CHAPTER 21 Prenatal Testing and Reproductive Genetics
Until recently, couples at high risk of having a child with a genetic disorder had to choose between taking the risk or considering other reproductive options, such as long-term contraception, sterilization, and termination of pregnancy. Other alternatives included adoption, long-term fostering, and donor insemination (DI).
The ethical issues surrounding prenatal diagnosis and selective termination of pregnancy are both complex and emotive, and are considered more fully in Chapter 24 (p. 363). In this chapter, we focus on the practical aspects of prenatal testing and diagnosis, including prenatal screening, as well as some aspects of reproductive genetics.
Techniques Used in Prenatal Diagnosis
There are several techniques that can be used for the prenatal diagnosis of hereditary disorders and structural abnormalities (Table 21.1).
Technique | Optimal Time (Weeks) | Disorders Diagnosed |
---|---|---|
Non-Invasive | ||
Maternal serum screening | ||
α-Fetoprotein | 16 | Neural tube defects |
Triple test | 16 | Down syndrome |
Ultrasound | 18 | Structural abnormalities (e.g., central nervous system, heart, kidneys, limbs) |
Invasive | ||
Amniocentesis | 16 | |
Fluid | Neural tube defects | |
Cells | Chromosome abnormalities, metabolic disorders, molecular defects | |
Chorionic villus sampling | 10–12 | Chromosome abnormalities, metabolic disorders, molecular defects |
Fetoscopy | ||
Blood (cordocentesis) | Chromosome abnormalities, hematological disorders, congenital infection | |
Liver | Metabolic disorders (e.g., ornithine transcarbamylase deficiency) | |
Skin | Hereditary skin disorders (e.g., epidermolysis bullosa) |
Amniocentesis
Amniocentesis involves the aspiration of 10 to 20 ml of amniotic fluid through the abdominal wall under ultrasonographic guidance (Figure 21.1). This is usually performed around the 16th week of gestation. The sample is spun down to yield a pellet of cells and supernatant fluid. The fluid can be used in the prenatal diagnosis of neural tube defects by assay of α-fetoprotein (p. 328). The cell pellet is resuspended in culture medium with fetal calf serum, which stimulates cell growth. Most of these cells in the amniotic fluid, that have been shed from the amnion, fetal skin, and urinary tract epithelium, are non-viable, but a small proportion will grow. After approximately 14 days, there are usually sufficient cells for chromosome and DNA analysis, although a longer period may be required before enough cells are obtained for biochemical assays. Increasingly, sensitive polymerase chain reaction (PCR) techniques make direct DNA analysis possible without the need for culture.
Chorionic Villus Sampling
In contrast to amniocentesis, chorionic villus sampling (CVS), first developed in China, enables prenatal diagnosis to be undertaken during the first trimester. This procedure is usually carried out at 11 to 12 weeks’ gestation under ultrasonographic guidance by either transcervical or, more usually, transabdominal aspiration of chorionic villus (CV) tissue (Figure 21.2). This tissue is fetal in origin, being derived from the outer cell layer of the blastocyst (i.e., the trophoblast). Maternal decidua, normally present in the biopsy sample, must be removed before the sample is analyzed. Placental biopsy is the term used when the procedure is carried out at later stages of pregnancy.
Chromosome analysis can be undertaken on CV tissue either directly, looking at metaphase spreads from actively dividing cells, or after culture. Direct chromosomal analysis of CV tissue usually allows a provisional result to be given within 24 hours. Nowadays rapid, direct fluorescent in-situ hybridization (FISH) probing (p. 34), or DNA analysis by the multiplex ligation-dependent probe amplification technique (p. 66), is used to test for common chromosome aneuploidies prior to a standard karyotype following culture of CV tissue; this may also detect other chromosome abnormalities and balanced rearrangements. For single-gene disorders, sufficient CV tissue is usually obtained to allow prenatal diagnosis by immediate biochemical assay or DNA analysis using uncultured CV tissue.
Ultrasonography
Ultrasonography offers a valuable means of prenatal diagnosis. It can be used not only for obstetric indications, such as placental localization and the diagnosis of multiple pregnancies, but also for prenatal diagnosis of structural abnormalities not associated with known chromosomal, biochemical or molecular defects. Ultrasonography is particularly valuable because it is non-invasive and conveys no known risk to the fetus or mother. It does, however, require expensive equipment and a skilled, experienced operator. For example, a search can be made for polydactyly as a diagnostic feature of a multiple abnormality syndrome, such as one of the autosomal recessive short-limb polydactyly syndromes that are associated with severe pulmonary hypoplasia—invariably lethal (Figure 21.3). Similarly, a scan can reveal that the fetus has a small jaw, which can be associated with a posterior cleft palate and other more serious abnormalities in several single-gene syndromes (Figure 21.4).
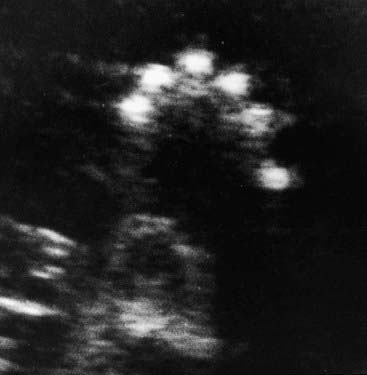
FIGURE 21.3 Ultrasonographic image of a transverse section of the hand of a fetus showing polydactyly.
Nuchal Translucency
In addition, the observation that increased nuchal translucency (NT) is seen in fetuses who are subsequently born with Down syndrome has resulted in the introduction of measurements of nuchal pad thickness (Figure 21.5) in the first and second trimesters as part of screening for Down syndrome (p. 273). In fact, the finding is not specific and may be seen in various chromosomal anomalies as well as isolated congenital heart disease.
Fetoscopy
Fetoscopy has also been used to obtain samples of tissue from the fetus that can be analyzed as a means of achieving the prenatal diagnosis of several rare disorders. These have included inherited skin disorders such as epidermolysis bullosa and, before DNA testing became available, metabolic disorders in which the enzyme is expressed only in certain tissues or organs, such as the liver—e.g., ornithine transcarbamylase deficiency (p. 172).
Cordocentesis
Although fetoscopy can also be used to obtain a small sample of fetal blood from one of the umbilical cord vessels in the procedure known as cordocentesis, improvements in ultrasonography have enabled visualization of the vessels in the umbilical cord, allowing transabdominal percutaneous fetal blood sampling. Fetal blood sampling is used routinely in the management of rhesus iso-immunization (p. 205) and can be used to obtain samples for chromosome analysis to resolve problems associated with possible chromosomal mosaicism in CV or amniocentesis samples.
Radiography
The fetal skeleton can be visualized by radiography from 10 weeks onwards, and this technique has been used in the past to diagnose inherited skeletal dysplasias. It is now employed only occasionally because of the dangers of radiography to the fetus (p. 26) and the widespread availability of detailed ultrasonography.
Prenatal Screening
The history of widespread prenatal (antenatal) screening really began with the finding, in the early 1970s, of an association between raised maternal serum α-fetoprotein (AFP) and neural tube defects (NTDs). Estimation of AFP levels was gradually introduced into clinical service, and the next significant development was ultrasonography, followed, in the 1980s, by the identification of maternal serum biochemical markers for Down syndrome. These are discussed in more detail below. Where the incidence of a genetic condition was high, for instance thalassemia in Cyprus, prenatal screening came into practice, as described in Chapter 20 (p. 321). However, molecular genetic advances, rather than biochemical, mean that the range of prenatal screening is continuing to evolve.
Testing for cystic fibrosis and fragile X syndrome are available in the UK, mainly for those willing to pay privately, and in Israel, for example, a wide range of relatively rare diseases can be screened for on the basis that they are more common in specific population groups that were originally isolates with multiple inbreeding, and therefore certain mutations are prevalent. Besides Tay-Sachs disease (carrier testing in this case is biochemical; see Chapter 20), familial dysautonomia, Canavan disease, Bloom syndrome, ataxia telangiectasia (North African Jews), limb-girdle muscular dystrophy (Libyan Jews) and Costeff syndrome (Iraqi Jews) are among the conditions for which screening is available. It does not come free of charge but the level of uptake of this screening is high, revealing the lengths to which some societies will go in order to avoid having children with serious genetic conditions. As DNA testing becomes more automated, rapid, and affordable, there will be pressure from some quarters to screen for many conditions, even though they are individually very rare. This challenge is already emerging with the potential use of microarray CGH in prenatal testing. If the use of microarray becomes routine there may be great difficulty in interpreting the consequences of rare or unique copy number variants. This ethical challenge is discussed more fully in Chapter 24.
Neural Tube Defects
In 1972 it was recognized that many pregnancies in which the baby had an open NTD (p. 258) could be detected at 16 weeks’ gestation by assay of AFP in maternal serum. AFP is the fetal equivalent of albumin and is the major protein in fetal blood. If the fetus has an open NTD, the level of AFP is raised in both the amniotic fluid and maternal serum as a result of leakage from the open defect. Open NTDs fulfil the criterion of being serious disorders, as anencephaly is invariably fatal, and between 80% and 90% of the small proportion of babies who survive with an open lumbosacral lesion are severely handicapped.
Unfortunately maternal serum AFP screening for NTDs is neither 100% sensitive nor 100% specific (p. 319). The curves for the levels of maternal serum AFP in normal and affected pregnancies overlap (Figure 21.6), so that in practice an arbitrary cut-off level has to be introduced below which no further action is taken. This is usually either the 95th centile, or 2.5 multiples of the median (MoM); as a result around 75% of screened open spina bifida cases are detected. Those pregnant women with results that lie above this arbitrary cut-off level are offered detailed ultrasonography; which is usually sufficient to diagnose NTD. In fact, ultrasonography has more or less superceded maternal serum screening as a means of diagnosing NTD. Anencephaly shows a dramatic deficiency in the cranium (Figure 21.7) and an open myelomeningocele is almost invariably associated with herniation of the cerebellar tonsils through the foramen magnum. This deforms the cerebellar hemispheres, which then have a curved appearance known as the ‘banana sign’; the forehead is also distorted, giving rise to a shape referred to as the ‘lemon sign’ (Figure 21.8). A posterior encephalocele is readily visualized as a sac in the occipital region (Figure 21.9) and always prompts a search for additional anomalies that might help diagnose a recognizable condition such as Meckel-Gruber syndrome.
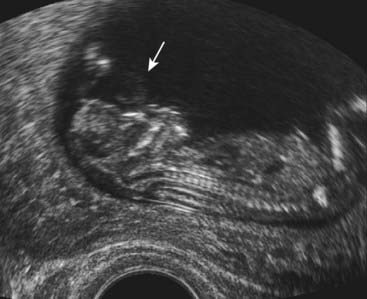
FIGURE 21.7 Anencephaly (arrow). There is no cranium and this form of neural tube defect is incompatible with life.
(Courtesy Dr. Helen Liversedge, Exeter, UK.)
A raised maternal serum AFP concentration is not specific for open NTDs (Box 21.1). Other causes include threatened miscarriage, twin pregnancy and a fetal abnormality such as exomphalos, in which there is a protrusion of abdominal contents through the umbilicus.
As a result of these screening modalities there has been a striking decline in the incidence of open NTDs in liveborn and stillborn babies. Other contributory factors are a general improvement in diet and the introduction of periconceptional folic acid supplementation (p. 258). In England and Wales the combined incidence of anencephaly and spina bifida in liveborn and stillborn babies fell from 1 in 250 in 1973 to 1 in 6250 in 1993.
Down Syndrome and Other Chromosome Abnormalities
The Triple Test
Confirmation of a chromosome abnormality in an unborn baby requires cytogenetic or molecular studies using material obtained by an invasive procedure such as CVS or amniocentesis (p. 325). However, chromosome abnormalities, and in particular Down syndrome, can be screened for in pregnancy by taking into account risk factors such as maternal age and the levels of three biochemical markers in maternal serum (Table 21.2).
Advanced age (35 years or older) Maternal serum |
MoM* |
α-Fetoprotein | (0.75) |
Unconjugated estriol | (0.73) |
Human chorionic gonadotrophin | (2.05) |
Inhibin-A | (2.10) |
* Values in parentheses refer to the mean values in affected pregnancies, expressed as multiples of the median (MoMs) in normal pregnancies.