Chapter 47 Principles of Endovascular Therapy
Ischemic Stroke
Acute Stroke Treatment
Stroke is the third leading cause of death and the most common reason for disability worldwide (Rosamond et al., 2008). The majority of ischemic strokes result from vessel occlusion secondary to thromboembolic or atheromatous processes (Sussman and Fitch, 1958). To date, timely revascularization and reperfusion of ischemic brain tissue is the most effective therapeutic strategy. Intravenous (IV) thrombolysis has been restricted to a very narrow 3-hour time window based on the NINDS trials (The National Institute of Neurological Disorders and Stroke rt-PA Stroke Study Group, 1995). Using modified clinical selection criteria, the third European Cooperative Acute Stroke Study recently demonstrated a benefit of IV thrombolysis up to 4.5 hours after symptom onset (Hacke et al., 2008). Nonetheless, the main restrictions on IV recombinant tissue plasminogen activator (rtPA) remain the narrow time window for administration and the nondiscerning systemic mode of delivery. Coupled with an insufficient public awareness, these factors have limited the use of IV thrombolysis to less than 5% of eligible candidates (Barber et al., 2001). Endovascular methods of acute stroke treatment have been developed to help address these shortcomings.
Although inclusion criteria vary for each of the endovascular acute stroke trials, careful patient selection and prudent utilization of recanalization therapies are critical. Treatment algorithms are predicated on clinical and radiographic criteria. Precise assessment of the National Institutes of Health Stroke Scale (NIHSS) (Box 47.1) and time of symptom onset determines risk/benefit stratification. Axial imaging (computed tomography [CT], magnetic resonance imaging [MRI]) is used to quantify infarct size and screen for hemorrhage. CT or MR angiography (CTA, MRA) can determine vessel patency, whereas perfusion-weighted MRI helps characterize salvageable tissue in the ischemic penumbra.
Box 47.1 National Institutes of Health Stroke Scale
Intraarterial Thrombolysis
Compared to IV approaches, intraarterial (IA) administration of thrombolytics via a microcatheter positioned in the cerebral vasculature affords rapid local delivery of greater therapeutic treatment concentrations to the site of vascular occlusion. The selective nature of the delivery system maximizes lysis while limiting systemic conversion and resultant hemorrhage. Intraarterial thrombolysis represents a targeted strategy that lengthens the total therapeutic window for acute stroke intervention. The technique entails performing a catheter-based cerebral angiogram to confirm the point of occlusion. Under fluoroscopic guidance, a microcatheter is advanced though a larger guide catheter to the base of, into, or distal to the clot. Once positioned, a thrombolytic agent is injected over a 1- to 2-hour time period as intermittent control angiograms are performed (Gandhi et al., 2009). Clinical trials have been performed using streptokinase, urokinase, rtPA, recombinant prourokinase, and reteplase. These agents differ in fibrin selectivity, stability, half-life, and mechanism of action (Gandhi et al., 2009; Schellinger et al., 2001). The Prolyse in Acute Cerebral Thromboembolism II (PROACT II) trial demonstrated the effectiveness of IA prourokinase when given within 6 hours of acute stroke caused by middle cerebral artery occlusion (Furlan et al., 1999). Patients were randomized to IA recombinant prourokinase and IV heparin or heparin alone. The primary clinical outcome, the proportion of patients with minimal or no disability at 90 days (modified Rankin Scale [mRS] score ≤ 2), was achieved in 40% of the treatment group (patients administered heparin and IA prourokinase) and 25% of the controls (patients administered heparin only).
Although IA thrombolysis is an effective treatment, the time delay required for cerebral angiography and microcatheter positioning is an inherent shortcoming. To address this, bridging strategies have employed a combined IV/IA approach. The first such trial was the Emergency Management of Stroke Bridging Trial (Lewandowski et al., 1999). This was a double-blind randomized placebo-controlled phase-I trial of IV rtPA or placebo followed by immediate IA treatment with rtPA. Although recanalization was significantly greater in the IV/IA rtPA group, there was no difference in 3-month functional outcomes, and an increased incidence of death was observed in that treatment group.
The Interventional Management of Stroke (IMS) study investigators compared acute stroke patients treated with a bridging IV rtPA dose (0.6 mg/kg) followed by IA rtPA, with historical controls given only the traditional IV dosing regimen from the NINDS trial. The IMS I study was an open-label single-arm feasibility study using a standard microcatheter to infuse a total dose of up to 22 mg of IA rtPA over 2 hours (IMS Study Investigators, 2004). The objective of the second IMS study (IMS II) was to continue investigating the feasibility of a combined IV/IA approach, permitting the use of the EKOS MicroLysUS (EKOS Corp., Bothell, Washington) microinfusion catheter, which incorporates sonographic technology to increase permeability and penetration of the intraluminal clot (IMS II Trial Investigators, 2007). The IMS study cohorts demonstrated better functional outcomes and a decreased mortality rate compared to historic controls. The rate of symptomatic intracranial hemorrhage was similar to that of the IV rtPA group in the NINDS trial (IMS Study Investigators, 2004; IMS II Trial Investigators, 2007; National Institute of Neurological Disorders and Stroke rt-PA Stroke Study Group, 1995). These data suggest that bridging protocols combining IV therapy and subsequent endovascular procedures may improve recanalization rates and functional outcome following acute stroke (Mazighi et al., 2009). Definitive evidence to support this combination may not be available, however, until the prospective randomized IMS III trial is completed.
Mechanical Recanalization
Although outcomes following acute stroke have improved with the administration of IV/IA thrombolytics, there are many patients who are not candidates for these treatment schemes (Furlan et al., 1999; Williams et al., 2009). Narrow time windows and the risk of hemorrhage associated with thrombolytic agents have prompted the design and application of mechanical thrombectomy devices. Endovascular clot retrieval provides potential for rapid restoration of flow, with a decreased incidence of clot fragmentation and distal embolism (Nogueira et al., 2009). Catheter-based retrieval/aspiration systems are used in acute stroke patients who are either ineligible for or have failed IV rtPA administration; they may be used in conjunction with thrombolytic infusion. Clot-retrieval devices have effectively lengthened the treatment window for acute stroke.
The first device to have received U.S. Food and Drug Administration (FDA) approval for IA stroke treatment is the MERCI clot retriever (Concentric Medical Inc., Mountain View, California). This retrieval system includes the MERCI retrieval device, a compatible microcatheter, and a balloon guide catheter. The retriever is a flexible tapered nitinol wire with radiopaque helical loops at the distal tip (Fig. 47.1). Newer-generation retrievers (L series and V series) have cylindrical rather than tapered loops (X series) and a bound suture material that enhances clot capture. An 8F or 9F balloon guide catheter is advanced into a proximal vessel and inflated to cease anterograde blood flow and thus prevent distal emboli during clot extraction. The microcatheter is advanced coaxially over a microguidewire into the target vessel and through the clot. Once situated in the distal vessel, the microguidewire is exchanged for the clot retriever. The device is unsheathed to position several loops distal to the clot, and the retriever/microcatheter unit is then withdrawn into the balloon guide catheter under constant manual suction (Fig. 47.2).
Results from the nonrandomized Mechanical Embolus Removal in Cerebral Ischemia (MERCI) trial demonstrated safety and efficacy of the X-series clot retrieval system in restoring the patency of occluded intracranial vessels within the first 8 hours of acute stroke (Smith et al., 2005). Some 48% of occluded vessels were recanalized, a rate significantly higher than that of the control arm in the PROACT II trial (18%) (Furlan et al., 1999; Nogueira et al., 2009b). After adjuvant therapy (IA rtPA, angioplasty, snare), the rate of recanalization was 60.3%. The overall rates of good outcome (mRS score ≤2) and mortality were 27.7% and 43.5%, respectively, with a clinically significant procedural complication rate of 7.1%. Revascularization was found to be an independent predictor of decreased mortality and favorable neurological outcome at 90 days.
More recently, the Multi-MERCI Stroke Trial was conducted. This was an international multicenter, prospective, single-arm trial similar to the previous MERCI trial (Smith et al., 2008). However, this study included patients receiving IV rtPA with persistent arterial occlusion and allowed utilization of a newer-generation retrieval device (L5). Treatment with the retriever alone resulted in successful recanalization of 55% of treatable vessels. Recanalization rate was 68% after adjuvant therapy. The overall rates of good outcome (36%) and mortality (34%) were substantially improved when compared to those of the original MERCI trial. Once again, good outcomes were more frequent and mortality was lower with successful recanalization. The authors concluded that mechanical thrombectomy after IV rtPA seems as safe as mechanical thrombectomy alone, and that thrombectomy is efficacious in opening intracranial vessels during acute stroke in patients who are either ineligible for or have failed to recanalize with IV rtPA.
The Penumbra System (Penumbra Inc., Alameda, California) is an aspiration device through which a thromboembolic clot can be retrieved following acute stroke (Fig. 47.3). The device removes thrombus via aspiration, mechanical disruption, and extraction. Multiple aspiration catheters of varying luminal diameters are available for use in the cervical and intracranial vasculature, depending on vessel caliber. The aspiration device is advanced coaxially to the level of the thrombus through a guide catheter. Once positioned just proximal to the target lesion, an aspiration pump is connected to the reperfusion catheter. The separator is then advanced through the catheter into the distal clot and repeatedly retracted and advanced, aiding in the debulking process. A multicenter prospective, single-arm, phase I trial of the Penumbra reperfusion catheter was designed to assess safety and efficacy (Bose et al., 2008). The primary endpoint was revascularization, defined by a Thrombolysis in Myocardial Infarction (TIMI) score of 2 or 3. Recanalization was achieved in all vessels in which the device was deployed. A secondary endpoint of mRS score of 2 or less or improvement of NIHSS score by 4 or better was achieved in 45% of patients. The Penumbra device has recently received FDA approval (Fig. 47.4).
No other mechanical embolectomy device is currently approved by the FDA for use in acute stroke. The Phenox Clot Retriever (Phenox GmbH, Bochum, Germany), Neuronet Endovascular Snare (Guidant Corp., Temecula, California), Catch Mechanical Thrombus Retriever (Balt, Montmorency, France), Alligator retriever (Chestnut Medical Technologies Inc., Menlo Park, California), Amplatz GooseNeck Snare (ev3 Inc., Irvine, California), and TriSpan device (Boston Scientific, Natick, Massachusetts) have all been used successfully in a limited number of acute stroke patients, without large-scale demonstration of safety or efficacy (Gandhi et al., 2009; Katz et al., 2006; Nogueira et al., 2009a).
Angioplasty and Stenting
Despite increased experience with IA thrombolytic administration and technical advancements in the development of embolic retrieval or aspiration devices, many intracranial thrombi remain recalcitrant to thrombolysis or embolectomy. Mature fibrinous clots are often difficult to treat pharmacologically, and thrombi adherent to the vascular intima or associated with underlying atheromatous disease pose inherent structural risks to mechanical embolectomy procedures. Percutaneous transluminal angioplasty (PTA) and stenting procedures have been successful in acute coronary revascularization. Recanalization in the absence of clot dissolution or extraction may be sufficient for successful acute stroke treatment (Levy et al., 2009). Several small studies have reported improvement in clinical outcome following angioplasty and thrombolytic administration in the setting of acute stroke (Nogueira et al., 2008; Ringer et al., 2001; Yoneyama et al., 2002). Recently a single-arm prospective trial of primary intracranial stenting for acute stroke demonstrated promising results. The authors reported 100% TIMI 2-3 recanalization in a cohort of 20 patients treated with intracranial stenting. Self-expanding stents (Wingspan [Boston Scientific, Natick, Massachusetts]; Enterprise [Cordis Neurovascular Inc., Miami Lakes, Florida]) designed exclusively for cerebral indications facilitated access and navigation through tortuous craniocervical anatomy. Although adjuvant IA antiplatelet therapy was used in 50% of patients, the authors reported only a 5% rate of symptomatic intracranial hemorrhage. At 1 month, 60% of patients had mRS scores of 3 or below, and 45% had mRS scores of 1 or below. These results are encouraging. Larger studies are needed, however, to adequately assess the safety and efficacy of this treatment modality.
Alternative Reperfusion Strategies
Alternatives to recanalization strategies focus on collateral perfusion as well as flow reversal following acute stroke. One such mechanical approach to flow augmentation is the NeuroFlo catheter (CoAxia Inc., Maple Grove, Minnesota), a dual-balloon catheter designed for partial occlusion of the descending aorta, above and below the renal arteries (Fig. 47.5). Temporary partial aortic occlusion is thought to augment cerebral blood flow through mechanical flow diversion. Multicenter clinical trials are currently being conducted to assess the safety and efficacy of this treatment modality (Liebeskind, 2010; Nogueira et al., 2009a; Uflacker et al., 2008). If efficacious, such alternate reperfusion strategies may serve to supplement existing recanalization treatments or represent a novel paradigm for patients ineligible for direct revascularization.
Carotid Artery Disease
The two large landmark randomized controlled trials that enrolled symptomatic carotid stenosis patients were the North American Symptomatic Carotid Endarterectomy Trial (NASCET) and the European Carotid Surgery Trial (ECST) (European Carotid Surgery Trialists’ Collaborative Group, 1996; Barnett et al., 1998). The NASCET investigators revealed that carotid endarterectomy reduced the risk of ipsilateral stroke (9%) at 2 years, compared to best medical therapy (26%) in patients harboring carotid stenoses of 70% to 99%. A more modest yet still significant reduction in the 5-year rate of ipsilateral stroke was evident in patients with 50% to 70% stenoses. The overall perioperative stroke and death rate for patients treated with CEA was 5.8%. Using a technically varied measurement criterion for quantifying luminal stenosis, the ECST arrived at a similar result. Data demonstrated that surgically treated patients with stenoses greater than 80% had a lower estimated risk of death or major stroke when compared to those managed medically.
The Asymptomatic Carotid Atherosclerosis Study (ACAS) and the Asymptomatic Carotid Surgery Trial (ACST) addressed indications for CEA in asymptomatic patients (Executive Committee for the Asymptomatic Carotid Atherosclerosis Study, 1995; Halliday et al., 2004). Both studies demonstrated that compared to those treated with best medical therapy, the 5-year incidence of ipsilateral stroke or death was reduced in the carotid endarterectomy group. The degree of benefit for asymptomatic patients was substantially less than previously documented in symptomatic individuals. The 5-year stroke and death rate was 11% for patients in the medical therapy cohort, while the stroke risk was 5.1% for patients treated with CEA. The benefit was evident predominantly in men.
Based on such results, the American Heart Association guidelines recommend CEA for symptomatic patients with 50% to 99% stenosis if the perioperative risk of stroke or death is less than 6%, and for asymptomatic patients with stenoses of 60% to 99% if the perioperative risk of stroke or death is less than 3% (Biller et al., 1998; Sacco et al., 2006).
Carotid Artery Angioplasty and Stenting
Angioplasty and Stenting Procedure
Next, embolic protection is secured in all attainable cases. Carotid plaques may be extremely friable, and even minor manipulation can result in distal embolization or vessel occlusion. Cerebral protection devices of varying construction are used to prevent embolic complications during CAS procedures. Most commonly, over-the-wire distal filters measured to the diameter of the native cervical internal carotid artery (ICA) are navigated past the stenotic lesion to capture embolic material (Fig. 47.6). Such devices allow continuous cerebral perfusion through pores in the filter baskets. Following angioplasty and stenting, the device is collapsed and withdrawn and embolic material retrieved. Alternatively, balloon occlusion devices may be used to completely prevent anterograde flow within the ICA. Any displaced material can be aspirated prior to balloon deflation. However, temporary carotid occlusion may not be well tolerated in all patients, limiting the applicability and utility of this strategy. Guide catheters with distal occlusion balloons may be inflated in the common carotid artery to achieve proximal control and distal flow reversal. Concomitant balloon inflation in the external carotid artery results in more thorough protection. Temporary proximal occlusion is generally performed when distal deployment of an embolic protective device (EPD) is not feasible owing to vessel size, tortuosity, or other anatomical restrictions.
Once embolic protection is achieved, balloon predilatation of tight stenoses can help ensure safe advancement of a self-expanding stent past the target lesion. Balloon inflation is done slowly to lessen the incidence of thromboembolism. Deflation must also be undertaken in a controlled, deliberate fashion. An experienced anesthesiologist and open communication are critical during this portion of the procedure. The endovascular surgeon must inform the anesthesia team of possible bradycardia associated with balloon inflation. This way, changes in the heart rate can be managed in a timely and proper fashion during angioplasty. Stent diameter is calibrated to 100% of the common carotid artery diameter, and length is measured to traverse the entire lesion with a margin of several millimeters both proximally and distally. Tapered stents are available to accommodate the larger diameter of the common carotid artery and the smaller lumen of the ICA. Depending on residual stenosis and distal perfusion, post-stent balloon dilatation may be undertaken. It is critical that balloon angioplasty remain within the confines of the stented arterial segment to prevent dissection of unprotected vascular intima (Fig. 47.7).
Clinical Trials
When carotid angioplasty procedures were initially introduced more than 25 years ago, they were performed without stenting or distal embolic protection. Despite these limitations, early data suggested the promise of percutaneous intervention (Yadav et al., 1997). The first multicenter randomized trial that compared carotid angioplasty and CEA was the Carotid and Vertebral Artery Transluminal Angioplasty Study (CAVATAS Investigators, 2001). This trial enrolled symptomatic patients with clinically significant (>80%) ICA stenosis. Only 26% of patients (those enrolled later in the study) underwent stenting in addition to transluminal angioplasty. Nonetheless, the 30-day risk of death or stroke was similar between the percutaneous and surgical groups.
The first multicenter randomized CAS study in the United States, the Carotid Wallstent Trial, was conducted using the tracheobronchial Wallstent device (Boston Scientific, Natick, Massachusetts) in symptomatic patients deemed standard risk for carotid endarterectomy. The trial was terminated prematurely, and although the outcomes for stenting and surgery were not statistically different, a strong trend favored surgery (Alberts, 2001). Many believe that relatively limited operator experience and early-generation nondedicated devices profoundly affected the outcome of the stenting cohort in this investigation. The efficacy of a nascent technique was being compared to that of a refined surgical procedure.
Through the 1990s, dedicated improved stent implants and EPDs were developed. Randomized CAS trials and registries targeted patients at high risk for endarterectomy, a cohort of individuals for which previous data were either lacking or revealed suboptimal surgical outcomes, The multicenter randomized Stenting and Angioplasty with Protection in Patients at High Risk for Endarterectomy (SAPPHIRE) trial enrolled high-risk symptomatic and asymptomatic patients (Yadav, 2004). The primary endpoint (death, stroke, and myocardial infarction (MI) at 30 days, plus ipsilateral stroke or neurological death at 1 year) occurred at a rate of 12.2% for stenting and 20.1% for surgery patients (P = 0.05). In addition to achieving noninferiority of the primary outcome, the study demonstrated significant decrease in target-vessel revascularization (repeat procedures) and cranial nerve palsies in the CAS cohort (P <0.05). On the basis of these data, the FDA recommended approval of the Cordis PRECISE stent and ANGIOGUARD distal filter device (Cordis Corp., Miami Lakes, Florida) for symptomatic patients at high risk for CEA. Three-year follow-up data demonstrated no significant difference in long-term outcomes between CAS and CEA (Gurm et al., 2008). Following the SAPPHIRE trial, a series of multicenter registries was undertaken to gain approval (ongoing or completed) for similar device configurations marketed by various manufacturers. Based on the results from these trials and registries, the Centers for Medicare and Medicaid Services (CMS) approved coverage for CAS with distal embolic protection in high-risk symptomatic patients with stenoses of 70% or greater (Box 47.2). Additionally, coverage was provided for high-risk symptomatic patients with between 50% and 70% stenoses and high-risk asymptomatic patients with 80% or greater stenoses in accordance with category B investigational device exemption (IDE) clinical trials regulation and enrollment in CAS postapproval studies.
Box 47.2 Patients at High Risk for Carotid Endarterectomy
Significant comorbid conditions include but are not limited to:
1. Congestive heart failure class III/IV
2. Left ventricular ejection fraction <30%
4. Contralateral carotid occlusion
5. Recent myocardial infarction
6. Previous CEA with recurrent stenosis
7. Prior radiation treatment to the neck; and
8. Other conditions that were used to determine patients at high risk for CEA in the prior carotid angioplasty followed by stenting (CAS) trials
Supplementing the results generated by the SAPPHIRE trial and subsequent registry studies was the Carotid Revascularization Using Endarterectomy or Stenting Systems (CARESS) trial (CARESS Steering Committee, 2003). This nonrandomized investigation examined all patient subsets (not limited to high risk) and allowed practitioners to determine treatment allocation. In a more realistic clinical paradigm, the study sought to address whether CAS with distal embolic protection was comparable to CEA in patients with symptomatic and asymptomatic carotid stenosis. One-year outcome data demonstrated a trend toward fewer strokes and death in the CAS group, but the difference was not statistically significant (13.6% CEA versus 10% CAS).
Results from two randomized European trials were published simultaneously in 2006. The Stent Protected Angioplasty versus Carotid Endarterectomy (SPACE) trial was a German study that enrolled symptomatic patients with stenoses greater than 50% (by NASCET criteria) and randomly allocated them to CAS or CEA (Ringleb et al., 2006; Stingele et al., 2008). The primary endpoint was ipsilateral stroke or death at 30 days. The steering committee terminated the trial early because of insufficient funding and the large projected number of patients required to demonstrate noninferiority. Nevertheless, primary outcome data appeared to demonstrate near equivalence among the two groups, despite a lack of EPD utilization in the majority of CAS patients.
The French Endarterectomy versus Stenting in Patients with Symptomatic Severe Carotid Stenosis (EVA-3S) trial had inclusion criteria similar to SPACE (symptomatic, >60% stenosis) (Mas et al., 2006). Primary outcome included any stroke or death at 30 days. The investigation was terminated prematurely owing to objective concerns for safety and futility. The primary endpoint was achieved in 9.6% of CAS patients and 3.9% of CEA patients (P = 0.01). Detractors claim that the infrequent use of EPDs and deficiencies in the experience of stent operators (as few as five lifetime procedures) may have contributed to poor outcome in the CAS cohort. Nonetheless, the disparate SPACE and EVA-3S results further complicated questions regarding CAS indications in standard-risk symptomatic patients. Furthermore, a secondary analysis of pooled data generated from the SPACE and EVA studies failed to demonstrate that outcome event frequency (stroke or death) was directly related to the use of EPDs, suggesting that not all embolic complications could be avoided with device utilization (Tietke and Jansen, 2009).
The large randomized prospective Carotid Revascularization Endarterectomy versus Stenting Trial (CREST) enrolled a total of 1326 symptomatic patients with greater than 50% stenoses and 1176 asymptomatic patients with greater than 60% stenoses deemed good candidates for either CEA or CAS (Hobson et al., 2004). Primary endpoints included death, stroke, and MI at 30 days or ipsilateral stroke at 1 year. Initial data suggested noninferiority of carotid artery angioplasty and stenting in this broad cohort. Findings indicated that the primary endpoint was not significantly different between the two arms (7.2% versus 6.8%). Additionally, the incidence of death, MI, or stroke at 30 days was similar between the two arms (5.2% versus 4.5%). Periprocedural strokes were significantly higher in the CAS arm (4.1% versus 2.3%), but the incidence of debilitating and major strokes was similar between the two arms (0.9% versus 0.7%). The incidence of MI was significantly lower in the CAS arm, compared with the CEA arm (1.1% versus 2.3%). Cranial nerve palsies were also significantly more common in the CEA arm. Long-term follow-up suggested that the incidence of ipsilateral stroke after the periprocedural period (approximately 4 years of follow-up) was similar between the two arms (2.0% versus 2.4%). Subgroup analyses indicated no difference based on gender, but there seemed to be evidence of effect modification by age such that patients 69 years of age or younger did better with CAS, whereas those aged 70 or older did better with CEA (Brott et al., 2010). These results will likely encourage expansion of indications for CAS procedures.
The International Carotid Stenting Study (ICSS), also known as CAVATAS II, is a European trial in symptomatic carotid stenosis patients. It was designed to revisit the questions raised in the original investigation while using stents in all percutaneous procedures (Ederle et al., 2010). Initial results were published at the same time the CREST data were revealed. ICSS enrolled 1713 patients and demonstrated a higher incidence of ischemic stroke and related events after stenting. The incidence of stroke, death, or procedural MI was significantly elevated in the stenting group (8.5%) compared to that the endarterectomy group (5.2%). Risks of any stroke and all-cause deaths were higher in the stenting group than in the endarterectomy group. There was an increased number of cranial nerve palsies in the CEA group.
Several other trials addressed the indications for CAS in patients who are not high risk for surgery. The ongoing ACT I investigation compared stenting and endarterectomy in standard–surgical risk, asymptomatic patients (Ricotta and Malgor, 2008). The proposed Transatlantic Asymptomatic Carotid Intervention Trial (TACIT) aimed to incorporate an optimal medical management arm comparing the best modern medical treatment regimen to CEA and CAS (Gaines and Randall, 2005; Ricotta and Malgor, 2008). Results generated from investigations such as these will help clarify the future role for CAS procedures. Lessons learned from prior studies will likely translate to rapid development of novel technology and refinement of clinical parameters explored in future CAS trials.
Intracranial Atherosclerotic Disease
Intracranial arterial stenosis is a common etiology of cerebrovascular disease, responsible for at least 9% of all ischemic strokes (Sacco et al., 1995). The prognosis for individuals afflicted with this pathology remains poor owing to a paucity of therapeutic options and a relative inefficiency of medical treatment. The Warfarin-Aspirin Symptomatic Intracranial Disease (WASID) trial and subset analyses demonstrated that patients who had suffered an ischemic cerebrovascular event and harbored more than 70% stenosis of a referable intracranial artery were at an exceptionally high risk for recurrent stroke (Chimowitz et al., 2005). A reported 23% of enrolled patients experienced a subsequent ipsilateral stroke over the course of the next year despite aggressive medical therapy (Chimowitz et al., 2005). This high event rate was experienced equally regardless of whether or not patients had failed antithrombotic therapy at the time of their qualifying event (Turan et al., 2009).
Intracranial Angioplasty
Balloon angioplasty was the first percutaneous transluminal technique evaluated. In a large retrospective study, Marks et al. (2006) reported on 120 patients with intracranial stenoses treated by primary angioplasty. The authors calculated a combined periprocedural stroke and death rate of 5.8% and a total yearly event rate of 3.2%. This and other investigations have reported posttreatment residual vessel stenoses in the range of 40% (Marks et al., 2006; Terada et al., 1996). High incidences of angiographic vessel dissection have been documented, although they have rarely been noted to manifest as clinical events (Kiyosue et al., 2004; Marks et al., 2006; Mori et al., 1998; Wojak et al., 2006). Durability of primary angioplasty has also been questioned, with documented retreatment rates near 20% (Siddiq et al., 2008). Mori et al. (1998) investigated lesion-specific features that predicted successful angioplasty and low restenosis rates. They determined that short segment, concentric narrowing, and subtotal angiographic occlusion correlated with the lowest incidence of restenosis following percutaneous transluminal angioplasty (PTA). By contrast, features that portended poor outcome or higher rates of restenosis were eccentricity, extreme angularity, length greater than 10 mm, or excessive tortuosity of the proximal vessel segment. Additionally, the success rate was significantly lower for lesions treated greater than 3 months from the time of stroke.
Experience in the coronary literature indicated that the shortcomings of primary angioplasty (plaque dislodgement, acute elastic recoil, vessel dissection, recurrent stenosis) could be overcome by subsequent stent deployment (George et al., 1998; Savage et al., 1998; Serruys et al., 1994). These concepts were translated directly to the intracranial vasculature.
Intracranial Angioplasty and Stenting
Several early case reports described angioplasty and stenting of cerebral vessels using coronary artery techniques (Chow et al., 2005; Feldman et al., 1996; Gomez et al., 2000; Yu et al., 2005). Device rigidity and vascular tortuosity rendered navigation difficult, resulting in periprocedural morbidity and mortality rates greater than that of angioplasty alone (Chow et al., 2005; Gomez et al., 2000; Jiang et al., 2004; Kim et al., 2004; Levy et al., 2001; Yu et al., 2005). The multicenter nonrandomized Stenting in Symptomatic Atherosclerotic Lesions of Vertebral and Intracranial Arteries (SSYLVIA) trial reported the safety and efficacy of a balloon-mounted stent (Neurolink, Guidant Corporation, Indianapolis, Indiana) designed specifically for craniocervical application; 43 patients with symptomatic intracranial disease and 18 with extracranial vertebral artery stenoses were evaluated. Successful stent placement was achieved in 95% of patients. The investigators reported a 6.6% periprocedural stroke rate and an additional 7.3% incidence of stroke at 1-year follow-up. However, angiographic restenosis was documented in 35% of patients and was symptomatic in over one-third of these individuals (SSYLVIA Study Investigators, 2004). Based on these data, the FDA granted the stent a humanitarian device exemption (HDE) for the treatment of high-risk patients with significant intracranial and extracranial atherosclerotic disease who had failed medical therapy. More recent studies have investigated modified balloon-mounted stenting systems designed exclusively for the cerebral vasculature. Jiang et al. (2004) documented a 91.7% rate of technical success with the Apollo intracranial stent (MicroPort Medical, Shanghai, China) in patients harboring stenoses greater than 50%. The primary endpoint, ischemic stroke in the target lesion arterial territory or any stroke/death within 30 days, occurred at a rate of 4.3 per 100 patient-years. A restenosis rate of 28% was reported. The Vitesse Intracranial Stent Study for Ischemic Therapy (VISSIT), designed to evaluate the Pharos Vitesse system (Micrus Endovascular, San Jose, California), is currently underway (Kurre et al., 2008b).
The Wingspan stent is the first self-expanding system designed solely for intracranial application (Fig. 47.8). A nitinol (nickel and titanium metal alloy with shape memory and superelasticity) construct with high radial force allows navigation of small and tortuous intracranial vessels. Recently the FDA granted an HDE approval for the Wingspan Stent System with Gateway PTA balloon catheter (Boston Scientific, Natick, Massachusetts) for symptomatic ICAD referable to an intracranial vessel with greater than 50% stenosis and refractory to medical therapy. Endorsement was predicated on a safety study conducted in Europe and Asia (Bose et al., 2007). In that initial investigation, technical success was achieved in 98% of patients, and the 6-month death or ipsilateral stroke rate was 7%, with an all-cause stoke rate of 9.7%. In the more recent NIH Multicenter Wingspan Intracranial Stent Registry Study, 129 patients with symptomatic 70% to 99% intracranial stenoses were enrolled. The technical success rate was 97%. The frequency of any stroke, intracerebral hemorrhage, and death within 30 days or ipsilateral stroke beyond 30 days was 14% at 6 months (Zaidat et al., 2008). The reported frequency of angiographic restenosis was 25%.
In-stent restenosis has decreased following cardiac angioplasty and stenting procedures with the advent of drug-eluting stents (Regar et al., 2002). While this generation of stents may be useful in reducing myointimal hyperplasia, no platforms have been specifically designed and approved for use in the small and tortuous intracranial vessels.
Angioplasty/Stenting Procedure
Intracranial angioplasty and stenting with the Gateway/Wingspan system is most often performed under general endotracheal anesthesia. Dual antiplatelet therapy is initiated in anticipation of the procedure. A diagnostic cerebral angiogram is performed to assess degree of stenosis, length of diseased segment, flow restriction, and the presence of leptomeningeal collaterals. A shuttle sheath or guide catheter is used to maintain proximal position and accommodate the angioplasty/stenting platform. Some operators use a distal support catheter in patients with particularly tortuous anatomy. After a microcatheter/microguidewire system is advanced past the lesion, an exchange-length microwire is used to advance, in series, the balloon angioplasty and stent catheters to the level of stenosis. The lesion is initially dilated with a PTA balloon sized to 80% of the native vessel luminal diameter, using the slow inflation technique advocated by Connors and Wojak (Connors and Wojak, 1999). After initial vessel dilation, the balloon catheter is exchanged for the self-expanding stent system, which is deployed across the stenotic lesion. Post-stenting balloon dilation is discouraged by the manufacturer (Figs. 47.9 and 47.10). At the conclusion of the procedure, the average reported residual stenosis (30%) is less than that observed following PTA alone (40%) but more than noted after balloon-mounted stent deployment (10%) (Fiorella and Woo, 2007; Henkes et al., 2005). Follow-up angiograms have demonstrated restenosis rates exceeding 30%, presumably due to elastic recoil, myointimal hyperplasia, and vascular remodeling (Garas et al., 2001). Coating stents with antiproliferative agents such as sirolimus and paclitaxel has been effective in reducing restenosis rates in the cardiology literature (Ong et al., 2006; Stone et al., 2004). Several recent studies have demonstrated encouraging results for the treatment of ICAD patients with drug-eluting stents (Gupta et al., 2006; Qureshi et al., 2006). Difficult vessel navigation with more rigid stenting systems and prolonged postoperative treatment with dual antiplatelet therapies are among the potential limitations to the successful translation of drug-eluting technology to pathologies of the intracranial vasculature.
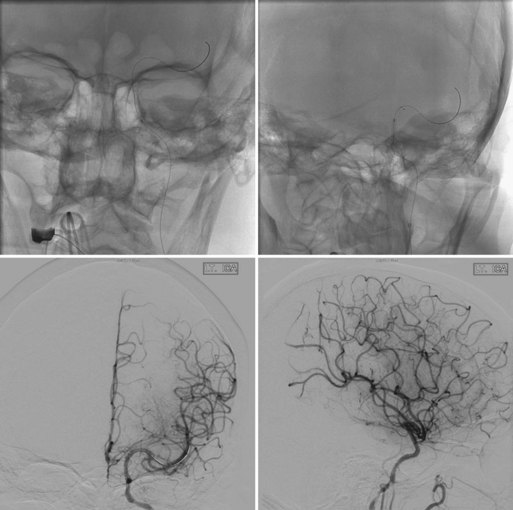
Fig. 47.10 Same patient as in Fig. 47.9. Top x-rays demonstrate balloon positioned across petrocavernous stenosis (black marker is the midpoint of the balloon, left) and stents deployed across petrocavernous and supraclinoid lesions (right). Anteroposterior (left) and lateral (right) post-stenting angiograms on the bottom demonstrate increased flow through stenoses and filling of both anterior and middle cerebral arteries on left.
Clinical Application
There remains controversy as to whether intracranial stent placement is superior to primary angioplasty in the treatment of ICAD. Studies have addressed the angiographic and clinical outcomes associated with each procedure, but relatively few comparative investigations have been reported. A multi-institutional (three tertiary care centers) retrospective study compared the clinical outcomes between primary angioplasty and stent placement for symptomatic intracranial atherosclerosis (Siddiq et al., 2008, 2009). Stroke and combined stroke and/or death were identified as primary clinical endpoints during the periprocedural and follow-up period of 5 years. The authors noted significantly fewer residual stenoses in the stent-treated cohort, but there was no significant reduction in stroke or mortality among the patients who underwent stenting procedures.
In a systematic literature review and meta-analysis, Siddiq et al. (2009) compared primary angioplasty and stent placement in patients with symptomatic intracranial atherosclerosis. They noted technical success rates of 80% in the angioplasty group and 95% in the stent cohort. There was no difference in the perioperative stroke and death rate between the two treatment groups (9% angioplasty, 8% stent). The pooled incidence of 1-year stroke and death was 20% in patients treated with primary angioplasty and 14% in those treated with stenting, signifying a statistically significant difference in outcome. Also noted was a significantly higher restenosis rate in the primary angioplasty group. Importantly, the authors demonstrated no discernable effect of study publication year on the risk of stroke and death.
Debate remains as to the precise indications and preferred treatment strategies for symptomatic ICAD. The Stenting and Aggressive Medical Management for Preventing Recurrent Stroke in Intracranial Stenosis (SAMMPRIS) study is an ongoing large-scale randomized investigation comparing intracranial angioplasty and stenting (Wingspan stent/Gateway balloon) and intensive medical management with optimal medical management alone. Patients with 70% to 99% intracranial stenosis who suffered a stroke or transient ischemic attack (TIA) within 30 days of enrollment are randomized and followed for up to 4 years. This marks the first large-scale randomized study comparing the efficacy of endovascular therapy and medical management in patients with ICAD (Rasmussen, 2009). Intracranial angioplasty with or without stenting is an emerging technique that may have a pivotal position in the treatment of symptomatic intracranial atherosclerosis. The results of SAMMPRIS and other ongoing investigations will better address the precise indications.
Hemorrhagic Stroke
Cerebral Aneurysms
Intracranial aneurysms are abnormal focal dilatations of the cerebral arteries, with thinning and weakening of the vessel wall. Aneurysms constitute a common cerebrovascular pathology, with a reported adult prevalence of 0.2% to 9% worldwide (Inagawa and Hirano, 1990). Vessel degeneration and hemodynamic factors contribute to changes in focal sheer stress and flow patterns that ultimately may result in aneurysmal enlargement or rupture.
Most unruptured aneurysms remain undetected, but an increasing number of incidental aneurysms is being discovered during diagnostic imaging studies (Wakhloo, 2008; Wiebers et al., 2003). Although patients harboring intracranial aneurysms may present with symptoms resulting from mass effect or thromboembolism, the most common and perilous clinical manifestation is that of subarachnoid hemorrhage (SAH). It is estimated that 10 of every 100,000 intracranial aneurysms rupture annually. Aneurysmal rupture carries a roughly 50% mortality rate, with 10% to 20% of survivors failing to regain long-term functional independence (Ellegala and Day, 2005; Hop et al., 1997; Schievink, 1997).
Decision Matrix
Ruptured Aneurysms
Following rupture of an intracranial aneurysm, recurrent hemorrhage occurs at a high frequency. Jane et al. (1985) documented that the incidence of rebleeding among patients with SAH and untreated aneurysms was 50% in the first 6 months and 3% per year thereafter. Because of the high risk of subsequent rupture, these aneurysms are nearly always treated urgently.
The randomized prospective multicenter International Subarachnoid Aneurysm Trial compared the efficacy of endovascular treatment with that of surgery in patients with ruptured intracranial aneurysms suitable for either treatment modality (Molyneux et al., 2002, 2005). A total of 2143 patients were randomized. Recruitment was prematurely terminated after a planned interim analysis demonstrated reduced disability in the endovascular treatment group. In an intention-to-treat analysis, the proportion of patients who were dead or disabled at 1 year was significantly lower in the endovascular therapy group compared to the surgical cohort. Additionally, the risk of perioperative seizures was lower following coiling procedures. However, the requirement for a second procedure was higher with endovascular treatment. The 1-year risk of rebleeding from the ruptured aneurysm was 2 per 1272 patient-years for individuals allocated to embolization and 0 per 1081 in the cohort allocated to open surgery. The investigators noted that the low rates of late rebleeding and potential periprocedural complications associated with retreatment in the endovascular group were unlikely to alter the treatment benefit at 1 year. They concluded that endovascular coil embolization of ruptured intracranial aneurysms when a patient has a good clinical grade (World Federation of Neurosurgical Societies grade I-III) and the aneurysm anatomy is suitable for endovascular treatment is more likely than not to lead to independent survival at 1 year those receiving neurosurgical treatment. Results may be generalized to good-grade patients harboring anterior circulation aneurysms. Statistical comparisons were difficult in poor-grade patients and those with posterior circulation aneurysms, as the vast majority of these individuals were not randomized due to treating physicians’ overwhelming preference for endovascular therapy.
To address issues regarding incomplete occlusion and recanalization following endovascular therapy, rerupture rates after treatment for aneurysmal subarachnoid hemorrhage were further investigated in the Cerebral Aneurysm Rupture After Treatment (CARAT) study (Johnston et al., 2008). All ruptured saccular aneurysms treated between 1996 and 1998 in nine institutions were evaluated for rerupture. A total of 1010 patients (711 surgically clipped, 299 treated by coil embolization) were contacted by written questionnaire or telephone. Rerupture of the treated aneurysm after 1 year occurred in one patient who underwent coil embolization (904 person-years of follow-up, 0.11% annual rate) and no patients treated with surgical clipping (2666 person-years). Aneurysm retreatment after 1 year was more frequent in the endovascular cohort. However, major complications were rare during follow-up treatment. The authors concluded that late events are unlikely to overwhelm differences between the procedures at 1-year follow-up.
Incidental Aneurysms
Rupture rates for intracranial aneurysms vary with size and location, as reported by the International Study of Unruptured Intracranial Aneurysms (International Study of Unruptured Intracranial Aneurysms Investigators, 1998; Wiebers et al., 2003). The prospective observational arm of this large study investigated 2686 unruptured, untreated cerebral aneurysms in 1692 patients. Individuals with no history of SAH who harbor incidental aneurysms located in the anterior circulation (ICA, anterior communicating or anterior cerebral artery, middle cerebral artery) had 5-year cumulative rupture rates of 0%, 2.6%, 14.5%, and 40% for aneurysms of less than 7 mm, 7 to 12 mm, 13 to 24 mm, and 25 mm or greater, respectively. Rupture rates for aneurysms of the same sizes located at the posterior communicating artery or in the posterior circulation were 2.5%, 14.5%, 18.4%, and 50%, respectively. Patients with a prior history of SAH resulting from rupture of an aneurysm distinct from that being studied had a 5-year cumulative rupture rate of 1.5% for aneurysms less than 7 mm in the anterior circulation, compared with 3.4% for aneurysms of the same size located at the posterior communicating artery or in the posterior circulation. Aneurysms greater than 7 mm in this cohort had similar rupture rates to the group with no prior history of SAH (Wiebers et al., 2003).
Therefore, it is generally believed that incidental aneurysms less than 7 mm, located in the anterior circulation, and in patients with no prior history of SAH may be managed expectantly with serial imaging. However, patient-specific desires or the presence of factors such as irregular morphology, documented growth, or a family history of intracranial aneurysms may encourage consideration of surgical or endovascular treatment even in this low-risk cohort (Broderick et al., 2009). Recent studies have focused on quantitative hemodynamic analysis using computer-generated flow models to predict aneurysmal growth and rupture (Chien, 2009). While these methods hold great promise, further development and application to large patient cohorts is required to develop reliable algorithms that accurately predict rupture risk in the clinical setting. According to the ISUIA data, aneurysms of any size located in the posterior circulation and those in patients who have experienced prior SAH carry a significant 5-year cumulative rupture risk and warrant treatment consideration (Wiebers et al., 2003).
There exist no large randomized controlled trials favoring endovascular or surgical management of unruptured intracranial aneurysms. Individualized algorithms must be tailored to specific clinical and anatomical factors when selecting an appropriate treatment paradigm (Raja et al., 2008).
Several large retrospective studies document very low morbidity and mortality rates for surgical clipping in the hands of experienced neurosurgeons (Bederson et al., 2000; Deruty et al., 1996; Dickey et al., 1994; Komotar et al., 2008a; Moroi et al., 2005; Raaymakers et al., 1998). Many authors promote microsurgical treatment in young patients with small anterior circulation aneurysms, suggesting that surgical clipping provides a more durable repair than coil embolization (Komotar et al., 2008a).
Observational studies have advocated surgical clipping for broad-necked aneurysms (Ogilvy et al., 2002; Raftopoulos et al., 2000, 2003; Regli et al., 1999, 2002) and for those with critical vessels emanating from the base (Johnston et al., 2001; Raftopoulos et al., 2000; Regli et al., 1999, 2002).
In 2003, Ogilvy and Carter retrospectively reviewed a series of 604 unruptured aneurysms in an attempt to identify risk factors associated with outcome following surgical treatment. The authors found patient age, aneurysm size, and location within the posterior circulation to be independent predictors of poor outcome (Ogilvy and Carter, 2003).
Emerging data on the endovascular treatment of unruptured intracranial aneurysms suggest low morbidity and mortality rates. Large studies indicate an overall morbidity of 5% to 10%, with mortality rates very close to zero (Johnston et al., 2001; Pouratian et al., 2006; Wiebers et al., 2003). Johnston et al. (2001) compared the safety of endovascular coiling and microsurgical clipping in 2069 patients treated in California between 1990 and 1998. In-hospital mortality and discharge to an alternate facility (rather than home) were significantly less frequent in the endovascular cohort. Mortality was 0.5% in the endovascular group and 3.5% in the open surgical cohort. These results are striking given that the investigation was conducted during the relatively early development of endovascular coiling technology. More recent data from the ISUIA study suggest that endovascular morbidity and mortality may be lower at 1 year than that reported for open surgical treatment in both patients with (7.1% versus 10.1%) and without (9.8% versus 12.6%) prior SAH (Wiebers et al., 2003). Furthermore, outcome following endovascular procedures appeared to be less dependent upon patient age. Direct comparisons were not generated, however, because the endovascular cohort was relatively small. Physician preference led to coil embolization treatment of a greater percentage of older medically ill patients and those with aneurysms located in the posterior circulation.
Endovascular Treatment Modalities
Coil Embolization
Dr. Guglielmi first introduced electrolytically detachable platinum microcoils in 1990. Initially, only aneurysms judged to be high risk for microsurgical clipping were treated by coil embolization. Since that time, rapid technological advancements have resulted in more efficacious endovascular treatment of complex aneurysms. Improved detachment mechanisms, stretch-resistant coils, three-dimensional complex shapes (Piotin et al., 2003; Wakhloo et al., 2007), varying degrees of softness, and coil lattice incorporating a bioabsorbable polymer (Fiorella et al., 2006; Linfante et al., 2005; Murayama et al., 2003) or hydrogel (Cloft, 2006; Gaba et al., 2006) have been designed to minimize procedural complications and help prevent delayed recanalization.
Coil embolization technique involves the careful placement of a framing coil into the aneurysm dome under real-time digital subtraction fluoroscopy. Once appropriate position is confirmed, the coil is detached. Proper selection and methodical placement of the first coil is of paramount importance, as this often establishes technical ease and safety of the entire procedure. If residual aneurysm filling is noted, additional coils of various shapes and successively diminishing sizes are deployed until complete occlusion is obtained (Fig. 47.11).
Balloon Remodeling
Initially described by Moret et al. (1997), the balloon remodeling technique affords an ability to effectively treat broad-necked cerebral aneurysms by mechanically protecting the parent vessel from coil prolapse or protrusion. Positioned within the parent artery, a small balloon occlusion microcatheter is intermittently inflated and deflated across the aneurysm neck (Fig. 47.12). This provides temporary structural support against which coils are then deployed through a second microcatheter located within the aneurysm dome. Anterograde flow is completely reestablished within the parent artery after successive balloon deflations. This adjunctive technique extends the indication for endovascular aneurysm treatment to cases with less favorable anatomy. It is suggested that remodeling leads to higher packing densities and more effective parent vessel reconstruction (Kurre et al., 2008). In a review of their initial experience, the Moret group documented complete angiographic occlusion in 20 of 21 broad-necked cerebral aneurysms treated via balloon-assisted coil embolization. They reported sustained occlusion without recanalization at angiographic follow-up of at least 4 months. The authors noted complication rates that were no greater than those experienced with primary coil embolization techniques (Moret et al., 1997). These results have been corroborated by several more recent clinical series (Cottier et al., 2001; Lefkowitz et al., 1999; Malek et al., 2000). Balloon assistance has greatly facilitated the endovascular treatment of wide-necked ruptured cerebral aneurysms in the acute phase, allowing for mechanical coil support and vessel remodeling in the absence of prolonged antiplatelet therapy.
Stent-Assisted Coil Embolization
In more challenging broad-necked cerebral aneurysms, balloon remodeling may not be sufficient to prevent coil protrusion into the parent vessel. In such cases an endoluminal/endovascular approach uses a permanently deployed intracranial stent as supportive scaffolding. Nitinol self-expanding stents with either open or closed-cell configuration have been designed exclusively for use in the intracranial vasculature (Akpek et al., 2005; Higashida et al., 2005) (Fig. 47.13). Many groups deploy a stent across the aneurysm neck and then introduce the coiling microcatheter into the sac through the stent interstices. An alternative method is to first position the coiling microcatheter within the aneurysm dome and then “jail” the distal tip in position through subsequent stent deployment. With either strategy, stent placement precedes positioning and detachment of the coils within the aneurysm (Figs. 47.14 and 47.15). Clinical studies have documented favorable results, with initial occlusion rates over 80% (Lylyk et al., 2005). While prospective studies have documented persistent complete or near-complete angiographic occlusion at 20 months, long-term data are necessary to determine sustained viability of stent-assisted coiling techniques (Fiorella et al., 2004). Studies have reported the use of complex stent-assisted techniques such as telescoping/overlapping multiple stents for the treatment of fusiform aneurysms (Crowley et al., 2009) and Y-stent configurations for bifurcation aneurysms (Chow et al., 2004). To date, stent-assisted coil embolization has been largely reserved for the treatment of unruptured aneurysms. Most physicians are reluctant to deploy stents in the setting of SAH, because they require aggressive long-term antithrombotic regimens to protect against thrombosis and embolic events.
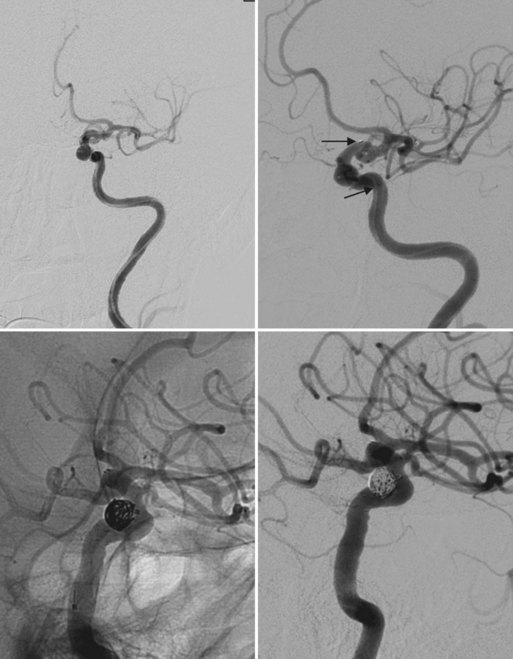
Fig. 47.15 Same aneurysm seen in Fig. 47.12. Large size of aneurysm neck compared to dome led to stent-assisted coiling technique being employed. Top left, Working projection oblique angiogram demonstrating stent tines positioned across neck of aneurysm. Top right, Arrows point to proximal and distal stent tines. Bottom left, Unsubtracted view demonstrating stent position and coil mass. Bottom right, Postintervention angiogram in working projection showing no residual aneurismal neck and no filling within coil mass.
Alternative Treatments
Substantial incomplete occlusion and subsequent recanalization rates following coil embolization have raised concerns regarding the durability of endovascular treatment for cerebral aneurysms with unfavorable geometry (Debrun et al., 1998; Fernandez Zubillaga et al., 1994; Guglielmi et al., 1992; Piske et al., 2009; Taha et al., 2006). Experimental models have demonstrated that coils occupy less than 40% of aneurysmal volume. Therefore, residual flow may remain even in tightly packed lesions (Piotin et al., 2000, 2003). This has prompted the development and application of novel techniques such as liquid embolic agents and flow-diverting stents.
Liquid embolic agents may permit more efficient aneurysmal dome and neck filling. Onyx LES (liquid embolic system [ev3 Inc., Irvine, California]) is an embolic system initially developed to treat high-flow lesions such as AVMs and fistulae. It is an ethylene vinyl alcohol dissolved in the organic solvent, dimethyl sulfoxide (DMSO), that precipitates to form a polymer cast when contacted by aqueous solution. The Cerebral Aneurysm Multicenter European Onyx (CAMEO) study assessed the safety and efficacy of Onyx HD-500 in treating aneurysms that presented difficulties for surgical or endovascular alternatives (wide necks/unfavorable geometry). Balloon remodeling was required in all cases. At 12-month follow-up, the authors reported higher occlusion rates, better outcome, and similar complication rates when compared to those reported with traditional embolization methods in comparable study cohorts (Molyneux et al., 2004). Similar results were noted in several other large case series (Cekirge et al., 2006; Piske et al., 2009).
The advent of flow-diversion devices represents a potential paradigm shift in the treatment strategy for broad-necked intracranial aneurysms. Circumferential endoluminal parent-vessel reconstruction may allow for complete aneurysmal occlusion in the absence of direct endovascular embolization. The Pipeline Embolization Device (PED [ev3 Inc., Irvine, California]) is specifically engineered to reconstruct a segmentally diseased vessel. Lylyk et al. (2009) detailed a large initial experience with the PED; 53 patients with 63 wide-necked aneurysms were treated. The study reported a mean follow-up time of 5.9 months. Complete angiographic occlusion was achieved in 95% of aneurysms, and no major complications were encountered. The investigation found the device to be safe, durable, and curative for selected wide-necked large or giant aneurysms.
Management of Cerebral Vasospasm
Cerebral vasospasm, a delayed reversible narrowing of the intracranial vasculature, is the leading cause of stroke, morbidity, and mortality following neurosurgical and endovascular treatment of aneurysmal subarachnoid hemorrhage (aSAH). Occurring most frequently between 3 and 14 days after aSAH, vasospasm causes a decrease in blood flow and a resultant lowering of cerebral perfusion pressure (Janardhan et al., 2006; Macdonald et al., 2007). Medical treatments including oral nimodipine and induced hypervolemia, hemodilution, and hypertension (“triple-H therapy”) have been employed to enhance cerebral oxygenation in the setting of vasoconstriction. Despite maximal medical measures, 15% of patients who initially survive aSAH experience stroke or death secondary to vasospasm (Mayberg, 1998). For vasospasm refractory to medical management or in patients with treatment contraindications, endovascular therapy has emerged as an alternative or supplementary therapeutic modality (Brisman et al., 2006; Newell et al., 1992). Both balloon angioplasty and IA vasodilator infusion have established roles in the management of medically intractable vasospasm. The optimal method and timing of endovascular treatment, however, remain controversial.
Balloon Angioplasty
A technique first applied by Zubkov et al. in 1984, percutaneous transluminal angioplasty commonly results in permanent mechanical reversal of cerebral vasospasm (Zubkov et al., 1984). The precise mode of action remains poorly understood. Proposed mechanisms for smooth-muscle dysfunction include endothelial denudation, collagen fiber stretching, and rupture of internal elastic lamina (Chan et al., 1995; Honma et al., 1995; Macdonald et al., 1995; Yamamoto et al., 1992).
If severe vasospasm is suspected, percutaneous balloon angioplasty is typically performed under general anesthesia with full muscle paralysis. A noncontrast head CT is obtained immediately prior to angiography to rule out rebleeding, hydrocephalus, or completed infarct. If available, careful examination of a prevasospasm angiogram or vascular study is critical. This allows for assessment of congenitally hypoplastic vascular segments and avoidance of dangerous balloon inflation in unaffected vessels. Vigilant blood pressure control and intracranial pressure monitoring are maintained throughout the treatment. The patient is anticoagulated, and a guide catheter is positioned in the proximal segment of the target vessel. Next, a balloon catheter is advanced over a microguidewire to the level of vessel narrowing. Two differing balloon technologies have been employed. Our preference is the more compliant Hyperglide/Hyperform (ev3 Inc., Irvine, California) or Sentry (Boston Scientific, Natick, Massachusetts) balloon catheters. The catheter lumen distal to the balloon tapers and is effectively occluded by a microguidewire, causing injected contrast to expand the balloon. The guidewire is left in place during balloon inflation and a 50 : 50 mixture of contrast and saline is used to ensure adequate angiographic opacification. Dilatation is performed until vessel conformation is noted in the shape of the balloon. Other groups employ less pliable noncompliant balloon devices such as the Gateway system or Maverick coronary balloon (Boston Scientific, Natick, Massachusetts) (Eddleman et al., 2009). In either case, the balloon is slowly inflated over the length of the accessible spastic segment, attempting to attain dilatation to greater than 50% of the original vessel caliber. Abrupt overdilation is avoided to decrease the risk of rupture and arterial dissection. Balloon angioplasty is generally restricted to larger proximal vessels of the circle of Willis and, less commonly, the smaller immediately more distal branches. Balloon angioplasty can also be effectively used to open proximal vessels and facilitate catheterization of small distal vessels previously inaccessible to microcatheters. This permits potential for subsequent injection of IA vasodilators in the respective distal vascular territories (Fig. 47.16).
An analysis of six retrospective series reporting treatment of cerebral vasospasm by balloon angioplasty demonstrated a mean clinical improvement of 65% (Komotar et al., 2008b). Eskridge et al. (1998) conducted the largest study, examining 50 patients and 170 arterial segments; 61% of patients demonstrated sustained clinical improvement within 72 hours of balloon angioplasty treatment. In a 2005 literature review, Hoh and Ogilvy (2005) found an overall clinical improvement in 62% of patients treated for vasospasm with balloon angioplasty. Rosenwasser et al. (1999) sought to ascertain the time period during which balloon angioplasty was maximally effective. The authors determined that 70% of patients treated within 2 hours of symptom onset demonstrated clinical improvement. By contrast, only 40% of those treated beyond the 2-hour window sustained recovery. Rates of angiographic vasospasm resolution were similar between the two cohorts, indicating that earlier treatment of patients with medically resistant cerebral vasospasm results in superior clinical outcome despite similar radiographic results. Preemptive balloon angioplasty has been advocated for vasospasm prophylaxis in high-risk patients (Muizelaar et al., 1999). A multicenter phase II study published in 2008 that evaluated prophylactic balloon angioplasty in patients with Fisher grade III SAH demonstrated no significant difference in Glasgow Outcome Score when compared to patients treated with standard-of-care therapy. While a statistically significant decrease in the need for therapeutic angioplasty was reported, four procedure-related vessel perforations and three resultant deaths were documented in the prophylaxis cohort (Zwienenberg-Lee et al., 2008).
Intraarterial Vasodilators
Intraarterial vasodilator therapy is often used to treat mild to moderate clinical vasospasm or disease affecting the more distal intracranial vasculature. Administration of IA pharmacological therapy is achieved in a technical fashion similar to that of balloon angioplasty, although often performed under conscious sedation. A guide/diagnostic catheter is advanced into the proximal portion of the affected vessel. The vasodilatory agent is then injected either directly through the larger catheter or via a microcatheter advanced to a more selective distal position within the target vessel. A slow infusion rate coupled with meticulous monitoring is critical in order to avoid systemic hypotension or undesired increases in ICP. To date, the most robust data regarding efficacy of IA pharmacological therapy for the treatment of cerebral vasospasm has been accumulated with papaverine. An opium alkaloid and nonspecific smooth muscle–cell relaxant, papaverine causes vasodilation through cyclic adenosine and guanosine monophosphate phosphodiesterase inhibition and has a half-life of nearly 2 hours. Investigations have documented incidences of immediate angiographic vasospasm relief ranging between 57% and 90% (Firlik et al., 1997; Kassell et al., 1992; Milburn et al., 1998). Hoh and Ogilvy reported in their systematic review that although papaverine produced clinical improvement in 43% of treated patients, the effect was temporary, and individuals often needed multiple treatment sessions. High rates of relapse in clinical vasospasm have been documented within 48 hours of treatment (Hoh and Ogilvy, 2005; Komotar et al., 2008b).
Concerns regarding the toxicity profile and short effect of papaverine have prompted the off-label use of IA calcium channel antagonists in the treatment of cerebral vasospasm (Smith et al., 2004). Feng et al. (2002) reported a 45% increase in vessel diameter and 33% clinical improvement following verapamil administration. Similarly, neurological improvement was documented in 42% of patients receiving IA nicardipine (Badjatia et al., 2004). Biondi et al. (2004) demonstrated a 43% rate of vessel dilation and a 76% incidence of clinical improvement in the first 24 hours following IA administration of nimodipine. The most significant shortcoming associated with the use of IA vasodilator therapy is the transitory efficacy. Repeat endovascular instillation is often necessary (Brisman et al., 2006; Hoh and Ogilvy, 2005). Furthermore, studies have demonstrated that longer durations of vasoconstriction are less responsive to treatment (Scroop, 2004). Side effects including systemic hypotension and increased ICP have further limited the effectiveness of these therapies, each directly related to rate of infusion (McAuliffe et al., 1995).
Cerebral Arteriovenous Malformations
A brain AVM is an aggregate of arterial and venous communications with no intervening capillary network. Studies suggest that the incidence of brain AVMs is 1.21 per 100,000 person-years (Stapf et al., 2002). Despite their congenital nature, cerebral AVMs may become symptomatic at any time, with a mean presentation age of 31.2 (Hofmeister et al., 2000). Over 50% of AVMs manifest with rupture (Brown et al., 1996; Hofmeister et al., 2000), yielding an annual hemorrhage rate between 2% and 4% (Kondziolka et al., 1995; Ondra et al., 1990). Although the resultant blood pattern is most often intracerebral, subarachnoid or intraventricular hemorrhage may occur. In total, AVM rupture accounts for roughly 2% of all intracranial hemorrhages (Stapf et al., 2001, 2002). The risk of rebleeding in patients who initially present with rupture may approach 6% to 17% during the first year before stabilizing to a baseline level after 3 years (Forster et al., 1972; Graf et al., 1983; Mast et al., 1997). Several demographic and anatomical factors including age, deep brain location, and deep venous drainage have been associated with hemorrhage at presentation in patients harboring brain AVMs (Stapf et al., 2006a; Turjman et al., 1995). As many as 35% of patients present with seizures or ischemic symptoms secondary to regional vascular steal phenomenon (Sekhon et al., 1997).
Because the distinct natural history of unruptured AVMs remains uncertain, A Randomized Trial of Unruptured Brain Arteriovenous Malformations (ARUBA) is currently being conducted. This multicenter trial compares a treatment strategy consisting of endovascular, microneurosurgical, and/or radiosurgical therapy to that of conservative management (Stapf et al., 2006b).
Embolization Procedure
A detailed superselective diagnostic cerebral angiogram is necessary to determine the angioarchitecture and flow patterns associated with an AVM prior to endovascular treatment. Penetration of the nidus with embolic material, rather than simple occlusion of the feeding vessels, decreases the incidence of recanalization via flow through collateral channels (Vinuela et al., 1986). Procedural staging reduces the likelihood of edema or hemorrhagic complications secondary to normal perfusion breakthrough (Andrews and Wilson, 1987; Spetzler et al., 1987). Superselective Wada testing with sodium Amytal allows for functional assessment in an awake patient. Infusion may reveal vascular supply to eloquent cortical tissue via en passage vessels not apparent on angiographic assessment (Purdy et al., 1991).
Angiography and embolization are usually performed under general anesthesia with muscle paralysis. If superselective Wada testing is desired, however, the patient is maintained awake for the diagnostic portion of the procedure and is then intubated and administered general anesthesia. Following a detailed diagnostic cerebral angiogram, a guide catheter is positioned in the proximal aspect of the target vessel. Under constant real-time roadmap guidance, an over-the-wire or flow-guided microcatheter is advanced to a distal intracranial position. Most often the microcatheter is advanced into the nidus without the use of a wire, limiting the potential for vessel trauma or perforation. If nidal catheterization cannot be achieved, a more proximal position may be accepted. Biplane superselective angiography is obtained and carefully analyzed for en passage vascular segments or cerebral capillary blush (Fig. 47.17). Several agents have been used for AVM embolization, discussed next.
Polyvinyl Alcohol
Polyvinyl alcohol (PVA) particles (Boston Scientific, Natick, Massachusetts) were the first material approved by the FDA for intravascular use. Dependent on flow, they are sized to the caliber of the target vessel. Consequently, the microcatheter lumen must be able to accommodate the particle diameter and prevent occlusion secondary to aggregation. Particle embolization occurs in a slower fashion than liquid embolic occlusion. Often, low-flow shunts are disconnected first, leading to increased pressure in the remaining feeding arteries and nidal vessels and rendering the AVM susceptible to rupture. Transient and permanent morbidity rates of 14.3% and 8.6%, respectively, have been reported following particle treatment (Schumacher and Horton, 1991). Nidal recanalization is the most significant failing associated with particle embolization. Following proximal occlusion, development of collateral supply results in persistent shunting through the malformation. In a large series, Sorimachi et al. (1999) reported a 43% rate of enlargement following particle embolization and an 80% nidal recanalization rate in completely occluded lesions. Mathis et al. (1995) demonstrated a 12% recanalization rate in AVMs embolized prior to stereotactic radiosurgery.
n-Butyl Cyanoacrylate
n-Butyl cyanoacrylate (nBCA) (TRUFILL [Cordis Neurovascular Inc., Miami Lakes, Florida]) is an adhesive liquid monomeric agent that polymerizes and solidifies upon coming into contact with an ionic solution such as blood (Debrun et al., 1997). Administration results in marked chronic inflammation, fibrosis, and vessel occlusion (Kish et al., 1983; Klara et al., 1985; Wikholm et al., 2001). The safety of nBCA in AVM embolization was assessed in a prospective randomized non-inferiority trial demonstrating that nBCA was equivalent to PVA in achieving target embolization volume goal and reduction in feeding vessel number. The rates of permanent occlusion were more favorable in the nBCA group, with very low incidences of recanalization (n-BCA Trial Investigators, 2002). These results led to FDA approval for this indication. More recent investigations confirm that nBCA embolization results in complete, durable vessel occlusion. Yu et al. (2004) reported no recanalization at 17- to 32-month follow-up in a series of AVMs treated with nBCA. Wikholm et al. (2001) demonstrated a lack of recanalization at 5-year follow-up. Achieving nidal penetration requires modification of polymerization and viscosity parameters according to the hemodynamic characteristics of the lesion. Such factors are adjusted by varying the relative concentrations of Thiodol and nBCA in the treatment mixture. Some groups advocate changing the pH of the solution with an organic acid such as glacial acetic acid to lengthen polymerization time (Lieber et al., 2005). Outcomes following nBCA embolization by experienced operators are favorable. In a series of 103 patients, Liu et al. (2000) reported transient and permanent complications of 4.9% and 1.9%, respectively, as well as two deaths. Gobin et al. (1996) documented morbidity and mortality rates of 12.8% and 1.8%, respectively.
Ethylene Vinyl Alcohol Copolymer
Ethylene vinyl alcohol copolymer was first proposed for the treatment of AVMs by Taki in 1990 (Taki et al., 1990). Subsequently, many groups have reported on its use as a liquid embolic agent (Jahan et al., 2001; Murayama et al., 1998). As noted earlier, Onyx consists of an ethyl-vinyl copolymer dissolved in DMSO, with tantalum powder added for increased radiopacity. Slow solidification permits prolonged controlled injections, with allowance for continuous assessment and deeper nidal penetration with each catheterization (Katsaridis et al., 2008; Murayama et al., 1998). Onyx was first tested in a phase I investigation conducted by Jahan et al. (2001). The group demonstrated a 63% volume reduction in 23 AVM patients with 129 targeted feeding artery pedicles. They reported a 12% transient and 4% permanent associated morbidity. Van Rooij and colleagues treated 44 patients with Onyx, embolizing 138 pedicles in 52 sessions. They documented an average volume reduction of 75%, with complete occlusion of 7 lesions (van Rooij et al., 2007). A morbidity rate of 8.5% and a mortality rate of 3.2% were reported. More recently, several large single-center case series have assessed potential for complete AVM occlusion with Onyx embolization. Katsaridis et al. (2008) reported a 53.9% rate of complete occlusion, with an associated morbidity and mortality of 3.7% and 1.4%, respectively, per embolization session. Using a combination of Onyx and nBCA therapy (Onyx as the first-line agent), Mounayer et al. (2007) reported a 49% complete occlusion rate, with similar incidences of complications (8.5% morbidity, 3.2% mortality).
Although it has revolutionized the way many groups treat brain AVMs, Onyx LES carries inherent limitations and disadvantages. It can be used only with DMSO-compatible catheters. This prohibits the use of most flow-guided microsystems that facilitate access to small distal intracranial vessels. Additionally, DMSO can be directly angiotoxic if injected too rapidly into the cerebral vasculature (Jahan et al., 2001). Furthermore, limited opacification may prevent adequate visualization of reflux into small vessels supplying viable cortical tissue (van Rooij et al., 2007). Recent studies have also suggested that Onyx AVM embolization requires increased fluoroscopy and procedure times compared to nBCA (Velat et al., 2008).
Cerebral Arteriovenous Fistulas
Cranial Dural Arteriovenous Fistulas
Cranial dural arteriovenous fistulas (DAVFs) are direct shunts between arteries and venous sinuses or cortical veins with no transitional capillary network. The mean age of presentation is 50 to 60 years, without gender predilection or evidence for familial inheritance (Zipfel et al., 2009). Although most are presumed to be idiopathic, DAVFs may be associated with trauma, craniotomy or venous thrombosis (Berenstein, Lasjaunias, and Ter Brugge, 2001; Chung et al., 2002). These lesions account for 6% of supratentorial and 35% of posterior fossa vascular malformations (Newton and Cronqvist, 1969). DAVFs present with symptoms according to anatomical localization and pattern of venous drainage. Lesions draining into the cavernous sinus often result in proptosis, chemosis, ophthalmoplegia or increased ocular pressure (Ito et al., 1983; Kim et al., 2002; van Dijk et al., 2004). By contrast, those with venous egress into the transverse or sigmoid sinus frequently present with pulsatile tinnitus (Brown et al., 1994). Posterior fossa DAVFs may cause lower cranial nerve deficits or brainstem findings (Borden et al., 1995; Kim et al., 2002). Lesions draining into the superior saggital sinus can manifest with nonspecific cortical symptoms such as hydrocephalus, seizures, or mental status change (Hirono et al., 1993; Hurst et al., 1998; Kim et al., 2002).
The natural history of DAVFs is directly influenced by cortical venous drainage (CVD), which if present, substantially increases the risk of intracranial hemorrhage and nonhemorrhagic neurological deficits (Borden et al., 1995; Cognard et al., 1995; Zipfel et al., 2009). The critical impact of CVD has been reflected in the angiographic classification systems of Borden and Cognard (Box 47.3), both of which categorize fistulas with cortical venous reflux as high-grade dangerous lesions (Borden et al., 1995; Cognard et al., 1995). Other associated angiographic features, such as venous ectasias and varices, have also been correlated with poor outcome. More recent studies report that individuals who present with symptoms of cortical venous hypertension have a substantially higher risk of new neurological events than those who present incidentally or with symptoms consistent with increased dural sinus drainage (Soderman et al., 2008; Strom et al., 2009).
Box 47.3
Borden and Cognard Classification Systems
Borden Classification System
Type I: dural arteriovenous fistula drainage into a dural venous sinus or meningeal vein with normal anterograde flow. Usually benign clinical behavior.
Type II: anterograde drainage into dural venous sinus and onwards, but retrograde flow is into cortical veins. May present with hemorrhage.
Type III: direct retrograde flow of blood from fistula into cortical veins, causing venous hypertension with a risk of hemorrhage
Cognard Classification System
Type I: normal anterograde flow into a dural venous sinus
Type IIa: drainage into a sinus, with retrograde flow within the sinus
Type IIb: drainage into a sinus, with retrograde flow into cortical vein(s)
Type II a + b: drainage into a sinus, with retrograde flow within the sinus and cortical vein(s)
Type III: direct drainage into a cortical vein, without venous ectasia
Type IV: direct drainage into a cortical vein, with ectasia > 5 mm and 3 times larger than the diameter of the draining vein
From Borden, J.A., Wu, J.K. Shucart, W.A., 1995. A proposed classification for spinal and cranial dural arteriovenous fistulous malformations and implications for treatment. J Neurosurg 82, 166–179; and Cognard, C., Gobin, Y.P., Pierot, L., et al., 1995. Cerebral dural arteriovenous fistulas: clinical and angiographic correlation with a revised classification of venous drainage. Radiology 194, 671–80.
MRI can reveal the presence of a DAVF through flow voids or edema secondary to venous hypertension. Furthermore, dilated vessels may be evident on either CTA or MRA. A catheter angiogram, however, remains the most accurate method of diagnosis and is critical in the assessment of lesional angioarchitecture and venous outflow pattern. Data suggest that lesions with dangerous CVD be addressed urgently (Duffau et al., 1999; van Dijk et al., 2002). Although angiographically benign fistulas may be managed conservatively, those with persistent clinical manifestations often warrant treatment for symptomatic relief.
Treatment options include endovascular occlusion, surgical disconnection, and stereotactic radiosurgery. Embolization, which is the preferred treatment option at most institutions, may be achieved through a transarterial or transvenous access route. Liquid embolic agents such as Onyx or nBCA are typically used alone or in conjunction with thrombogenic coils to attain fistula occlusion. Complete embolization via infiltration of the venous pouch and outflow tract is the ultimate objective. However, partial treatment with selective CVD disconnection is indicated in cases where normal venous drainage through an affected sinus precludes total fistula obliteration. Surgical treatment is typically reserved for lesions not amenable to endovascular therapy or select anterior cranial fossa DAVFs supplied by ethmoidal branches of the ophthalmic artery. Stereotactic radiosurgery is an effective treatment modality used either alone or in conjunction with endovascular therapy (Chandler et al., 1993; Guo et al., 1998; Pollock et al., 1999). However, obliteration occurs over the course of several years, with an inherent interim risk of hemorrhage and neurological events according to the natural history of the specific lesion.
Carotid-Cavernous Fistulas
Carotid-cavernous fistulas (CCFs), abnormal arteriovenous communications in the cavernous sinus, represent a unique subset of cranial arteriovenous fistulas. These lesions may be classified on the basis of etiology (traumatic or spontaneous) or angioarchitecture (direct or indirect). The most commonly used classification system was proposed by Barrow et al. (1985) and segregates lesions according to arterial supply (Box 47.4).
Box 47.4 Grading Scale for Carotid Cavernous Fistulas
Type A (direct): direct communication between the internal carotid artery (ICA) and the cavernous sinus. Usually arises as a result of traumatic laceration or aneurysmal rupture and presents with high flow rate.
Type B (indirect): supplied only by the dural branches of the ICA. Most often arises spontaneously and presents with a lower flow rate.
Type C (indirect): supplied only by dural branches of the external carotid artery (ECA). Most often arises spontaneously and presents with a lower flow rate.
Type D (indirect): supplied by dural branches of the ICA and ECA. Most often arises spontaneously and presents with a lower flow rate.
The classic presentation of direct CCFs is the sudden onset of exophthalmos, conjunctival injection, and a cephalic bruit. Associated cranial nerve palsies and visual decline are common. Indirect CCFs typically have a more gradual onset and mild presentation but may manifest in a similar clinical fashion. Proposed etiologies include pregnancy, sinusitis, trauma, and cavernous sinus thrombosis (Kwan et al., 1989).
Direct CCFs can be approached through transarterial or transvenous access. The standard endovascular treatment of direct CCFs had been transarterial disconnection with a detachable balloon. However, technical problems forced removal of this device from the U.S. market. Current strategies employ the use of detachable coils, liquid embolic agents, and covered stents via either a transarterial or transvenous approach. The transvenous route usually involves a posterior approach through the inferior petrosal sinus (IPS). However, if the IPS is occluded or absent, the superior ophthalmic vein (via the angular branch of the facial vein), inferior ophthalmic vein, superior petrosal sinus, pterygoid plexus, or sphenoparietal sinus may be used for access. A temporary balloon can be inflated in the ICA, across the communication, to assist with visualization and protect the integrity of the vessel lumen. Endovascular occlusion with parent-vessel preservation may not be achievable or necessary for direct CCFs caused by extensive injury to the ICA or those associated with significant steal. In such circumstances, occlusion of the parent artery may be the best (or only) viable management option (Fig. 47.18).
Indirect fistulas may thrombose without vascular intervention (Higashida et al., 1986). However, persistent indirect CCFs are embolized through arterial or venous access routes (listed earlier) in the manner previously described for cranial DAVFs, in general.
Spinal Vascular Malformations
Spinal vascular malformations are a rare and underdiagnosed entity that, if not treated properly, can result in progressive spinal cord symptoms with considerable associated morbidity (Krings and Geibprasert, 2009). A majority of the lesions occur in males and are located in the thoracic or lumbar region of the spinal cord. Dural AVFs frequently cause gradual ascending paraparesis or bowel and bladder dysfunction, whereas intramedullary spinal cord malformations typically present with hemorrhage. While MR studies may reveal flow voids or conus medullaris edema, spinal digital subtraction angiography is necessary for diagnosis, characterization of lesional angioarchitecture, and treatment planning. Spinal angiography is most often performed under general endotracheal anesthesia to limit patient movement due to breathing and facilitate visualization of small segmental vessels.
The arterial supply to the spinal cord is derived from a single anterior spinal artery (ASA) and paired posterior spinal arteries (PSAs). The ASA originates from both vertebral arteries, typically just proximal to the vertebrobasilar junction. It courses through the anterior sulcus of the spinal cord and receives tributaries from radiculomedullary branches of the segmental arteries. The most robust anastomotic supply is provided in the cervical lower thoracic and lumbar regions, with a paucity of radicular connections at the upper thoracic levels. One such anastomotic branch, the artery of lumbar enlargement or artery of Adamkiewicz, provides the main arterial supply to the spinal cord from the lower thoracic region to the conus medullaris. It most often originates from a radicular vessel on the left, between the T8 and L4 levels (McCutcheon et al., 1996). The PSAs originate more proximally from the bilateral vertebral arteries. The paired vessels course along the posterolateral aspect of the spinal cord and anastomose with multiple radiculopial branches of the segmental arteries, which enter the dura through the nerve root sheaths. Classification schemes for spinal vascular malformations are based upon this arterial anatomy (Kim and Spetzler, 2006; Spetzler et al., 2002).
Spinal Dural Arteriovenous Fistula (Type I)
These lesions represent the most common type of spinal vascular malformation and may be supplied by one (type 1a) or multiple (type 1b) dorsal radiculomedullary vessels, which form a fistulous connection with the coronal venous plexus upon entering the dura at the root sleeve (Anson and Spetzler, 1992; Kim and Spetzler, 2006; Sivakumar et al., 2009). They occur most frequently in men older than 50 years of age and classically present with progressive myelopathy secondary to chronic venous hypertension. Endovascular treatment consists of embolization of the fistulous origin, including the proximal portion of the draining vein (van Dijk et al., 2002). Open surgical disconnection may be employed to achieve a similar result.
Glomus Arteriovenous Malformation (Type II)
Most similar to intracranial AVMs, these lesions are supplied by perforating braches of the anterior or posterior spinal arteries and drain into the venous plexus surrounding the spinal cord (Kim et al., 2006; Spetzler et al., 2002; Wakhloo, 2008). A high-pressure, high-flow nidus may be diffuse or compact and is often associated with a feeding artery or intranidal aneurysms. These lesions most frequently occur in younger men and women and frequently present with hemorrhage. Embolization is usually employed as an adjunct to surgical resection.
Juvenile/Metameric Arteriovenous Malformation (Type III)
Found predominantly in children and young adults, these lesions are fed by multiple arterial pedicles and affect bone and soft tissue in addition to spinal cord parenchyma (Kim and Spetzler, 2006; Spetzler et al., 2002; Wakhloo, 2008). Involvement of an entire metamere including skin, soft tissue, bone, and spinal cord parenchyma is referred to as Cobb syndrome. Multimodality therapy is frequently indicated because of the complex nature of these malformations.
Perimedullary Arteriovenous Malformation (Type IV)
Vascular supply to these intradural extramedullary fistulas is derived from either the anterior (most commonly) or posterior spinal arteries. They occur most frequently between the ages of 30 and 60 and often present with progressive neurological deficits (Anson and Spetzler, 1992; Heros et al., 1986). The treatment paradigm is dependent on the angioarchitecture of the lesion, including the number of feeding pedicles and rate of fistulous flow. Surgical disconnection has been successfully employed for low-flow fistulas supplied by a single feeding artery, while a combination of endovascular and surgical techniques have commonly been used for the management of more complex lesions.
Tumor Embolization
Preoperative embolization of extracranial head and neck or intracranial tumors is a useful adjunct to microsurgical resection. Tumors most commonly treated by this means are meningiomas, glomus tumors, juvenile nasopharyngeal angiofibromas, hemangioblastomas, sarcomas, head and neck squamous cell carcinomas, and choroid plexus tumors (Eskridge et al., 1996; Scholtz et al., 2001). These lesions are often quite vascular, so preoperative embolization can ease complete resection by diminishing surgical time and intraoperative blood loss (Dowd et al., 2003; Gruber et al., 2000; Macpherson, 1991; Manelfe et al., 1986). Many cranial-base tumors are characterized by vascular pedicles that are medially located with respect to the operative approach (Rosen et al., 2002). In such cases, the surgical corridor is often narrow and deep, which amplifies the difficulty of resection. In addition to improving operative safety and visualization, preoperative embolization is believed to reduce transmitted forces to adjacent neural tissues during surgical resection by causing ischemic necrosis and a resultant softening of the tumor mass (Yoon et al., 2008). In selected patients who are not suitable candidates for surgery, embolization can be used for palliation, with a goal of size and tumor growth reduction. Because the primary blood supply is most frequently derived from the external carotid artery, a thorough understanding of the head, neck, and intracranial vascular anatomy is critical.
Embolization Procedure
After a diagnostic cerebral angiogram is performed to identify blood supply and assess for feasibility and safety of embolization, a microcatheter is advanced over a microguidewire through the larger diagnostic or guide catheter. Under real-time digital subtraction fluoroscopy, the microsystem is advanced into the vascular pedicle supplying the tumor. Superselective angiography is performed through the microcatheter to confirm proper position, identify any distal branches supplying normal tissue, and assess for the presence of dangerous anastomoses. Provocative testing with IA sodium Amytal or lidocaine may aide in the detection of unsafe collaterals or cranial nerve supply distal to the microcatheter tip (Dowd et al., 2003; Yoon et al., 2008). After proper positioning of the microcatheter, injection of embolic material is performed under constant real-time digital subtraction fluoroscopy. Distal tumoral penetration prevents flow to the mass via collateral vessels and results in devascularization and subsequent tumor necrosis (Fig. 47.19). Direct tumor puncture is an alternate means of accessing hypervascular neoplasms of the head and neck (Abud et al., 2004; Chaloupka et al., 1999). This technique may be used alone or in conjunction with transfemoral embolization.
Embolization Materials
A number of agents have been used to embolize tumors, including particles (Bendszus et al., 2000; Wakhloo et al., 1993), nBCA (Capo et al., 1991), Onyx (Shi et al., 2008), platinum coils (Guglielmi, 1998), ethanol (Jungreis, 1991), fibrin glue (Probst et al., 1999), and gelfoam pledgets (Manelfe et al., 1986; Richter and Schachenmayr, 1983). Particulate agents are generally favored over liquid embolic materials owing to their ease of use and relative safety. The precise penetration capacity of particles is dependent on their size, so appropriate selection precludes infiltration into the small arterioles supplying cranial nerves. Since the vasa nervorum are small, the use of particles larger than 150 microns in diameter has been suggested to lessen the risk of ischemic cranial nerve injury (Bendszus et al., 2005; Carli et al., 2010). PVA particles are believed to remain occlusive in vessels for weeks, after which vascular recanalization may occur (Kuroiwa et al., 1996). This property is suitable for preoperative tumor embolization. Because the tumor mass is excised during definitive surgical resection, a long-term durable embolization is not necessary. These agents have been shown by several groups to be safe and effective in decreasing intraoperative blood loss in paraganglioma surgery (Persky et al., 2002; Tikkakoski et al., 1997). After particle embolization is performed, a platinum coil or gelfoam pledget may be placed more proximally in the treated vessel to help prevent recanalization and aid in surgical transection (Dowd et al., 2003). In contrast to particles, liquid embolic materials such as nBCA and Onyx can more easily enter anastomotic channels that supply cranial nerves or cortical vessels not evident on the initial diagnostic angiogram. The operator must be acutely aware of this possibility when using such agents for tumor embolization. Additionally, microcatheter retention can result in vessel rupture or ischemic sequelae. As more aggressive liquid embolic agents are often used for palliative embolization, care must be taken to avoid proximal reflux of any embolic material into eloquent vascular branches.
Vessel Selection
Determining target vessels for endovascular therapy may be difficult. Embolization can effectively obliterate high-flow arteriovenous shunting through the tumor vasculature. Most agree that large external carotid artery branches that directly supply a tumor mass and are distant from dangerous anastomoses may be treated. However, intracranial tumors supplied by both the external and internal carotid artery are reported to exhibit increased pial supply subsequent to embolization of external branches (Manelfe et al., 1986). Augmented blood flow and hypertrophy of the small, deep intracranial vessels may increase the difficulty of the surgical resection, since this vascular supply is often not encountered until the end of the operation. Unless necessary, preoperative embolization of pial blood supply is generally avoided; the benefit of embolization is usually outweighed by the potential risk of ischemia.
Clinical Evidence
Several large case series document angiographic, histological, and surgical benefit following meningioma embolization (Dean et al., 1994; Gruber et al., 2000; Macpherson, 1991; Manelfe et al., 1986; Wakhloo et al., 1993; Yoon et al., 2008). Endovascular treatment of juvenile nasopharyngeal angiofibromas has been shown to reduce both perioperative blood loss and the duration of surgical resection (Davis, 1987; Economou et al., 1988; Roberson et al., 1979). Other reports question the benefit, citing no differences in operative time, blood loss, or clinical outcome (Bendszus et al., 2000). Some groups reserve preoperative embolization for difficult skull-base lesions, referencing complication rates deemed unacceptable for straightforward surgical resections (Rosen et al., 2002). The optimal timing of resection following tumor embolization has been debated. Some operators contend that timing of surgery does not affect outcome, but others have advocated delayed resection to allow for interval tumor necrosis. A comparative retrospective analysis of 50 patients who underwent preoperative embolization for meningioma resection indicated that delaying resection more than 24 hours after embolization can decrease intraoperative blood loss (Chun et al., 2002). Other groups have suggested that the optimal interval between embolization and resection is between 7 and 9 days, as this permits maximal tumor softening (Kai et al., 2002). A significantly long interval between particulate embolization and surgical resection, however, may allow for recanalization of blood vessels supplying the tumor.
Significant neurological deficits have been reported following preoperative tumor embolization. Causes include distal vessel occlusion, reflux of embolic agents, cranial nerve injury, and tumor swelling or hemorrhage following devascularization (Bendszus et al., 2005; Carli et al., 2010; Marangos and Schumacher, 1999). Therefore, potential benefits of embolization must be carefully weighed against the risk of adverse outcome. Patients harboring large hypervascular tumors supplied primarily by external carotid artery branches that are located in anatomical regions difficult to access surgically likely derive the greatest benefit from preoperative embolization.
Abud D.G., Mounayer C., Benndorf G., et al. Intratumoral injection of cyanoacrylate glue in head and neck paragangliomas. AJNR Am J Neuroradiol. 2004;25:1457-1462.
Akpek S., Arat A., Morsi H., et al. Self-expandable stent-assisted coiling of wide-necked intracranial aneurysms: a single-center experience. AJNR Am J Neuroradiol. 2005;26:1223-1231.
Alberts M.J. Publications Committee of the WALLSTENT. Results of a multicenter prospective randomized trial of carotid artery stenting vs. carotid endarterectomy. Stroke. 2001;32:325.
Andrews B.T., Wilson C.B. Staged treatment of arteriovenous malformations of the brain. Neurosurgery. 1987;21:314-323.
Anson J.A., Spetzler R.F. Interventional neuroradiology for spinal pathology. Clin Neurosurg. 1992;39:388-417.
Badjatia N., Topcuoglu M.A., Pryor J.C., et al. Preliminary experience with intra-arterial nicardipine as a treatment for cerebral vasospasm. AJNR Am J Neuroradiol. 2004;25:819-826.
Barber P.A., Zhang J., Demchuk A.M., et al. Why are stroke patients excluded from TPA therapy? An analysis of patient eligibility. Neurology. 2001;56:1015-1020.
Barnett H.J., Taylor D.W., Eliasziw M., et al. Benefit of carotid endarterectomy in patients with symptomatic moderate or severe stenosis. North American Symptomatic Carotid Endarterectomy Trial Collaborators. N Engl J Med. 1998;339:1415-1425.
Barrow D.L., Spector R.H., Braun I.F., et al. Classification and treatment of spontaneous carotid-cavernous sinus fistulas. J Neurosurg. 1985;62:248-256.
Bederson J.B., Awad I.A., Wiebers D.O., et al. Recommendations for the management of patients with unruptured intracranial aneurysms: a statement for healthcare professionals from the Stroke Council of the American Heart Association. Stroke. 2000;31:2742-2750.
Bendszus M., Monoranu C.M., Schutz A., et al. Neurologic complications after particle embolization of intracranial meningiomas. AJNR Am J Neuroradiol. 2005;26:1413-1419.
Bendszus M., Rao G., Burger R., et al. Is there a benefit of preoperative meningioma embolization? Neurosurgery. 2000;47:1306-1311. discussion 1311-1312
Berenstein A.L., Lasjaunias P., Ter Brugge K. Surgical Neuroangiography:1 Clinical Vascular Anatomy and Variations. Berlin: Springer-Verlag, 2001.
Biller J., Feinberg W.M., Castaldo J.E., et al. Guidelines for carotid endarterectomy: a statement for healthcare professionals from a Special Writing Group of the Stroke Council, American Heart Association. Circulation. 1998;97:501-509.
Biondi A., Ricciardi G.K., Puybasset L., et al. Intra-arterial nimodipine for the treatment of symptomatic cerebral vasospasm after aneurysmal subarachnoid hemorrhage: preliminary results. AJNR Am J Neuroradiol. 2004;25:1067-1076.
Borden J.A., Wu J.K., Shucart W.A. A proposed classification for spinal and cranial dural arteriovenous fistulous malformations and implications for treatment. J Neurosurg. 1995;82:166-179.
Bose A., Hartmann M., Henkes H., et al. A novel, self-expanding, nitinol stent in medically refractory intracranial atherosclerotic stenoses: the Wingspan study. Stroke. 2007;38:1531-1537.
Bose A., Henkes H., Alfke K., et al. The Penumbra System: a mechanical device for the treatment of acute stroke due to thromboembolism. AJNR Am J Neuroradiol. 2008;29:1409-1413.
Brisman J.L., Eskridge J.M., Newell D.W. Neurointerventional treatment of vasospasm. Neurol Res. 2006;28:769-776.
Broderick J.P., Brown R.D.Jr., Sauerbeck L., et al. Greater rupture risk for familial as compared to sporadic unruptured intracranial aneurysms. Stroke. 2009;40:1952-1957.
Brott T.G., Hobson R.W.2nd, Howard G., et al. Stenting versus endarterectomy for treatment of carotid-artery stenosis. N Engl J Med.. 2010.
Brown R.D.Jr., Wiebers D.O., Nichols D.A. Intracranial dural arteriovenous fistulae: angiographic predictors of intracranial hemorrhage and clinical outcome in nonsurgical patients. J Neurosurg. 1994;81:531-538.
Brown R.D.Jr., Wiebers D.O., Torner J.C., et al. Frequency of intracranial hemorrhage as a presenting symptom and subtype analysis: a population-based study of intracranial vascular malformations in Olmsted County, Minnesota. J Neurosurg. 1996;85:29-32.
Capo H., Kupersmith M.J., Berenstein A., et al. The clinical importance of the inferolateral trunk of the internal carotid artery. Neurosurgery. 1991;28:733-737. discussion 737-738
CARESS steering committee. Carotid revascularization using endarterectomy or stenting systems (CARESS): phase I clinical trial. J Endovasc Ther. 2003;10:1021-1030.
Carli D.F., Sluzewski M., Beute G.N., et al. Complications of particle embolization of meningiomas: frequency, risk factors, and outcome. AJNR Am J Neuroradiol. 2010;31:152-154.
CAVATAS Investigators. Endovascular versus surgical treatment in patients with carotid stenosis in the Carotid and Vertebral Artery Transluminal Angioplasty Study (CAVATAS): a randomised trial. Lancet. 2001;357:1729-1737.
Cekirge H.S., Saatci I., Geyik S., et al. Intrasaccular combination of metallic coils and onyx liquid embolic agent for the endovascular treatment of cerebral aneurysms. J Neurosurg. 2006;105:706-712.
Chaloupka J.C., Mangla S., Huddle D.C., et al. Evolving experience with direct puncture therapeutic embolization for adjunctive and palliative management of head and neck hypervascular neoplasms. Laryngoscope. 1999;109:1864-1872.
Chan P.D., Findlay J.M., Vollrath B., et al. Pharmacological and morphological effects of in vitro transluminal balloon angioplasty on normal and vasospastic canine basilar arteries. J Neurosurg. 1995;83:522-530.
Chandler H.C.Jr., Friedman W.A. Successful radiosurgical treatment of a dural arteriovenous malformation: case report. Neurosurgery. 1993;33:139-141. discussion 141-142
Chien A., Castro M.A., Tateshima S., et al. Quantitative hemodynamic analysis of brain aneurysms at different locations. AJNR Am J Neuroradiol. 2009;30:1507-1512.
Chimowitz M.I., Lynn M.J., Howlett-Smith H., et al. Comparison of warfarin and aspirin for symptomatic intracranial arterial stenosis. N Engl J Med. 2005;352:1305-1316.
Chow M.M., Masaryk T.J., Woo H.H., et al. Stent-assisted angioplasty of intracranial vertebrobasilar atherosclerosis: midterm analysis of clinical and radiologic predictors of neurological morbidity and mortality. AJNR Am J Neuroradiol. 2005;26:869-874.
Chow M.M., Woo H.H., Masaryk T.J., et al. A novel endovascular treatment of a wide-necked basilar apex aneurysm by using a Y-configuration, double-stent technique. AJNR Am J Neuroradiol. 2004;25:509-512.
Chun J.Y., Mcdermott M.W., Lamborn K.R., et al. Delayed surgical resection reduces intraoperative blood loss for embolized meningiomas. Neurosurgery. 2002;50:1231-1235. discussion 1235-1237
Chung S.J., Kim J.S., Kim J.C., et al. Intracranial dural arteriovenous fistulas: analysis of 60 patients. Cerebrovasc Dis. 2002;13:79-88.
Cloft H.J. HydroCoil for Endovascular Aneurysm Occlusion (HEAL) study: periprocedural results. AJNR Am J Neuroradiol. 2006;27:289-292.
Cognard C., Gobin Y.P., Pierot L., et al. Cerebral dural arteriovenous fistulas: clinical and angiographic correlation with a revised classification of venous drainage. Radiology. 1995;194:671-680.
Connors J.J.3rd, Wojak J.C. Percutaneous transluminal angioplasty for intracranial atherosclerotic lesions: evolution of technique and short-term results. J Neurosurg. 1999;91:415-423.
Cottier J.P., Pasco A., Gallas S., et al. Utility of balloon-assisted Guglielmi detachable coiling in the treatment of 49 cerebral aneurysms: a retrospective, multicenter study. AJNR Am J Neuroradiol. 2001;22:345-351.
Crowley R.W., Evans A.J., Kassell N.F., et al. Endovascular treatment of a fusiform basilar artery aneurysm using multiple “in-stent stents.” Technical note. J Neurosurg Pediatr. 2009;3:496-500.
Davis K.R. Embolization of epistaxis and juvenile nasopharyngeal angiofibromas. AJR Am J Roentgenol. 1987;148:209-218.
Dean B.L., Flom R.A., Wallace R.C., et al. Efficacy of endovascular treatment of meningiomas: evaluation with matched samples. AJNR Am J Neuroradiol. 1994;15:1675-1680.
Debrun G.M., Aletich V., Ausman J.I., et al. Embolization of the nidus of brain arteriovenous malformations with n-butyl cyanoacrylate. Neurosurgery. 1997;40:112-120. discussion 120-121
Debrun G.M., Aletich V.A., Kehrli P., et al. Aneurysm geometry: an important criterion in selecting patients for Guglielmi detachable coiling. Neurol Med Chir (Tokyo). 1998;38(Suppl):1-20.
Deruty R., Pelissou-Guyotat I., Mottolese C., et al. Management of unruptured cerebral aneurysms. Neurol Res. 1996;18:39-44.
Dickey P., Nunes J., Bautista C., et al. Intracranial aneurysms: size, risk of rupture, and prophylactic surgical treatment. Conn Med. 1994;58:583-586.
Dowd C.F., Halbach V.V., Higashida R.T. Meningiomas: the role of preoperative angiography and embolization. Neurosurg Focus. 2003;15:E10.
Duffau H., Lopes M., Janosevic V., et al. Early rebleeding from intracranial dural arteriovenous fistulas: report of 20 cases and review of the literature. J Neurosurg. 1999;90:78-84.
Economou T.S., Abemayor E., Ward P.H. Juvenile nasopharyngeal angiofibroma: an update of the UCLA experience, 1960-1985. Laryngoscope. 1988;98:170-175.
Eddleman C.S., Hurley M.C., Naidech A.M., et al. Endovascular options in the treatment of delayed ischemic neurological deficits due to cerebral vasospasm. Neurosurg Focus. 2009;26:E6.
Ederle J., Dobson J., Featherstone R.L., et al. Carotid artery stenting compared with endarterectomy in patients with symptomatic carotid stenosis (International Carotid Stenting Study): an interim analysis of a randomised controlled trial. Lancet. 2010;375:985-997.
Ellegala D.B., Day A.L. Ruptured cerebral aneurysms. N Engl J Med. 2005;352:121-124.
Eskridge J.M., McAuliffe W., Harris B., et al. Preoperative endovascular embolization of craniospinal hemangioblastomas. AJNR Am J Neuroradiol. 1996;17:525-531.
Eskridge J.M., McAuliffe W., Song J.K., et al. Balloon angioplasty for the treatment of vasospasm: results of first 50 cases. Neurosurgery. 1998;42:510-516. discussion 516-7
European Carotid Surgery Trialists’ Collaborative Group. Endarterectomy for moderate symptomatic carotid stenosis: interim results from the MRC European Carotid Surgery Trial. Lancet. 1996;347:1591-1593.
Executive Committee for the Asymptomatic Carotid Atherosclerosis Study. Endarterectomy for asymptomatic carotid artery stenosis. Executive Committee for the Asymptomatic Carotid Atherosclerosis Study. JAMA. 1995;273:1421-1428.
Feldman R.L., Trigg L., Gaudier J., et al. Use of coronary Palmaz-Schatz stent in the percutaneous treatment of an intracranial carotid artery stenosis. Cathet Cardiovasc Diagn. 1996;38:316-319.
Feng L., Fitzsimmons B.F., Young W.L., et al. Intraarterially administered verapamil as adjunct therapy for cerebral vasospasm: safety and 2-year experience. AJNR Am J Neuroradiol. 2002;23:1284-1290.
Fernandez Zubillaga A., Guglielmi G., Vinuela F., et al. Endovascular occlusion of intracranial aneurysms with electrically detachable coils: correlation of aneurysm neck size and treatment results. AJNR Am J Neuroradiol. 1994;15:815-820.
Fiorella D., Albuquerque F.C., Han P., et al. Preliminary experience using the Neuroform stent for the treatment of cerebral aneurysms. Neurosurgery. 2004;54:6-16. discussion 16-17
Fiorella D., Albuquerque F.C., Mcdougall C.G. Durability of aneurysm embolization with matrix detachable coils. Neurosurgery. 2006;58:51-59. discussion 51-59
Fiorella D., Woo H.H. Emerging endovascular therapies for symptomatic intracranial atherosclerotic disease. Stroke. 2007;38:2391-2396.
Firlik A.D., Kaufmann A.M., Jungreis C.A., et al. Effect of transluminal angioplasty on cerebral blood flow in the management of symptomatic vasospasm following aneurysmal subarachnoid hemorrhage. J Neurosurg. 1997;86:830-839.
Forster D.M., Steiner L., Hakanson S. Arteriovenous malformations of the brain. A long-term clinical study. J Neurosurg. 1972;37:562-570.
Furlan A., Higashida R., Wechsler L., et al. Intra-arterial prourokinase for acute ischemic stroke. The PROACT II study: a randomized controlled trial. Prolyse in Acute Cerebral Thromboembolism. JAMA. 1999;282:2003-2011.
Gaba R.C., Ansari S.A., Roy S.S., et al. Embolization of intracranial aneurysms with hydrogel-coated coils versus inert platinum coils: effects on packing density, coil length and quantity, procedure performance, cost, length of hospital stay, and durability of therapy. Stroke. 2006;37:1443-1450.
Gaines P.A., Randall M.S. Carotid artery stenting for patients with asymptomatic carotid disease (and news on TACIT). Eur J Vasc Endovasc Surg. 2005;30:461-463.
Gandhi C.D., Christiano L.D., Prestigiacomo C.J. Endovascular management of acute ischemic stroke. Neurosurg Focus. 2009;26:E2.
Garas S.M., Huber P., Scott N.A. Overview of therapies for prevention of restenosis after coronary interventions. Pharmacol Ther. 2001;92:165-178.
George C.J., Baim D.S., Brinker J.A., et al. One-year follow-up of the Stent Restenosis (STRESS I) Study. Am J Cardiol. 1998;81:860-865.
Gobin Y.P., Laurent A., Merienne L., et al. Treatment of brain arteriovenous malformations by embolization and radiosurgery. J Neurosurg. 1996;85:19-28.
Gomez C.R., Misra V.K., Campbell M.S., et al. Elective stenting of symptomatic middle cerebral artery stenosis. AJNR Am J Neuroradiol. 2000;21:971-973.
Graf C.J., Perret G.E., Torner J.C. Bleeding from cerebral arteriovenous malformations as part of their natural history. J Neurosurg. 1983;58:331-337.
Gruber A., Killer M., Mazal P., et al. Preoperative embolization of intracranial meningiomas: a 17-years single center experience. Minim Invasive Neurosurg. 2000;43:18-29.
Guglielmi G. Use of the GDC crescent for embolization of tumors fed by cavernous and petrous branches of the internal carotid artery. Technical note. J Neurosurg. 1998;89:857-860.
Guglielmi G., Vinuela F., Duckwiler G., et al. Endovascular treatment of posterior circulation aneurysms by electrothrombosis using electrically detachable coils. J Neurosurg. 1992;77:515-524.
Guo W.Y., Pan D.H., Wu H.M., et al. Radiosurgery as a treatment alternative for dural arteriovenous fistulas of the cavernous sinus. AJNR Am J Neuroradiol. 1998;19:1081-1087.
Gupta R., Al-Ali F., Thomas A.J., et al. Safety, feasibility, and short-term follow-up of drug-eluting stent placement in the intracranial and extracranial circulation. Stroke. 2006;37:2562-2566.
Gurm H.S., Yadav J.S., Fayad P., et al. Long-term results of carotid stenting versus endarterectomy in high-risk patients. N Engl J Med. 2008;358:1572-1579.
Hacke W., Kaste M., Bluhmki E., et al. Thrombolysis with alteplase 3 to 4.5 hours after acute ischemic stroke. N Engl J Med. 2008;359:1317-1329.
Halliday A., Mansfield A., Marro J., et al. Prevention of disabling and fatal strokes by successful carotid endarterectomy in patients without recent neurological symptoms: randomised controlled trial. Lancet. 2004;363:1491-1502.
Henkes H., Miloslavski E., Lowens S., et al. Treatment of intracranial atherosclerotic stenoses with balloon dilatation and self-expanding stent deployment (wingspan). Neuroradiology. 2005;47:222-228.
Heros R.C., Debrun G.M., Ojemann R.G., et al. Direct spinal arteriovenous fistula: a new type of spinal AVM. Case report. J Neurosurg. 1986;64:134-139.
Higashida R.T., Halbach V.V., Dowd C.F., et al. Initial clinical experience with a new self-expanding nitinol stent for the treatment of intracranial cerebral aneurysms: the Cordis Enterprise stent. AJNR Am J Neuroradiol. 2005;26:1751-1756.
Higashida R.T., Hieshima G.B., Halbach V.V., et al. Closure of carotid cavernous sinus fistulae by external compression of the carotid artery and jugular vein. Acta Radiol Suppl. 1986;369:580-583.
Hirono N., Yamadori A., Komiyama M. Dural arteriovenous fistula: a cause of hypoperfusion-induced intellectual impairment. Eur Neurol. 1993;33:5-8.
Hobson R.W.2nd, Howard V.J., Roubin G.S., et al. Credentialing of surgeons as interventionalists for carotid artery stenting: experience from the lead-in phase of CREST. J Vasc Surg. 2004;40:952-957.
Hofmeister C., Stapf C., Hartmann A., et al. Demographic, morphological, and clinical characteristics of 1289 patients with brain arteriovenous malformation. Stroke. 2000;31:1307-1310.
Hoh B.L., Ogilvy C.S. Endovascular treatment of cerebral vasospasm: transluminal balloon angioplasty, intra-arterial papaverine, and intra-arterial nicardipine. Neurosurg Clin N Am. 2005;16:501-516. vi
Honma Y., Fujiwara T., Irie K., et al. Morphological changes in human cerebral arteries after percutaneous transluminal angioplasty for vasospasm caused by subarachnoid hemorrhage. Neurosurgery. 1995;36:1073-1080. discussion 1080-1081
Hop J.W., Rinkel G.J., Algra A., et al. Case-fatality rates and functional outcome after subarachnoid hemorrhage: a systematic review. Stroke. 1997;28:660-664.
Hurst R.W., Bagley L.J., Galetta S., et al. Dementia resulting from dural arteriovenous fistulas: the pathologic findings of venous hypertensive encephalopathy. AJNR Am J Neuroradiol. 1998;19:1267-1273.
IMS Study Investigators. Combined intravenous and intra-arterial recanalization for acute ischemic stroke: the Interventional Management of Stroke Study. Stroke. 2004;35:904-911.
IMS II Trial Investigators. The Interventional Management of Stroke (IMS) II Study. Stroke. 2007;38:2127-2135.
Inagawa T., Hirano A. Ruptured intracranial aneurysms: an autopsy study of 133 patients. Surg Neurol. 1990;33:117-123.
International Study of Unruptured Intracranial Aneurysms Investigators. Unruptured intracranial aneurysms–risk of rupture and risks of surgical intervention. International Study of Unruptured Intracranial Aneurysms Investigators. N Engl J Med. 1998;339:1725-1733.
Ito J., Imamura H., Kobayashi K., et al. Dural arteriovenous malformations of the base of the anterior cranial fossa. Neuroradiology. 1983;24:149-154.
Jahan R., Murayama Y., Gobin Y.P., et al. Embolization of arteriovenous malformations with Onyx: clinicopathological experience in 23 patients. Neurosurgery. 2001;48:984-995. discussion 995-997
Janardhan V., Biondi A., Riina H.A., et al. Vasospasm in aneurysmal subarachnoid hemorrhage: diagnosis, prevention, and management. Neuroimaging Clin N Am. 2006;16:483-496. viii-ix
Jane J.A., Kassell N.F., Torner J.C., et al. The natural history of aneurysms and arteriovenous malformations. J Neurosurg. 1985;62:321-323.
Jiang W.J., Wang Y.J., Du B., et al. Stenting of symptomatic M1 stenosis of middle cerebral artery: an initial experience of 40 patients. Stroke. 2004;35:1375-1380.
Johnston S.C., Dowd C.F., Higashida R.T., et al. Predictors of rehemorrhage after treatment of ruptured intracranial aneurysms: the Cerebral Aneurysm Rerupture After Treatment (CARAT) study. Stroke. 2008;39:120-125.
Johnston S.C., Zhao S., Dudley R.A., et al. Treatment of unruptured cerebral aneurysms in California. Stroke. 2001;32:597-605.
Jungreis C.A. Skull-base tumors: ethanol embolization of the cavernous carotid artery. Radiology. 1991;181:741-743.
Kai Y., Hamada J., Morioka M., et al. Appropriate interval between embolization and surgery in patients with meningioma. AJNR Am J Neuroradiol. 2002;23:139-142.
Kassell N.F., Helm G., Simmons N., et al. Treatment of cerebral vasospasm with intra-arterial papaverine. J Neurosurg. 1992;77:848-852.
Katsaridis V., Papagiannaki C., Aimar E. Curative embolization of cerebral arteriovenous malformations (AVMs) with Onyx in 101 patients. Neuroradiology. 2008;50:589-597.
Katz J.M., Gobin Y.P. Merci Retriever in acute stroke treatment. Expert Rev Med Devices. 2006;3:273-280.
Kim J.K., Ahn J.Y., Lee B.H., et al. Elective stenting for symptomatic middle cerebral artery stenosis presenting as transient ischaemic deficits or stroke attacks: short term arteriographical and clinical outcome. J Neurol Neurosurg Psychiatry. 2004;75:847-851.
Kim L.J., Spetzler R.F. Classification and surgical management of spinal arteriovenous lesions: arteriovenous fistulae and arteriovenous malformations. Neurosurgery. 2006;59:S195-S201. discussion S3-13
Kim M.S., Han D.H., Kwon O.K., et al. Clinical characteristics of dural arteriovenous fistula. J Clin Neurosci. 2002;9:147-155.
Kish K.K., Rapp S.M., Wilner H.I., et al. Histopathologic effects of transarterial bucrylate occlusion of intracerebral arteries in mongrel dogs. AJNR Am J Neuroradiol. 1983;4:385-387.
Kiyosue H., Okahara M., Yamashita M., et al. Endovascular stenting for restenosis of the intracranial vertebrobasilar artery after balloon angioplasty: two case reports and review of the literature. Cardiovasc Intervent Radiol. 2004;27:538-543.
Klara P.M., George E.D., Mcdonnell D.E., et al. Morphological studies of human arteriovenous malformations. Effects of isobutyl 2-cyanoacrylate embolization. J Neurosurg. 1985;63:421-425.
Komotar R.J., Mocco J., Solomon R.A. Guidelines for the surgical treatment of unruptured intracranial aneurysms: the first annual J. Lawrence pool memorial research symposium–controversies in the management of cerebral aneurysms. Neurosurgery. 2008;62:183-193. discussion 193-194
Komotar R.J., Zacharia B.E., Otten M.L., et al. Controversies in the endovascular management of cerebral vasospasm after intracranial aneurysm rupture and future directions for therapeutic approaches. Neurosurgery. 2008;62:897-905. discussion 905-907
Kondziolka D., Mclaughlin M.R., Kestle J.R. Simple risk predictions for arteriovenous malformation hemorrhage. Neurosurgery. 1995;37:851-855.
Krings T., Geibprasert S. Spinal dural arteriovenous fistulas. AJNR Am J Neuroradiol. 2009;30:639-648.
Kuroiwa T., Tanaka H., Ohta T., et al. Preoperative embolization of highly vascular brain tumors: clinical and histopathological findings. Noshuyo Byori. 1996;13:27-36.
Kurre W., Berkefeld J. Materials and techniques for coiling of cerebral aneurysms: how much scientific evidence do we have? Neuroradiology. 2008;50:909-927.
Kurre W., Berkefeld J., Sitzer M., et al. Treatment of symptomatic high-grade intracranial stenoses with the balloon-expandable Pharos stent: initial experience. Neuroradiology. 2008;50:701-708.
Kwan E., Hieshima G.B., Higashida R.T., et al. Interventional neuroradiology in neuro-ophthalmology. J Clin Neuroophthalmol. 1989;9:83-97.
Lefkowitz M.A., Gobin Y.P., Akiba Y., et al. Ballonn-assisted Guglielmi detachable coiling of wide-necked aneurysma: part II–clinical results. Neurosurgery. 1999;45:531-537. discussion 537-538
Levy E.I., Horowitz M.B., Koebbe C.J., et al. Transluminal stent-assisted angioplasty of the intracranial vertebrobasilar system for medically refractory, posterior circulation ischemia: early results. Neurosurgery. 2001;48:1215-1221. discussion 1221-1223
Levy E.I., Siddiqui A.H., Crumlish A., et al. First Food and Drug Administration-approved prospective trial of primary intracranial stenting for acute stroke: SARIS (Stent-Assisted Recanalization in Acute Ischemic Stroke). Stroke. 2009;40:3552-3556.
Lewandowski C.A., Frankel M., Tomsick T.A., et al. Combined intravenous and intra-arterial r-TPA versus intra-arterial therapy of acute ischemic stroke: Emergency Management of Stroke (EMS) Bridging Trial. Stroke. 1999;30:2598-2605.
Lieber B.B., Wakhloo A.K., Siekmann R., et al. Acute and chronic swine rete arteriovenous malformation models: effect of Ethiodol and glacial acetic acid on penetration, dispersion, and injection force of N-butyl 2-cyanoacrylate. AJNR Am J Neuroradiol. 2005;26:1707-1714.
Liebeskind D.S. Reperfusion for acute ischemic stroke: arterial revascularization and collateral therapeutics. Curr Opin Neurol. 2010;23:36-45.
Linfante I., Akkawi N.M., Perlow A., et al. Polyglycolide/polylactide-coated platinum coils for patients with ruptured and unruptured cerebral aneurysms: a single-center experience. Stroke. 2005;36:1948-1953.
Liu H.M., Huang Y.C., Wang Y.H. Embolization of cerebral arteriovenous malformations with n-butyl-2-cyanoacrylate. J Formos Med Assoc. 2000;99:906-913.
Lylyk P., Ferrario A., Pasbon B., et al. Buenos Aires experience with the Neuroform self-expanding stent for the treatment of intracranial aneurysms. J Neurosurg. 2005;102:235-241.
Lylyk P., Miranda C., Ceratto R., et al. Curative endovascular reconstruction of cerebral aneurysms with the pipeline embolization device: the Buenos Aires experience. Neurosurgery. 2009;64:632-642. discussion 642-643; quiz N6
Macdonald R.L., Pluta R.M., Zhang J.H. Cerebral vasospasm after subarachnoid hemorrhage: the emerging revolution. Nat Clin Pract Neurol. 2007;3:256-263.
Macdonald R.L., Wallace M.C., Montanera W.J., et al. Pathological effects of angioplasty on vasospastic carotid arteries in a rabbit model. J Neurosurg. 1995;83:111-117.
Macpherson P. The value of pre-operative embolisation of meningioma estimated subjectively and objectively. Neuroradiology. 1991;33:334-337.
Malek A.M., Halbach V.V., Phatouros C.C., et al. Balloon-assist technique for endovascular coil embolization of geometrically difficult intracranial aneurysms. Neurosurgery. 2000;46:1397-1406. discussion 1406-1407
Manelfe C., Lasjaunias P., Ruscalleda J. Preoperative embolization of intracranial meningiomas. AJNR Am J Neuroradiol. 1986;7:963-972.
Marangos N., Schumacher M. Facial palsy after glomus jugulare tumour embolization. J Laryngol Otol. 1999;113:268-270.
Marks M.P., Wojak J.C., Al-Ali F., et al. Angioplasty for symptomatic intracranial stenosis: clinical outcome. Stroke. 2006;37:1016-1020.
Mas J.L., Chatellier G., Beyssen B., et al. Endarterectomy versus stenting in patients with symptomatic severe carotid stenosis. N Engl J Med. 2006;355:1660-1671.
Mast H., Young W.L., Koennecke H.C., et al. Risk of spontaneous haemorrhage after diagnosis of cerebral arteriovenous malformation. Lancet. 1997;350:1065-1068.
Mathis J.A., Barr J.D., Horton J.A., et al. The efficacy of particulate embolization combined with stereotactic radiosurgery for treatment of large arteriovenous malformations of the brain. AJNR Am J Neuroradiol. 1995;16:299-306.
Mayberg M.R. Cerebral vasospasm. Neurosurg Clin N Am. 1998;9:615-627.
Mazighi M., Serfaty J.M., Labreuche J., et al. Comparison of intravenous alteplase with a combined intravenous-endovascular approach in patients with stroke and confirmed arterial occlusion (RECANALISE study): a prospective cohort study. Lancet Neurol. 2009;8:802-809.
McAuliffe W., Townsend M., Eskridge J.M., et al. Intracranial pressure changes induced during papaverine infusion for treatment of vasospasm. J Neurosurg. 1995;83:430-434.
McCutcheon I.E., Doppman J.L., Oldfield E.H. Microvascular anatomy of dural arteriovenous abnormalities of the spine: a microangiographic study. J Neurosurg. 1996;84:215-220.
Milburn J.M., Moran C.J., Cross D.T.3rd, et al. Increase in diameters of vasospastic intracranial arteries by intraarterial papaverine administration. J Neurosurg. 1998;88:38-42.
Molyneux A., Kerr R., Stratton I., et al. International Subarachnoid Aneurysm Trial (ISAT) of neurosurgical clipping versus endovascular coiling in 2143 patients with ruptured intracranial aneurysms: a randomised trial. Lancet. 2002;360:1267-1274.
Molyneux A.J., Cekirge S., Saatci I., et al. Cerebral Aneurysm Multicenter European Onyx (CAMEO) trial: results of a prospective observational study in 20 European centers. AJNR Am J Neuroradiol. 2004;25:39-51.
Molyneux A.J., Kerr R.S., Yu L.M., et al. International subarachnoid aneurysm trial (ISAT) of neurosurgical clipping versus endovascular coiling in 2143 patients with ruptured intracranial aneurysms: a randomised comparison of effects on survival, dependency, seizures, rebleeding, subgroups, and aneurysm occlusion. Lancet. 2005;366:809-817.
Moret J., Cognard C., Weill A., et al. Reconstruction technic in the treatment of wide-neck intracranial aneurysms. Long-term angiographic and clinical results. Apropos of 56 cases. J Neuroradiol. 1997;24:30-44.
Mori T., Fukuoka M., Kazita K., et al. Follow-up study after intracranial percutaneous transluminal cerebral balloon angioplasty. AJNR Am J Neuroradiol. 1998;19:1525-1533.
Moroi J., Hadeishi H., Suzuki A., et al. Morbidity and mortality from surgical treatment of unruptured cerebral aneurysms at Research Institute for Brain and Blood Vessels-Akita. Neurosurgery. 2005;56:224-231. discussion 224-231
Mounayer C., Hammami N., Piotin M., et al. Nidal embolization of brain arteriovenous malformations using Onyx in 94 patients. AJNR Am J Neuroradiol. 2007;28:518-523.
Muizelaar J.P., Zwienenberg M., Rudisill N.A., et al. The prophylactic use of transluminal balloon angioplasty in patients with Fisher Grade 3 subarachnoid hemorrhage: a pilot study. J Neurosurg. 1999;91:51-58.
Murayama Y., Tateshima S., Gonzalez N.R., et al. Matrix and bioabsorbable polymeric coils accelerate healing of intracranial aneurysms: long-term experimental study. Stroke. 2003;34:2031-2037.
Murayama Y., Vinuela F., Ulhoa A., et al. Nonadhesive liquid embolic agent for cerebral arteriovenous malformations: preliminary histopathological studies in swine rete mirabile. Neurosurgery. 1998;43:1164-1175.
n-BCA Trial Investigators. N-butyl cyanoacrylate embolization of cerebral arteriovenous malformations: results of a prospective, randomized, multi-center trial. AJNR Am J Neuroradiol. 2002;23:748-755.
Newell D.W., Eskridge J., Mayberg M., et al. Endovascular treatment of intracranial aneurysms and cerebral vasospasm. Clin Neurosurg. 1992;39:348-360.
Newton T.H., Cronqvist S. Involvement of dural arteries in intracranial arteriovenous malformations. Radiology. 1969;93:1071-1078.
Nogueira R.G., Schwamm L.H., Buonanno F.S., et al. Low-pressure balloon angioplasty with adjuvant pharmacological therapy in patients with acute ischemic stroke caused by intracranial arterial occlusions. Neuroradiology. 2008;50:331-340.
Nogueira R.G., Schwamm L.H., Hirsch J.A. Endovascular approaches to acute stroke, part 1: Drugs, devices, and data. AJNR Am J Neuroradiol. 2009;30:649-661.
Nogueira R.G., Yoo A.J., Buonanno F.S., et al. Endovascular approaches to acute stroke, part 2: a comprehensive review of studies and trials. AJNR Am J Neuroradiol. 2009;30:859-875.
Ogilvy C.S., Carter B.S. Stratification of outcome for surgically treated unruptured intracranial aneurysms. Neurosurgery. 2003;52:82-87. discussion 87-88
Ogilvy C.S., Hoh B.L., Singer R.J., et al. Clinical and radiographic outcome in the management of posterior circulation aneurysms by use of direct surgical or endovascular techniques. Neurosurgery. 2002;51:14-21. discussion 21-22
Ondra S.L., Troupp H., George E.D., et al. The natural history of symptomatic arteriovenous malformations of the brain: a 24-year follow-up assessment. J Neurosurg. 1990;73:387-391.
Ong A.T., Van Domburg R.T., Aoki J., et al. Sirolimus-eluting stents remain superior to bare-metal stents at two years: medium-term results from the Rapamycin-Eluting Stent Evaluated at Rotterdam Cardiology Hospital (RESEARCH) registry. J Am Coll Cardiol. 2006;47:1356-1360.
Persky M.S., Setton A., Niimi Y., et al. Combined endovascular and surgical treatment of head and neck paragangliomas–a team approach. Head Neck. 2002;24:423-431.
Piotin M., Iijima A., Wada H., et al. Increasing the packing of small aneurysms with complex-shaped coils: an in vitro study. AJNR Am J Neuroradiol. 2003;24:1446-1448.
Piotin M., Mandai S., Murphy K.J., et al. Dense packing of cerebral aneurysms: an in vitro study with detachable platinum coils. AJNR Am J Neuroradiol. 2000;21:757-760.
Piske R.L., Kanashiro L.H., Paschoal E., et al. Evaluation of Onyx HD-500 embolic system in the treatment of 84 wide-neck intracranial aneurysms. Neurosurgery. 2009;64:E865-875. discussion E875
Pollock B.E., Nichols D.A., Garrity J.A., et al. Stereotactic radiosurgery and particulate embolization for cavernous sinus dural arteriovenous fistulae. Neurosurgery. 1999;45:459-466. discussion 466-467
Pouratian N., Oskouian R.J.Jr., Jensen M.E., et al. Endovascular management of unruptured intracranial aneurysms. J Neurol Neurosurg Psychiatry. 2006;77:572-578.
Probst E.N., Grzyska U., Westphal M., et al. Preoperative embolization of intracranial meningiomas with a fibrin glue preparation. AJNR Am J Neuroradiol. 1999;20:1695-1702.
Purdy P.D., Batjer H.H., Samson D., et al. Intraarterial sodium Amytal administration to guide preoperative embolization of cerebral arteriovenous malformations. J Neurosurg Anesthesiol. 1991;3:103-106.
Qureshi A.I., Kirmani J.F., Hussein H.M., et al. Early and intermediate-term outcomes with drug-eluting stents in high-risk patients with symptomatic intracranial stenosis. Neurosurgery. 2006;59:1044-1051. discussion 1051
Raaymakers T.W., Rinkel G.J., Limburg M., et al. Mortality and morbidity of surgery for unruptured intracranial aneurysms: a meta-analysis. Stroke. 1998;29:1531-1538.
Raftopoulos C., Goffette P., Vaz G., et al. Surgical clipping may lead to better results than coil embolization: results from a series of 101 consecutive unruptured intracranial aneurysms. Neurosurgery. 2003;52:1280-1287. discussion 1287-1290
Raftopoulos C., Mathurin P., Boscherini D., et al. Prospective analysis of aneurysm treatment in a series of 103 consecutive patients when endovascular embolization is considered the first option. J Neurosurg. 2000;93:175-182.
Raja P.V., Huang J., Germanwala A.V., et al. Microsurgical clipping and endovascular coiling of intracranial aneurysms: a critical review of the literature. Neurosurgery. 2008;62:1187-1202. discussion 1202-1203
Rasmussen P.A. The evolution and role of endovascular therapy for the treatment of intracranial atherosclerotic disease. J Neuroimaging. 2009;19(Suppl 1):30S-34S.
Regar E., Serruys P.W., Bode C., et al. Angiographic findings of the multicenter randomized study with the sirolimus –eluting Bx velocity balloon-expandable stent (RAVEL): sirolimus eluting stents inhibit restenosis irrespective of the vessel size. Circulation. 2002;106:1949-1956.
Regli L., Dehdashti A.R., Uske A., et al. Endovascular coiling compared with surgical clipping for the treatment of unruptured middle cerebral artery aneurysms: an update. Acta Neurochir Suppl. 2002;82:41-46.
Regli L., Uske A., De Tribolet N. Endovascular coil placement compared with surgical clipping for the treatment of unruptured middle cerebral artery aneurysms: a consecutive series. J Neurosurg. 1999;90:1025-1030.
Richter H.P., Schachenmayr W. Preoperative embolization of intracranial meningiomas. Neurosurgery. 1983;13:261-268.
Ricotta J.J.2nd, Malgor R.D. A review of the trials comparing carotid endarterectomy and carotid angioplasty and stenting. Perspect Vasc Surg Endovasc Ther. 2008;20:299-308.
Ringer A.J., Qureshi A.I., Fessler R.D., et al. Angioplasty of intracranial occlusion resistant to thrombolysis in acute ischemic stroke. Neurosurgery. 2001;48:1282-1288. discussion 1288-1290
Ringleb P.A., Allenberg J., Bruckmann H., et al. 30 day results from the SPACE trial of stent-protected angioplasty versus carotid endarterectomy in symptomatic patients: a randomised non-inferiority trial. Lancet. 2006;368:1239-1247.
Roberson G.H., Price A.C., Davis J.M., et al. Therapeutic embolization of juvenile angiofibroma. AJR Am J Roentgenol. 1979;133:657-663.
Rosamond W., Flegal K., Furie K., et al. Heart disease and stroke statistics–2008 update: a report from the American Heart Association Statistics Committee and Stroke Statistics Subcommittee. Circulation. 2008;117:e25-e146.
Rosen C.L., Ammerman J.M., Sekhar L.N., et al. Outcome analysis of preoperative embolization in cranial base surgery. Acta Neurochir (Wien). 2002;144:1157-1164.
Rosenwasser R.H., Armonda R.A., Thomas J.E., et al. Therapeutic modalities for the management of cerebral vasospasm: timing of endovascular options. Neurosurgery. 1999;44:975-979. discussion 979-980
Sacco R.L., Adams R., Albers G., et al. Guidelines for prevention of stroke in patients with ischemic stroke or transient ischemic attack: a statement for healthcare professionals from the American Heart Association/American Stroke Association Council on Stroke: co-sponsored by the Council on Cardiovascular Radiology and Intervention: the American Academy of Neurology affirms the value of this guideline. Circulation. 2006;113:e409-e449.
Sacco R.L., Kargman D.E., Gu Q., et al. Race-ethnicity and determinants of intracranial atherosclerotic cerebral infarction. The Northern Manhattan Stroke Study. Stroke. 1995;26:14-20.
Savage M.P., Fischman D.L., Rake R., et al. Efficacy of coronary stenting versus balloon angioplasty in small coronary arteries. Stent Restenosis Study (STRESS) Investigators. J Am Coll Cardiol. 1998;31:307-311.
Schellinger P.D., Fiebach J.B., Mohr A., et al. Thrombolytic therapy for ischemic stroke–a review. Part I–Intravenous thrombolysis. Crit Care Med. 2001;29:1812-1818.
Schievink W.I. Intracranial aneurysms. N Engl J Med. 1997;336:28-40.
Scholtz A.W., Appenroth E., Kammen-Jolly K., et al. Juvenile nasopharyngeal angiofibroma: management and therapy. Laryngoscope. 2001;111:681-687.
Schumacher M., Horton J.A. Treatment of cerebral arteriovenous malformations with PVA. Results and analysis of complications. Neuroradiology. 1991;33:101-105.
Scroop R., Britz G.W., West G.A., et al. Endovascular therapy for vasospasm associated with subarachnoid hemorrhage. In: Winn H.R., Le Roux P. Management of Cerebral Aneurysms. Philadelphia: Saunders; 2004:489-498.
Sekhon L.H., Morgan M.K., Spence I., et al. Chronic cerebral hypoperfusion: pathological and behavioral consequences. Neurosurgery. 1997;40:548-556.
Serruys P.W., De Jaegere P., Kiemeneij F., et al. A comparison of balloon-expandable-stent implantation with balloon angioplasty in patients with coronary artery disease. Benestent Study Group. N Engl J Med. 1994;331:489-495.
Shi Z.S., Feng L., Jiang X.B., et al. Therapeutic embolization of meningiomas with Onyx for delayed surgical resection. Surg Neurol. 2008;70:478-481.
Siddiq F., Memon M.Z., Vazquez G., et al. Comparison between primary angioplasty and stent placement for symptomatic intracranial atherosclerotic disease: meta-analysis of case series. Neurosurgery. 2009;65:1024-1033. discussion 1033-1034
Siddiq F., Vazquez G., Memon M.Z., et al. Comparison of primary angioplasty with stent placement for treating symptomatic intracranial atherosclerotic diseases: a multicenter study. Stroke. 2008;39:2505-2510.
Sivakumar W., Zada G., Yashar P., et al. Endovascular management of spinal dural arteriovenous fistulas. A review. Neurosurg Focus. 2009;26:E15.
Smith W.S., Dowd C.F., Johnston S.C., et al. Neurotoxicity of intra-arterial papaverine preserved with chlorobutanol used for the treatment of cerebral vasospasm after aneurysmal subarachnoid hemorrhage. Stroke. 2004;35:2518-2522.
Smith W.S., Sung G., Saver J., et al. Mechanical thrombectomy for acute ischemic stroke: final results of the Multi MERCI trial. Stroke. 2008;39:1205-1212.
Smith W.S., Sung G., Starkman S., et al. Safety and efficacy of mechanical embolectomy in acute ischemic stroke: results of the MERCI trial. Stroke. 2005;36:1432-1438.
Soderman M., Pavic L., Edner G., et al. Natural history of dural arteriovenous shunts. Stroke. 2008;39:1735-1739.
Sorimachi T., Koike T., Takeuchi S., et al. Embolization of cerebral arteriovenous malformations achieved with polyvinyl alcohol particles: angiographic reappearance and complications. AJNR Am J Neuroradiol. 1999;20:1323-1328.
Spetzler R.F., Detwiler P.W., Riina H.A., et al. Modified classification of spinal cord vascular lesions. J Neurosurg. 2002;96:145-156.
Spetzler R.F., Martin N.A., Carter L.P., et al. Surgical management of large AVMs by staged embolization and operative excision. J Neurosurg. 1987;67:17-28.
SSYLVIA Study Investigators. Stenting of Symptomatic Atherosclerotic Lesions in the Vertebral or Intracranial Arteries (SSYLVIA): study results. Stroke. 2004;35:1388-1392.
Stapf C., Labovitz D.L., Sciacca R.R., et al. Incidence of adult brain arteriovenous malformation hemorrhage in a prospective population-based stroke survey. Cerebrovasc Dis. 2002;13:43-46.
Stapf C., Mast H., Sciacca R.R., et al. Predictors of hemorrhage in patients with untreated brain arteriovenous malformation. Neurology. 2006;66:1350-1355.
Stapf C., Mohr J.P., Choi J.H., et al. Invasive treatment of unruptured brain arteriovenous malformations is experimental therapy. Curr Opin Neurol. 2006;19:63-68.
Stapf C., Mohr J.P., Pile-Spellman J., et al. Epidemiology and natural history of arteriovenous malformations. Neurosurg Focus. 2001;11:e1.
Stingele R., Berger J., Alfke K., et al. Clinical and angiographic risk factors for stroke and death within 30 days after carotid endarterectomy and stent-protected angioplasty: a subanalysis of the SPACE study. Lancet Neurol. 2008;7:216-222.
Stone G.W., Ellis S.G., Cox D.A., et al. A polymer-based, paclitaxel-eluting stent in patients with coronary artery disease. N Engl J Med. 2004;350:221-231.
Strom R.G., Botros J.A., Refai D., et al. Cranial dural arteriovenous fistulae: asymptomatic cortical venous drainage portends less aggressive clinical course. Neurosurgery. 2009;64:241-247. discussion 247-248
Sussman B.J., Fitch T.S. Thrombolysis with fibrinolysin in cerebral arterial occlusion. J Am Med Assoc. 1958;167:1705-1709.
Taha M.M., Nakahara I., Higashi T., et al. Endovascular embolization vs surgical clipping in treatment of cerebral aneurysms: morbidity and mortality with short-term outcome. Surg Neurol. 2006;66:277-284. discussion 284
Taki W., Yonekawa Y., Iwata H., et al. A new liquid material for embolization of arteriovenous malformations. AJNR Am J Neuroradiol. 1990;11:163-168.
Terada T., Higashida R.T., Halbach V.V., et al. Transluminal angioplasty for arteriosclerotic disease of the distal vertebral and basilar arteries. J Neurol Neurosurg Psychiatry. 1996;60:377-381.
The National Institute of Neurological Disorders and Stroke rt-PA Stroke Study Group. Tissue plasminogen activator for acute ischemic stroke. The National Institute of Neurological Disorders and Stroke rt-PA Stroke Study Group. N Engl J Med. 1995;333:1581-1587.
Tietke M., Jansen O. Cerebral protection vs no cerebral protection: timing of stroke with CAS. J Cardiovasc Surg (Torino). 2009;50:751-760.
Tikkakoski T., Luotonen J., Leinonen S., et al. Preoperative embolization in the management of neck paragangliomas. Laryngoscope. 1997;107:821-826.
Turan T.N., Maidan L., Cotsonis G., et al. Failure of antithrombotic therapy and risk of stroke in patients with symptomatic intracranial stenosis. Stroke. 2009;40:505-509.
Turjman F., Massoud T.F., Vinuela F., et al. Correlation of the angioarchitectural features of cerebral arteriovenous malformations with clinical presentation of hemorrhage. Neurosurgery. 1995;37:856-860. discussion 860-862
Uflacker R., Schonholz C., Papamitisakis N. Interim report of the SENTIS trial: cerebral perfusion augmentation via partial aortic occlusion in acute ischemic stroke. J Cardiovasc Surg (Torino). 2008;49:715-721.
Van Dijk J.M., Terbrugge K.G., Willinsky R.A., et al. Clinical course of cranial dural arteriovenous fistulas with long-term persistent cortical venous reflux. Stroke. 2002;33:1233-1236.
Van Dijk J.M., Terbrugge K.G., Willinsky R.A., et al. Selective disconnection of cortical venous reflux as treatment for cranial dural arteriovenous fistulas. J Neurosurg. 2004;101:31-35.
Van Rooij W.J., Sluzewski M., Beute G.N. Brain AVM embolization with Onyx. AJNR Am J Neuroradiol. 2007;28:172-177. discussion 178
Velat G.J., Reavey-Cantwell J.F., Sistrom C., et al. Comparison of N-butyl cyanoacrylate and onyx for the embolization of intracranial arteriovenous malformations: analysis of fluoroscopy and procedure times. Neurosurgery. 2008;63:ONS73-78. discussion ONS78-80
Vinuela F., Fox A.J., Pelz D., et al. Angiographic follow-up of large cerebral AVMs incompletely embolized with isobutyl-2-cyanoacrylate. AJNR Am J Neuroradiol. 1986;7:919-925.
Wakhloo A.K., Gounis M.J., Sandhu J.S., et al. Complex-shaped platinum coils for brain aneurysms: higher packing density, improved biomechanical stability, and midterm angiographic outcome. AJNR Am J Neuroradiol. 2007;28:1395-1400.
Wakhloo A.K., Juengling F.D., Van Velthoven V., et al. Extended preoperative polyvinyl alcohol microembolization of intracranial meningiomas: assessment of two embolization techniques. AJNR Am J Neuroradiol. 1993;14:571-582.
Wakhloo A.K., Sandhu J.S., Linfante I. Principles in endovascular therapy. In: Bradley W.G., editor. Neurology in Clinical Practice. fifth ed. Philadelphia: Butterworth-Heinemann; 2008:965-992.
Wiebers D.O., Whisnant J.P., Huston J.3rd, et al. Unruptured intracranial aneurysms: natural history, clinical outcome, and risks of surgical and endovascular treatment. Lancet. 2003;362:103-110.
Wikholm G., Lundqvist C., Svendsen P. The Goteborg cohort of embolized cerebral arteriovenous malformations: a 6-year follow-up. Neurosurgery. 2001;49:799-805. discussion 805-806
Williams M., Patil S., Toledo E.G., et al. Management of acute ischemic stroke: current status of pharmacological and mechanical endovascular methods. Neurol Res. 2009;31:807-815.
Wojak J.C., Dunlap D.C., Hargrave K.R., et al. Intracranial angioplasty and stenting: long-term results from a single center. AJNR Am J Neuroradiol. 2006;27:1882-1892.
Yadav J.S. Carotid stenting in high-risk patients: design and rationale of the SAPPHIRE trial. Cleve Clin J Med. 2004;71(Suppl 1):S45-S46.
Yadav J.S., Roubin G.S., Iyer S., et al. Elective stenting of the extracranial carotid arteries. Circulation. 1997;95:376-381.
Yamamoto Y., Smith R.R., Bernanke D.H. Mechanism of action of balloon angioplasty in cerebral vasospasm. Neurosurgery. 1992;30:1-5. discussion 5-6
Yoneyama T., Nakano S., Kawano H., et al. Combined direct percutaneous transluminal angioplasty and low-dose native tissue plasminogen activator therapy for acute embolic middle cerebral artery trunk occlusion. AJNR Am J Neuroradiol. 2002;23:277-281.
Yoon Y.S., Ahn J.Y., Chang J.H., et al. Pre-operative embolisation of internal carotid artery branches and pial vessels in hypervascular brain tumours. Acta Neurochir (Wien). 2008;150:447-452. discussion 452
Yu S.C., Chan M.S., Lam J.M., et al. Complete obliteration of intracranial arteriovenous malformation with endovascular cyanoacrylate embolization: initial success and rate of permanent cure. AJNR Am J Neuroradiol. 2004;25:1139-1143.
Yu W., Smith W.S., Singh V., et al. Long-term outcome of endovascular stenting for symptomatic basilar artery stenosis. Neurology. 2005;64:1055-1057.
Zaidat O.O., Klucznik R., Alexander M.J., et al. The NIH registry on use of the Wingspan stent for symptomatic 70-99% intracranial arterial stenosis. Neurology. 2008;70:1518-1524.
Zipfel G.J., Shah M.N., Refai D., et al. Cranial dural arteriovenous fistulas: modification of angiographic classification scales based on new natural history data. Neurosurg Focus. 2009;26:E14.
Zubkov Y.N., Nikiforov B.M., Shustin V.A. Balloon catheter technique for dilatation of constricted cerebral arteries after aneurysmal SAH. Acta Neurochir (Wien). 1984;70:65-79.
Zwienenberg-Lee M., Hartman J., Rudisill N., et al. Effect of prophylactic transluminal balloon angioplasty on cerebral vasospasm and outcome in patients with Fisher grade III subarachnoid hemorrhage: results of a phase II multicenter, randomized, clinical trial. Stroke. 2008;39:1759-1765.