Chapter 41 Prevention and Treatment of Knee Arthrofibrosis
INTRODUCTION
Loss of a normal range of knee motion after an injury or ligament reconstruction is a potentially devastating complication. Although a multitude of investigations and publications have appeared since the early 1990s regarding this problem, loss of knee motion continues to be one of the most frequently reported complications after surgical procedures.27,31,72,83,84 Although the definition and use of the term arthrofibrosis vary among authors, it implies a loss of knee flexion, extension, or both compared with the contralateral normal knee.
Primary arthrofibrosis is caused by an exaggerated inflammatory response to an injury or surgical procedure, followed by the production of fibroblastic cells and an increase in the deposition of extracellular matrix proteins.147 Proliferative scar tissue or fibrous adhesions form within the joint, which can result in either localized or diffuse involvement of all of the compartments of the knee and the extra-articular soft tissues (Fig. 41-1). In the most severe cases, dense scar tissue obliterates the normal peripatellar recesses, suprapatellar pouch, intercondylar notch, and articular surfaces. Formation of dense scar tissue in the infrapatellar region may result in a patella infera and permanent limitation of normal knee flexion and extension. The consequent pain and restricted knee motion resulting from arthrofibrosis may lead to disabling events including severe quadriceps atrophy, loss of patellar mobility, patellar tendon adaptive shortening, patella infera, and articular cartilage deterioration.105,106
The incidence of arthrofibrosis after ligament reconstructive surgery varies according to several factors, which are discussed. Two of the most commonly noted factors are knee dislocations and major concurrent operative procedures performed with the ligament surgery such as reconstruction of other knee ligaments, complex meniscus repair, or high tibial osteotomy (Table 41-1).7,91–103 Publications show wide disparity among authors regarding the treatment of this problem, especially the time postoperatively that intervention is warranted and what type of treatment should be implemented for losses of knee extension and flexion and loss of patella mobility.
It is important to note that limitations in knee flexion and extension may occur owing to reasons other than arthrofibrosis. Any acute injury causing pain and swelling may cause a short-term loss of knee motion, which usually resolves as symptoms subside. Other factors influencing the restoration of knee motion include a mechanical block from a displaced bucket-handle meniscus tear, an impinged or improperly placed anterior cruciate ligament (ACL) graft, a cyclops lesion,65 improper graft tensioning that constrains normal knee joint motion,20 and graft fixation at 30° or more of extension.5 In addition, contracture of the posterior capsular structures may limit knee extension. If not resolved, these problems may result in the development of what is termed secondary arthrofibrosis, complicating the course of treatment.
Normal knee motion varies, but most individuals have some amount of hyperextension, which averages 5° in men and 6° in women.30,31,74 This degree of hyperextension is required so that the normal “screw-home” mechanism can occur that allows the knee to be stabilized in extension during the stance phase, with the quadriceps muscle relaxed. Normal knee flexion averages 140° in men and 143° in women. It is generally assumed that at least 125° of knee flexion is required for activities of daily living.31,74 Rowe and coworkers120 measured knee kinematics during functional activities in a group of 20 subjects aged 49 to 80 years and reported that 90° of flexion is required for gait and slopes, 90° to 120° for stairs and sitting, and 135° for bathing. Patients not involved in athletics tolerate small flexion deficits. Significant flexion deficits of less than 90° cause problems with squatting, stair-climbing, and sitting. Athletes have a poorer tolerance for even minor losses of flexion, which affect running and jumping. Cosgarea and associates24 reported that deficits of 10° or more of flexion were associated with decreased running speed.
All patients, regardless of their activity level, have greater problems with loss of knee extension. A loss of just 5° of extension may produce a flexed-knee gait, fatigue the quadriceps muscle, and cause patellofemoral pain.63,66,86,89,121 Limitation of more than 20° may cause a functional limb-length discrepancy.24 Many years ago, Perry and colleagues114 demonstrated the effect of a loss of extension on joint contact pressures, quadriceps muscle activity, and fatigue. These investigators measured the extensor forces required to stabilize the flexed knee during simulated weight-bearing in cadaver specimens. The quadriceps force required to stabilize the knee was 75% of the load on the head of the femur at 15° of flexion, which increased to 210% at 30° of flexion, and to 410% at 60° of flexion. The quadriceps force was equal to 20% of the average maximum quadriceps strength at 15° of flexion, but increased to 50% at 30° of flexion. Surface pressure in the tibiofemoral and patellofemoral joints also increased with greater amounts of knee flexion.
TERMINOLOGY AND CLASSIFICATION SYSTEMS
A variety of terms have been used to describe, define, or classify a limitation of knee flexion and extension including arthrofibrosis, flexion contracture, ankylosis, infrapatellar contracture syndrome, and motion loss. Some of the terms indicate a specific process whereas others simply imply a limitation of motion compared with the contralateral normal knee. Ankylosis is one such generic term that indicates stiffness of joints that occurs for any reason and may represent loss of knee flexion, extension, or both. The term motion loss is another general phrase used to describe deviations from the amount of flexion and extension compared with the contralateral normal knee. Conversely, arthrofibrosis describes a specific cause for a limitation of knee motion, which is the formation of diffuse scar tissue or fibrous adhesions within a joint.63,111,115 Flexion contracture indicates a loss of extension due to any cause and is accompanied by a relative shortening of the posterior soft tissues (in either the capsule or the muscles). Petsche and Hutchinson115 stated that arthrofibrosis and flexion contracture are general terms, and it is necessary to indicate a specific cause for loss of motion.
Critical Points TERMINOLOGY AND CLASSIFICATION SYSTEMS
Classification systems have been proposed by various authors to describe limitations in knee motion, based on either the anatomic location of scar tissue and adhesions or the amount of knee motion in the affected joint (Table 41-2).12,33,111,132,141 Sprague and coworkers141 first introduced a system based on the arthroscopically observed location of scar tissues and adhesions in 24 patients who had a severe limitation of knee motion. Paulos and associates111 described three stages of knee motion restrictions due to infrapatellar contracture syndrome. Del Pizzo and colleagues33 and Shelbourne and coworkers132 developed classification systems based on the deviation of knee motion compared to the opposite knee. Blauth and Jaeger12 described a system based on the total arc of motion in the affected knee.
Reference | Classification System |
---|---|
Sprague et al., 1982141 |
The authors developed a classification system based on the anatomic sites at which scar tissue, adhesions, and adaptive shortening of soft tissues occur (Fig. 41-2 and Table 41-3). This system is advantageous because it highlights the areas that must be addressed surgically, as is discussed.
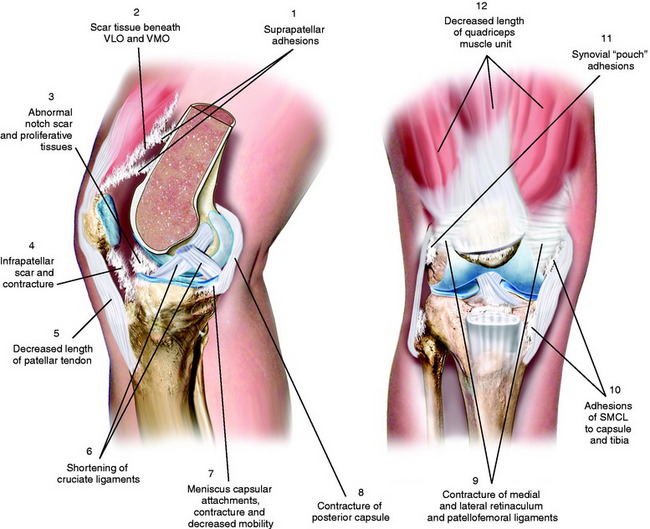
FIGURE 41-2 Multiple areas of soft tissue contracture, adhesions, and scar tissue formation with knee arthrofibrosis.
TABLE 41-3 Authors’ Anatomic Classification System of Arthrofibrosis
Loss of Flexion |
Quadriceps muscle |
Suprapatellar pouch |
Medial and lateral capsular pouches |
Patellar retinaculum and associated ligaments |
Patellar tendon |
Medial and lateral extra-articular ligament structures |
Loss of Extension |
Posterior capsule |
Femoral notch |
Hamstrings muscle |
Cruciate ligaments |
ACL, anterior cruciate ligament; PCL, posterior cruciate ligament; VLO, vastus lateralis obliquus; VMO, vastus medialis obliquus.
RISK FACTORS AFTER KNEE LIGAMENT RECONSTRUCTION
A variety of factors appear to influence the requirement for treatment intervention for knee motion limitations (Table 41-4) and the final amount of knee flexion and extension gained after ligament reconstruction. These factors are related to the severity of the injury, the timing of surgery, preoperative treatment, technical aspects of the ligament reconstruction, and the postoperative course and rehabilitation. Although it remains uncertain why some knees are more likely to form an abnormal scar response to trauma and surgery than others, knowledge of these factors may help reduce this problem.
TABLE 41-4 Risk Factors for the Development of Knee Arthrofibrosis
ACL, anterior cruciate ligament; MCL, medial collateral ligament.
Severity of the Injury
Patients who sustain knee dislocations are at increased risk for developing motion complications (Fig. 41-3).23,35,83,97,127,136 These injuries frequently occur from high-energy accidents that produce extreme soft tissue swelling and edema, hematomas, muscle damage, and other multiple trauma that must be resolved before consideration of knee soft tissue reconstructions. Early operative intervention when possible is advisable for multiple ligament injuries that involve the lateral and posterolateral structures where acute repair and augmentation procedures are performed (see Chapter 22, Posterolateral Ligament Injuries: Diagnosis, Operative Techniques, and Clinical Outcomes). For all other dislocations, surgery is delayed with the limb immobilized in a posterior plaster splint or bivalved cast with a posterior pad to prevent posterior tibial subluxation. Even in these severe knee injuries, it is possible to start immediate range of motion and prevent scar tissue formation that may compromise the outcome of a subsequent ligament reconstruction. Too often, these serious knee joint injuries are not treated with an early motion and function program. When surgical treatment is elected, the reconstructive and repair procedures of torn ligaments, capsular structures, and menisci are performed in a manner that allows immediate knee motion to be instituted postoperatively.97
Preoperative Issues
Performing ACL and other knee ligament reconstruction within a few weeks of the injury or before the resolution of swelling, pain, quadriceps muscle atrophy, abnormal gait mechanics, and motion limitations has been noted by many authors to correlate with postoperative knee motion problems.25,54,86,129,130,134,144,152 Shelbourne and associates134 noted an increased rate of arthrofibrosis in patients who underwent acute ACL reconstruction (within 1 week of the injury) compared with those in whom the reconstruction was delayed for at least 21 days. Similar findings were reported by Wasilewski and colleagues152 who noted that arthrofibrosis was found in 22% of acutely reconstructed knees versus 12.5% of knees reconstructed with chronic ACL deficiency. Mohtadi and coworkers86 found that ACL reconstructions performed less than 6 weeks postinjury had a higher rate of knee stiffness than those done more than 6 weeks postinjury (11% and 4%, respectively). Harner and associates54 reported a 37% rate of motion complications after acute ACL reconstructions (done within 1 month of the injury) compared with a 5% rate in chronic ACL-deficient knees.
Critical Points RISK FACTORS AFTER KNEE LIGAMENT RECONSTRUCTION
ACL, anterior cruciate ligament; MCL, medial collateral ligament.
Although Bach and colleagues6 did not observe a significant difference in knee motion complications between acute and chronic ACL-reconstructed knees, the authors stressed the need to delay surgery until patients achieved 120° of knee motion and swelling had resolved. The authors found “no advantage to performing urgent acute ACL reconstruction.”6 Sterett and coworkers143 found no association between surgical timing and postoperative motion problems. However, in their series, all patients had achieved at least 0° to 120°, had good quadriceps control, and could perform a straight leg raise without an extensor lag before undergoing surgery. Eight percent of the patients in this investigation’s acute subgroup required arthroscopic débridement of scar tissue. Meighan and associates81 also found no advantage in performing early ACL reconstruction and noted an increased rate of complications in patients who underwent surgery within 2 weeks of the injury compared with those who had surgery between 8 and 12 weeks postinjury. The delayed group had a faster return of knee motion and quadriceps strength.
A few authors have not found a higher rate of postoperative motion problems after acute ACL reconstruction.18,62,75 However, it appears that delaying surgery until knee motion is regained, swelling is resolved, and a good quadriceps contraction is demonstrated is advantageous in decreasing the risk of postoperative arthrofibrosis. The inflammatory response to the initial injury varies among patients. Whereas some have little effusion and swelling, others have an exaggerated inflammatory response characterized by pain, soft tissue edema, and redness and increased warmth to tissues surrounding the knee. These knees are placed into an initial conservative treatment program to resolve these problems first and are also carefully monitored after ACL reconstruction for a similar exaggerated inflammatory reaction postoperatively. Knees with isolated ACL ruptures that have little swelling and demonstrate normal motion early after injury may be considered for earlier reconstruction. In addition, knees with multiple ligament ruptures that include the posterolateral structures are also candidates for early surgical repair, as discussed in Chapter 22, Posterolateral Ligament Structures: Diagnosis, Operative Techniques, and Clinical Outcomes.
Technical Factors at Surgery
Improper ACL graft placement has been frequently noted as a cause of loss of knee motion.* On the tibia, grafts placed anterior to the native ACL insertion result in impingement on the roof of the intercondylar notch when the knee is extended.59,60,78 Yaru and colleagues154 recommended that with passive extension, there should be a 3-mm clearance between the anterior portion of the intercondylar notch and the graft to prevent impingement. Grafts placed lateral to the insertion site impinge on the lateral wall of the notch. In addition, Romano and coworkers119 found that ACL grafts placed too far anterior in the tibial tunnel can cause knee extension deficits, and grafts placed too far medially in the tibial tunnel can cause knee flexion deficits. On the femur, an excessive anterior graft placement causes deleterious forces on the graft, leading to limitations in flexion and potential graft failure (Fig. 41-4).
Overtensioning ACL grafts may lead to abnormal knee kinematics.84,115 In addition, the degree of knee extension during graft tensioning and fixation may affect postoperative motion.5,20,47,82,89 Austin and associates5 noted in a cadaver study that the amount of graft tension (44 N or 89 N) did not effect knee extension; however, tensioning the graft in knee flexion was associated with extension deficits. The authors reported that grafts tensioned and fixed at 30° of flexion had more than a 12° increase in knee flexion after ACL reconstruction compared with those tensioned and fixed at 0° of flexion. From a two-part biomechanical and clinical study, Nabors and colleagues89 suggested that grafts tensioned in full extension result in a low incidence of knee motion loss. In their series of 57 patients who underwent patellar tendon autograft ACL reconstruction, only 1 patient had a mild (5°) loss of extension.
Concurrent medial collateral ligament (MCL) repair with ACL reconstruction has been associated with an increased risk of knee arthrofibrosis.54,98,128 Harner and associates54 postulated that concurrent MCL primary repair may cause a limitation of knee motion due to the disruption of the medial capsule, because the procedure does not restore the normal tissue planes, resulting in scar formation and a heightened pain response. The studies of Shelbourne and coworkers128,133 and the authors of this chapter98 led to the recommendation many years ago to treat the majority of combined ACL-MCL ruptures conservatively. This allows healing of the medial-side injury, followed by ACL reconstruction in appropriately indicated patients (see Chapter 24, Medial and Posteromedial Ligament Injuries: Diagnosis, Operative Techniques, and Clinical Outcomes). There are exceptions to this rule, and any surgical procedure on the medial side of the knee joint should be carefully observed postoperatively for a limitation of motion or, in acute knee injuries, the development of heterotopic soft tissue ossificiation requiring treatment.126,150
Postoperative Course and Rehabilitation
There is consensus in the literature that immobilization is detrimental to all of the knee joint structures and may result in a permanent limitation of knee motion, prolonged muscle atrophy, patella infera, and articular cartilage deterioration.25,51,67,104–106,112,122 Early knee joint motion decreases pain and postoperative joint effusions, aids in the prevention of scar tissue formation and capsular contractions that can limit normal knee flexion and extension, decreases muscle disuse effects, maintains articular cartilage nutrition, and benefits the healing ACL graft.† Modern rehabilitation programs (see Chapters 12, Scientific Basis of Rehabilitation after Anterior Cruciate Ligament Autogenous Reconstruction; 13, Rehabilitation of Primary and Revision Anterior Cruciate Ligament Reconstructions; and 14, Neuromuscular Retraining after Anterior Cruciate Ligament Reconstruction) incorporate immediate knee motion and muscle-strengthening exercises the day after surgery, both of which have been shown to be safe and not deleterious to healing grafts. Importantly, the immediate motion program must include patellar mobilization (inferior, superior, medial, and lateral directions) to avoid an infrapatellar contracture.
Patient compliance with postoperative rehabilitation is essential in recovering full knee motion. In the authors’ experience, the small percentage of patients who have permanent restrictions in extension or flexion have often been unwilling to perform the required motion, strengthening, and patellar mobilization exercises postoperatively. In addition, in instances in which an early postoperative limitation of motion has been recognized, these patients are also unwilling to undergo treatment recommendations such as overpressure exercises, extension casts, and other modalities that are usually effective in resolving these problems.101,102 Thus, in a majority of cases, the inflammatory and fibrotic response that follows surgery and initially limits knee motion is treatable if no delay occurs in instituting a gentle motion and overpressure program along with appropriate anti-inflammatory medications. There is a distinct group of patients, probably in the range of 1% to 2%, who demonstrate a pathologic exaggerated fibrous tissue proliferative response from a genetic basis, in which the treatment is prolonged and may not be successful. This unique group of patients are discussed in a later section of this chapter.
Significant lower extremity muscle atrophy represents an unresolved problem after ACL reconstruction, because the magnitude of quadriceps atrophy and strength loss may exceed 20% to 30% in the first few months postoperatively.39,40,50,81,117 Prolonged quadriceps atrophy may affect the ability to regain normal knee motion and maintain knee extension. Chronic swelling may also cause a limitation of knee motion by inhibiting the function of the quadriceps muscle.29,110,140,145,146 It is important that a knee joint effusion be treated to lessen its deleterious effect on quadriceps function.
Infection may result in loss of knee motion after ACL reconstruction. The rule followed in the authors’ clinical practice is to always consider first that an exaggerated inflammatory response with joint swelling, synovitis, and early limitation of joint motion is caused by a joint infection until proved otherwise. Even when an infectious process appears to have been excluded, a knee joint that does not respond to the gentle modalities to regain knee motion, or that has continued pain or lack of patellar mobility, should undergo repeat aspiration, cell count, culture, and diagnostic studies. In the authors’ experience, the most severe cases of arthrofibrosis that initially are presumed to be on a genetic basis are subsequently proved to have an occult unrecognized infectious etiology. In patients with an established infectious process, the principles of treatment have been discussed (see Chapter 7, Anterior Cruciate Ligament Primary and Revision Reconstruction: Diagnosis, Operative Techniques, and Clinical Outcomes), and it is important that gentle motion and overpressure programs continue to maintain the joint motion. Even in knees with swelling of soft tissues and the initial host response to an infection, it is possible within days after instituting arthroscopic lavage, débridement, and appropriate antibiotics to initiate motion exercises and prevent a flexion contracture or patella infera. Although aggressive management of this complication usually leads to resolution of the infection, studies report permanent loss of extension and flexion in the majority of patients treated, which may be avoidable.80,125,148,153
Complex regional pain syndromes and reflex sympathetic dystrophy may cause knee motion problems as a result of quadriceps atrophy, chronic swelling, and an increased sensitivity to pain.49,107 These issues are discussed in detail in Chapter 43, Diagnosis and Treatment of Complex Regional Pain Syndrome. Appropriate management using a variety of medical specialists is essential to diagnose and treat these problems.
PATHOPHYSIOLOGY
In 1972, Enneking and Horowitz36 published one of the first studies on the pathophysiology of a joint contracture after immobilization in humans. The investigators described in a series of case reports the presence of fibrofatty connective tissue in the infrapatellar fat pad, suprapatellar pouch, and posterior recesses of the knee joint with eventual obliteration of the joint cavity. Over time, this tissue was replaced with mature fibrous connective tissue.
Tissue homeostasis is maintained by the normal level of cell growth and proliferation along with the production and turnover of the extracellular matrix.13,15 Polypeptides known as cytokines or growth factors are responsible for the constant signaling that occurs both between local cells (paracrine) and within cells (autocrine). Cytokines exist as small proteins that signal cells in response to injury and infection. One cytokine, interleukin-1, has been found to stimulate platelets and macrophages to release a variety of growth factors, including transforming growth factor–β (TGF-β). TGF-β is thought to be one of the factors (among others19,68,124) mediating Dupuytren’s Contracture.3 Cytokines regulate all aspects of tissue remodeling and may act positively or negatively on tissue damage. This variability results in a wide range of potential responses to injury between patients.
Critical Points PATHOPHYSIOLOGY
ASMA, α-smooth muscle actin; TGF-β, transforming growth factor–β.
TGF-β, released by platelets, tendon fibroblasts,137,138 and the joint capsule,52,53 has been identified as a key cytokine that both initiates and ends the process of tissue repair. Although other growth factors are involved in tissue remodeling, TGF-β is unique in its actions that enhance the deposition of extracellular matrix and regulate the actions of other cytokines.118,138 An overexpression of TGF-β1 results in progressive accumulation of matrix in tissues and an elevated number of myofibroblasts, leading to fibrosis.13,15,52,57,58,87 Border and Noble13 believe that repeated injury or trauma may also cause autoinduction of TGF-β beyond normal levels, creating a “chronic, vicious circle of TGF-β overproduction,” resulting in tissue fibrosis that may also occur in organ systems such as the kidney, liver, and lung. Investigators have documented that elevated levels of myofibroblasts and TGF-β occur rapidly, within 2 weeks after injury in experimental models, and that these changes are similar to those observed in humans with chronic elbow joint contractures.52,57,58
There is evidence that during the exaggerated fibrotic response that occurs in knee arthrofibrosis, a certain amount of tissue contraction or shrinkage occurs.16,111,131 Specific α-smooth muscle actin (ASMA)–expressing myofibroblasts generate tissue contraction during wound healing and are responsible for collagen overproduction during fibrotic diseases.28,34,38,46 TGF-β is capable of up-regulating ASMA and collagen in fibroblasts.
Unterhauser and associates147 measured the amount of ASMA-containing fibroblastic cells in arthrofibrotic tissue to determine whether the number of these cells was increased over that found in normal tissue. Tissue biopsies were obtained from 9 patients who underwent surgery for arthrofibrosis that had developed after ACL reconstruction. The patients all had primary arthrofibrosis and underwent the débridement procedure between 4 and 12 months after the ACL reconstruction. Tissue samples were also taken from 5 patients who underwent ACL reconstruction for chronic ligament deficiency and from 8 patients who had follow-up arthroscopy after ACL reconstruction for meniscus pathology; all 13 of these control patients showed no signs of arthrofibrosis. A significantly higher expression of ASMA-positive fibroblastic cells were found in the collagen bundles and remaining fatty tissue in the study group compared with both control groups (23.4% of the total cell count vs. 2.3% and 10.8%, respectively; P < .001). The authors concluded that this cell type is involved in scar formation and scar tissue contraction during the course of primary arthrofibrosis. They speculated that the expression of ASMA is most likely variable according to the time from the onset of the disease to tissue biopsy and probably decreases as the disease progresses.
Unterhauser and associates147 also reported that the fatty tissues in the arthrofibrotic knees were replaced by a dense collageneous network with parallel fiber orientation. A significantly higher total cell count and lower vessel density were measured in the arthrofibrotic knees compared with the control group who underwent ACL reconstruction. Similar findings were noted by Mariani and colleagues76 who biopsied 17 knees with arthrofibrosis that occurred after a variety of surgical procedures. The tissue samples were obtained less than 6 months from the onset of stiffness in 9 patients, from 6 to 12 months in 5 patients, and more than 12 months in 4 patients. The histologic evaluations demonstrated collagen-producing fibroblastic tissue with differing amounts of cellularity and vascularization. This study supports the hypothesis that arthrofibrotic tissue undergoes progressive remodeling and acquires the characteristics of mature collagen, all within the first 6 months after the onset of joint stiffness. There was no association between the amount of knee motion and the time from surgery or the degree of tissue maturation. The severity of loss of motion was associated only with the location and amount of adhesive tissue in the knee joint.
Unterhauser and associates147 concluded that the ratio of myofibroblasts to the total cells in connective tissue is a factor in the onset of arthrofibrosis. The authors suggested that future antifibrosis agents (such as decorin) be evaluated, because they inhibit the signaling pathway of TGF-β. Decorin is a human proteoglycan that inactivates the effect of TGF-β and may have a beneficial effect in arthrofibrotic tissues. Fukushima and coworkers45 reported that injection of decorin improved both the muscle structure and the function of lacerated muscle to near-complete recovery in mice. Several other experimental models have demonstrated the effectiveness of decorin in inhibiting adhesion formation in intra-articular adhesions,44 kidneys,14,64 and lungs.48 However, the effectiveness of this agent in humans with early or later-stage arthrofibrosis has not been investigated to date.58
In an in vivo small animal model, Bedair and associates8 showed that an antiotension II systemic receptor blocker decreased fibrosis and enhanced muscle regeneration in acutely injured skeletal muscle and hypothesized that the blocker modulated TGF-β1. Hagiwara and colleagues53 reported that the joint capsule has the potential to produce TGF-β1 and connective tissue growth factor, both of which play a role in causing fibrosis.
Zeichen and coworkers155 compared tissue samples of 13 patients with arthrofibrosis, obtained a mean of 16 months after the inciting knee ligament surgery, with those of 8 patients undergoing primary ACL surgery. Histologic examination from the patients with arthrofibrosis showed marked synovial hyperplasia, infiltration of inflammatory cells, and vascular proliferation with an increased level of collagen types VI and III. None of the control subjects demonstrated these findings. The authors noted that this confirmed the observations of Murakami and associates88 regarding the association between an inflammatory process and the proliferation of fibroblasts. The suggestion was made that collagen type VI could play a contributory role in the deposition of extracellular matrix that leads to arthrofibrosis.
Bosch and colleagues17 advanced the hypothesis that primary arthrofibrosis is the result of an immune response. Tissue samples obtained from 18 patients between 4 and 48 months from the triggering event to surgical débridement demonstrated synovial hyperplasia with fibrotic enlargement of the subintima and infiltration of inflammatory cells. The authors believed their findings supported an immune response as the cause of capsulitis leading to formation of diffuse scar tissue. The clinical implication was to avoid further surgery or aggressive manipulation while patients are suffering from acute capsulitis and to wait 3 to 6 months for the inflammatory response to resolve before débridement is considered. As is discussed, too often, there is a delay in treatment that results in a permanent limitation of knee motion. Unfortunately, even though a delay in treatment may allow tissues to “quiet down,” the resulting fibrotic tissue that forms becomes well organized and dense and treatment becomes more difficult. It appears that in the future, chemical agents will become available to treat fibrotic response to injury by controlling elevated levels of myofibroblast up-regulators and fibrogenic growth factors.8,22,42,45,52,57,58,70,90
PREVENTION
Preoperative
After an acute ACL tear and before reconstruction, the surgeon-therapist team must manage pain, effusion, quadriceps weakness, knee motion limitations, and gait abnormalities. Effusion and hemarthrosis are treated with appropriate nonsteroidal anti-inflammatory drugs (NSAIDs), cryotherapy, compression, and limb elevation. Patients work with physical therapists to learn safe and effective muscle-strengthening exercises (such as straight leg raises, bicycling, mini-squats, wall-sits, calf raises, knee extensions from 90°–30°, hamstring curls, and swimming) and must demonstrate good quadriceps control without an extensor lag before consideration for surgery. Regaining normal or nearly normal knee motion is essential and may take several weeks after an ACL rupture. The exceptions are knees with a mechanical block to motion, such as a displaced meniscus tear, in which early surgical intervention is warranted to preserve and repair the meniscus. A decision regarding future ACL reconstruction is based on the patient’s activity goals and other factors (see Chapter 7, Anterior Cruciate Ligament Primary and Revision Reconstruction: Diagnosis, Operative Techniques, and Clinical Outcomes). Other exceptions are knees with concomitant posterolateral ligament ruptures who are candidates for surgical intervention within 7 to 10 days of the injury. Knees with concomitant MCL tears are treated conservatively for at least 4 to 8 weeks to allow the medial side structures to heal and regain motion and muscle strength before proceeding to ACL reconstruction (see Chapter 24, Medial and Posteromedial Ligament Injuries: Diagnosis, Operative Techniques, and Clinical Outcomes).
Intraoperative
Intraoperative
The principles of correct graft placement, tensioning, and fixation are paramount to avoid a limitation of knee motion postoperatively (see Chapter 7, Anterior Cruciate Ligament Primary and Revision Reconstruction: Diagnosis, Operative Techniques, and Clinical Outcomes). The ACL graft is placed within the femoral and tibial footprint, occupying the central two thirds or more of the footprint, to achieve an anatomic position that allows the graft to control the pivot shift and anterior tibial translation. To achieve an anatomic graft placement in the center of the tibial attachment may require a limited notchplasty to avoid a limitation of knee extension. For the femoral tunnel, an anteromedial portal drilling technique or a two-incision procedure is favored to obtain a central anatomic femoral footprint placement. An endoscopic transtibial drilling technique is not recommended.55 In most chronic ACL-deficient knees, there is an overgrowth of cartilage and spur formation in the femoral notch, which necessitates a notchplasty to prevent ACL graft impingement.