablated tissue is proportional to the square of the RF current (RF power density). It is also dependent on the size of the electrode and the duration of the applied energy. The RF power density decreases in proportion to the square of the distance from the electrode, resulting in a rapid decrease in tissue temperature with increasing distance from the electrode (Strasberg & Linehan, 2003). Changing technology strives to improve ablation zones by limiting impedance and increasing the RF power density.
Three different RF electrodes and generators are commercially available. The Radiofrequency Interstitial Tissue Ablation (RITA; AngioDynamics, Latham, NY) and RadioTherapeutics (Boston Scientific, Natick, MA) systems use multiarray electrodes (Fig. 85C.2). The RITA system has a single electrode through which multiple tines are deployed. The ablation is performed at sequential steps of electrode deployment. Thermoprobes measure temperature in the ablation zone, and an automated adjustment is then made to maintain a steady temperature during the ablation. The electrode/generator system measures temperature and time as end points of ablation. This system was recently modified by the addition of saline perfusion to reduce impedance and increase the diameter of the ablation field to 7 cm. The RadioTherapeutics system automatically adjusts current according to tissue impedance; temperature is not measured. The multiarray umbrella-shaped electrode can ablate fields of about 4 cm. The Cool Tip Cluster Electrode, a single or three-pronged electrode, is manufactured by Covidien (Mansfield, MA); each electrode is internally perfused with cold saline, the current is sent in pulses, and the system measures impedance and temperature. Ablation zones can reach 5 cm in diameter with the three-pronged device.
Technical Aspects of Radiofrequency Ablation
If extrahepatic and/or extensive intrahepatic disease has been detected, ablation is unlikely to be of any benefit. US during surgical or percutaneous RFA or CT during percutaneous RFA is used to guide the probe into the lesion, and the ablation is monitored by real-time US imaging of an expanding hyperechogenic zone (Fig. 85C.3). Depending on the maximal ablation size achievable by the probe, multiple overlapping ablations may be needed to destroy a tumor and produce a surrounding rim of necrosis (Fig. 85C.4). This can be technically challenging. US cannot reliably distinguish between ablated and normal tissue, so ablation should begin at the most posterior portion of the tumor. The probe then can be withdrawn in approximately 2-cm increments to create sequential overlapping zones of ablated tissue. If target temperatures are not reached, as may be the case for lesions near major vascular structures because of a heat-sink effect, the tines are withdrawn slightly or rotated approximately 45 degrees to increase the temperature in the region of ablation. Each ablation should create a 1-cm margin of treated normal parenchyma to ensure complete tumor destruction and reduce the risk of local recurrence. After ablation, as the RFA needle is withdrawn, the probe tract is cauterized to prevent hemorrhage and tumor seeding of the needle tract.
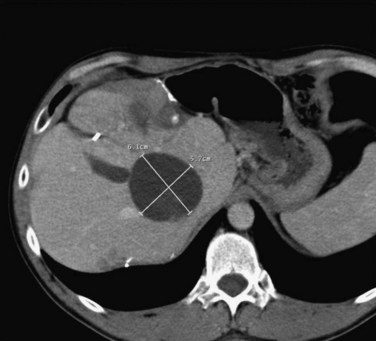
FIGURE 85C.4 Computed tomographic scan demonstrating large-volume ablation achieved with multiple overlapping ablation technique.
Percutaneous Radiofrequency Ablation
The percutaneous approach is somewhat limited, as some liver lesions can only be accessed by a surgical approach. Tumors near the periphery of the liver may be problematic because of the risk of thermal injury to other visceral structures such as the small bowel, stomach, or transverse colon; with an open technique, these structures can be retracted away from the liver. Other lesions can be approached percutaneously but raise technical challenges. For example, ablation of lesions at the dome of the liver might result in thermal injury to the diaphragm. Introduction of an air pocket can be used to separate the diaphragm and liver to avoid diaphragmatic thermal injury (Raman et al, 2004); this requires skill and may result in complications such as diaphragmatic injury or pneumothorax. Injection of saline or use of a balloon catheter has also been reported to separate structures from the liver to avoid thermal injury; however, clinically relevant diaphragmatic injuries are rare in our experience (Fig. 85C.5). Because a percutaneous approach is less invasive than a surgical approach, it may also be preferable in patients with comorbid conditions or poor medical status.
Open or Laparoscopic Radiofrequency Ablation
RFA via celiotomy or laparoscopy is performed in the operating room by a surgeon, while the patient is under general anesthesia. Unlike the percutaneous approach, a surgical approach allows visual inspection of the intraabdominal cavity for extrahepatic disease. Intraoperative US is used to evaluate the liver for intrahepatic lesions not seen on preoperative imaging. In a study of 308 patients undergoing laparoscopic RFA with intraoperative hepatic US, preoperative imaging failed to identify extrahepatic disease in 12% of patients and additional hepatic lesions in 33% of patients (Bilchik et al, 2000). Similarly, Siperstein and colleagues (2000) found that 30% of patients who underwent laparoscopic US of the liver prior to RFA had additional metastatic nodules not apparent on the preoperative CT scan. Larger liver lesions (>5 cm) are more easily treated with an operative approach than percutaneously. These lesions may require multiple overlapping ablations. Intraoperative occlusion of the portal triad can increase the ablation zone by decreasing the heat-sink effect created by nearby vessels. Furthermore, a surgical approach is sometimes necessary when other treatments are used in combination with RFA. For example, a surgical approach is ideal for patients who have bilobar liver metastases, who may benefit from concomitant liver resection. Larger liver lesions may be resected, and smaller lesions in the opposite lobe may be treated by RFA.
Monitoring and Follow-up
Contrast-enhanced CT scans obtained within a month and then at 3-month intervals after RFA should be compared with preprocedure CT scans. Liver necrosis from RFA can be seen as a nonenhancing hypodense region. When complete necrosis of the tumor is achieved, the resulting necrotic zone exceeds the original tumor size. Postprocedure CT scans are important to determine the success of RFA and to monitor the liver for evidence of recurrence. PET scans may also be particularly sensitive in the assessment of the adequacy of ablation and presence of marginal recurrence (Fig. 85C.6) or to distinguish viable tumor from areas of previous surgical or ablative therapy (Fig. 85C.7).