Chapter 195 Postoperative Spinal Deformities
Definition
Postoperative deformity is an important complication, and a major reason for revision spine surgery. Revision rates in spine surgery have been reported variably, and the rates depend on the primary pathology treated, patient factors, methods of treatment, and choice of levels for arthrodesis.1–4 Postoperative deformity with adjacent-segment pathology is associated with significant disability and is one of the most common reasons for revision surgery.5–9 This chapter addresses the etiologies of postoperative spinal deformity and provides guidance for both avoiding and managing deformities after spine surgery.
Fusion of the Spine in Malalignment
Fusion of the spine with inadequate correction in the sagittal plane is a common cause of immediate postoperative deformity. Neutral sagittal alignment follows a vertical axis from C2, in front of T7, behind L3, and within 3 cm of the dorsal margin of the sacrum.10 There is significant normal variation of lumbar lordosis and thoracic kyphosis in maintaining overall sagittal balance.11,12 Sagittal balance is important for biomechanical optimization of forces at segmental interspaces. Sagittal plane malalignment is most often clinically significant when there is loss of normal lordosis of the lumbar and cervical spine. Excessive kyphosis across these mobile, unsupported segments increases intradiscal pressures and compromises the mechanical advantage of the erector spinae musculature.13,14 Clinically, the patient with fixed sagittal deformity presents with intractable pain, early fatigue, and a subjective sense of imbalance and leaning forward and difficulty with horizontal gaze. Compensation can be gained with extension of the hips, increased positive tilt (retroversion) of the pelvis, and flexion of the knees, although at the expense of increased fatigue.15
Flatback deformity or syndrome is the term commonly applied to postoperative malalignment of the spine. Flatback syndrome first was recognized by Doherty in 1973 as a postural disorder of the spine caused specifically by distraction instrumentation in the correction of scoliosis. Doherty recognized the loss of normal lordosis that occurred as a result of distraction across the lumbar spine and the thoracolumbar junction with the use of Harrington instrumentation for correction of coronal deformity in adolescent idiopathic deformity.16 Kyphotic decompensation syndrome is a more general term for postoperative kyphosis that is caused by immediate malalignment of the spine.17 Kyphotic decompensation may occur at a single segment of the spine or, more commonly, in fusion of the spine over multiple segments.
Fixation of the spine in coronal plane malalignment also is an important cause of immediate postoperative malalignment. Coronal plane malalignment after surgery may result in significant truncal shift, shoulder asymmetry, apparent leg-length discrepancy, pelvic obliquity with imbalance, rib-on-pelvis deformity, and complaints regarding appearance.18 A small malalignment or coronal tilt at the lumbosacral segments may cause a significant truncal shift and imbalance. A common cause of postoperative coronal malalignment is asymmetrical correction of balanced curves in a double major deformity or in a thoracolumbar scoliosis with a fixed lumbosacral obliquity.
Avoiding Fusion of the Spine in Malalignment
Preoperative planning and recognition of global sagittal and coronal plane alignment are important for avoiding postoperative malalignment of the spine.19 On physical examination, evaluation of the patient with knees fully extended is essential to assess the sagittal plane. Recognition of pelvic tilt (version of the pelvis) is important to determine whether a patient may be compensating for sagittal deformity in the spine with hyperextension of the hips and increase in pelvic tilt. In the coronal plane, correction of pelvic obliquity during the examination is important to assess coronal alignment of the spine prior to surgery. Leg-length discrepancy or apparent pelvic obliquity may be corrected with standing blocks to evaluate the spine with a level pelvis. Standing 36-inch radiographs are important in the evaluation of preoperative deformity and for identifying goals of surgical correction. Preoperative planning is based on calculations of sagittal and coronal changes needed for spinal realignment and may include digital image manipulation or modeling of the spine.20 Preoperative planning in deformity surgery may be characterized by significant variability within and between observers.21
During the procedure, patient positioning and intraoperative radiographs are critical for obtaining optimal alignment of the spine and for avoiding immediate postoperative deformity. Patient positioning has a significant impact on spinal alignment in the coronal and sagittal planes, and use of an appropriate operative frame to optimize passive correction of deformity and to avoid generation of deformity is important.22,23 Positioning the patient in a flexed posture may be useful for decompressive procedures, but arthrodesis of the spine with the patient in a knee-chest position, or in a flexed posture, is an important cause of postoperative deformity in even short-segment fusions.24,25 Intraoperative radiology is useful in assessing alignment of the spine after placement of fixation. Full-length, 36-inch radiographs obtained in the sagittal and coronal planes are most useful for assessment of global balance.
Treatment of Postoperative Malalignment of the Spine
When postoperative malalignment is recognized immediately after surgery, the patient may be treated with early revision and may not require osteotomies to correct a fixed deformity. Early revision may include improving segmental or regional sagittal alignment in a mobile spine with facetectomies or, more significantly, with Ponte osteotomies.26 In a patient with coronal imbalance due to asymmetrical correction of double curves, revision surgery may include improving the correction of the curve contralateral to the coronal offset or reducing the correction of the curve ipsilateral to the coronal offset Early detection of immediate postoperative deformity facilitates correction in a mobile spine.
The patient in whom postoperative deformity is recognized late, or after solid arthrodesis of the spine in a malaligned position, can present a difficult challenge for realignment. Osteotomies of the spine are the most commonly used technique for correction of postoperative malalignment.27 Surgical correction of fixed sagittal deformity was first reported by Smith-Petersen et al. in 1945.28 This osteotomy pivots on the posterior longitudinal ligament, with consequent lengthening of the anterior column through the disc space.29 The technique is well suited for patients with flexible discs ventrally and dorsal pseudarthrosis at multiple levels. Combined ventral and dorsal surgery offers the advantage of a controlled manipulation of the anterior column and fusion with structural graft. LaChapelle described a combined ventral and dorsal osteotomy for the management of ankylosing spondylitis that involved cutting the anterior column directly, followed by grafting to avoid risks of a ventral opening wedge.30 The combined ventral and dorsal osteotomy for the correction of iatrogenic lumbar kyphosis has led to good restoration of lumbar lordosis, maintained at more than 2 years’ follow-up.31
A dorsal-only approach to correction of sagittal deformity offers several advantages, including single-stage surgery, reduced morbidity compared with combined surgery, addressing the deformity at the apex, creation of compressive forces at the osteotomy site, and maximal correction with a minimal number of osteotomies.32 Dorsal surgical options can be categorized by the fulcrum across which correction is achieved. Dorsal osteotomies that hinge on the posterior longitudinal ligament (e.g., Smith-Petersen) cause opening of the anterior column. Spinal shortening osteotomies gain sagittal plane correction without distraction of the anterior column. Heinig’s eggshell decancellation procedure includes a controlled compression fracture of the anterior column with differentially more closure dorsally.33 In contrast, the Thomasen osteotomy maintains the height of the anterior column and hinges on the anterior longitudinal ligament.34 Asymmetrical closure of the pedicle subtraction osteotomy may permit up to 6 cm correction of coronal plane deformity as well as sagittal plane deformity.35 Larger corrections of trunk translation or coronal deformity may require a vertebral column resection.18,36,37
Case Examples: Immediate Postoperative Deformity
Case 1
A 54-year-old woman presented with a double major scoliosis. Asymmetrical flexibility of the lumbar and thoracic curves resulted in overcorrection of the lumbar curve relative to the thoracic curve and postoperative coronal decompensation. Realignment of the spine with an asymmetrical pedicle subtraction osteotomy (PSO) at L3 resulted in improvement of sagittal and coronal plane alignment (Fig. 195-1).
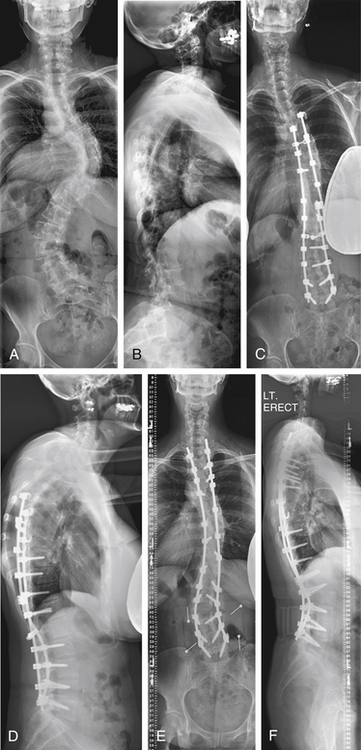
FIGURE 195-1 Preoperative standing anteroposterior (A) and lateral (B) radiographs. C and D, Initial postoperative appearance with asymmetrical curve correction. E and F, Final postoperative appearance with restoration of coronal and sagittal imbalance using an asymmetrical pedicle subtraction osteotomy.
Case 2
A 41-year-old woman was treated with combined ventral and dorsal surgery for lumbar adult scoliosis. The patient was fused in a fixed kyphotic and coronal plane decompensation. An asymmetrical PSO provided correction of deformity in the sagittal and coronal planes (Fig. 195-2).
Postoperative Decompensation
Late decompensation within the fused segments is most characteristic of incomplete fusion, or pseudarthrosis of the spine. Failure to achieve solid arthrodesis of the spine may lead to symptoms including progressive deformity, pain, dyspnea, and neurologic deficit. Pseudarthrosis of the spine is a contributing factor in as many as 80% of reoperations in failed spinal fusions.38–40 Symptomatic pseudarthroses are most common in the thoracolumbar and lumbosacral regions. Because revision surgery for symptomatic pseudarthrosis is characterized by high rates of recurrent pseudarthrosis and clinical failure,41,42 obtaining solid arthrodesis of the spine at the time of initial surgery is valuable because this may help avoid revision for pseudarthrosis. The role of biologics in improving arthrodesis in ventral dorsal spinal fusion surgery may reduce the burden of revision surgery for late postoperative deformity due to pseudarthrosis.43–45
Postoperative deformity due to pseudarthrosis may occur in both instrumented and noninstrumented fusions. Recognition and detection of pseudarthrosis may be challenging. The reported rates of pseudarthrosis are highly variable, and an important cause of observed variability in published rates is the difficulty of making a radiographic diagnosis of pseudarthrosis.46,47 In an instrumented fusion, pseudarthrosis may be apparent by implant loosening with osteolysis around screws, or by rod breakage. In noninstrumented fusions, identification of a pseudarthrosis may be more challenging. In a study correlating radiographic findings with intraoperative findings, Dawson et al. reported that plain anteroposterior and lateral views of the spine accurately identified a pseudarthrosis in only 48% of cases.48 The authors identified CT in the coronal plane as the most accurate study for identifying pseudarthrosis, with a 96% correlation with findings at surgery. Deckey et al. reported a finding of progressive deformity in 4 of 14 patients (28%) who underwent removal of instrumentation with an apparently solid fusion.49 This finding brings into question the effectiveness of even second-look surgery. DePalma and Rothman reported that the condition of pseudarthrosis is iatrogenic and responsibility for it lies entirely within the hands of the surgeon.50 Although surgical technique is an important consideration in the generation of a successful arthrodesis, other factors also have an important influence on the occurrence of nonunion in the spine, including the region of the spine fused, surgical approach, underlying diagnosis, implant stiffness, local conditions for bone formation, smoking, infection, and bone graft choices.7, 51–57
Crankshaft deformity of the spine is an important example of postoperative deformity within a fused region of the spine. In children with spinal deformity, progressive curvature, vertebral rotation, and rib prominence after a dorsal fusion may be due to continued anterior column growth of the spine despite a solid dorsal arthrodesis. This pattern of deformity progression was first identified by Hefti and McMaster.58 Duboisset et al. introduced the term “crankshaft phenomenon” as a descriptive term for this type of progressive deformity, making the analogy with an automotive crankshaft rotating around a center arm.59 Young patients who have significant ventral growth remaining at the time of a dorsal spine fusion are at risk of postoperative deformity progression, and incidences of 37% to 43% have been reported in patients who are Risser 0 and have open triradiate cartilages at the time of dorsal fusion.60,61 A more accurate radiographic parameter for predicting the risk of the crankshaft phenomenon after dorsal surgery may be the assessment of peak height velocity. Saunders et al. reported a high incidence (8/8) of crankshaft phenomenon in patients fused before reaching peak height velocity, and a low incidence (2/15) in patients fused after reaching peak height velocity.62 The risk of crankshaft phenomenon causing progressive deformity in children may be limited in the setting of contemporary rigid segmental instrumentation systems. Burton et al. reported only one case of progressive deformity after dorsal-only surgery in a cohort of skeletally immature patients, suggesting that ventral surgery may be unnecessary to prevent crankshaft deformity.63
Late decompensation of the unfused segments of the spine in patients undergoing selective thoracic or lumbar fusions for deformity is another important cause of postoperative deformity. It may lead to deformity in the sagittal or coronal plane. Late decompensation of the unfused segments in the sagittal plane is commonly due to proximal junctional kyphosis, a cause of postoperative deformity that is addressed in the next section of this chapter. Late decompensation in the coronal plane most commonly is due to progression of scoliosis in the unfused region of the spine. The choice of fusion levels at the time of initial surgery, and the techniques of correction used in the initial surgery are important determinants of the rate of late coronal plane decompensation in spine surgery.
In the coronal plane, decompensation after surgical correction of deformity may occur due to either deformity progression in the mobile lumbar spine or the exclusion of a structural curve from the segments chosen for arthrodesis. The development of progressive lumbar deformity after selective thoracic fusion, or fusion to the mobile lumbar spine, is an important cause of postoperative degenerative changes, pain, and decompensation in the coronal plane.64–66 Spontaneous correction of lumbar deformity may be reliably expected to occur in some cases of selective thoracic fusion with compensatory lumbar deformity.67 However, Miller et. al demonstrated an incidence of coronal decompensation after selective thoracic fusion of nearly 17% at 2 years in patients with adolescent scoliosis.68 Newton et al. demonstrated that factors determining the surgical choice to include a nonstructural lumbar curve in the fusion levels include the magnitude of the curve, the apical displacement, and the ratio of the size of the lumbar curve to the thoracic curve.69 Late decompensation of the lumbar unfused segments may occur secondary to degenerative changes. Cochran et al. reported a high incidence of retrolisthesis, facet joint changes, and disc degeneration subjacent to the fused levels in patients fused below L3 with Harrington instrumentation.70 Correction of thoracic deformity using derotation of the thoracic spine may increase rotation of the unfused lumbar segments.64,65,71