Physical and Chemical Methods of Control
After reading this chapter, the student should be able to:
• Describe the factors that need to be considered before deciding on the most appropriate method to be used for microbial control
• Differentiate between sterilization, disinfection, antisepsis, degermination, sanitization, and pasteurization and explain their practical uses
• Define microbial death and describe its significance in microbial control
• Describe the different physical methods that can be used in the control of microbial growth
• Define the terms microbicidal and microbiostatic
• Describe the factors influencing antimicrobial effectiveness
• Compare and contrast the methods used to measure the effectiveness of disinfectants and antiseptics
• Compare and contrast the different types of antimicrobial chemicals, and discuss the advantages and disadvantages of each
• Describe the different methods used in food preservation and explain their effectiveness in the control of microbial growth
Microbial Control
General Considerations in Microbial Control
The control of microorganisms in the environment is a never-ending concern in healthcare, in the laboratory environment (see Chapter 4, Microbiological Laboratory Techniques), as well as in various industries, especially the food industry. Microbial control can be achieved by physical methods, chemical agents, or a combination of both. Under ideal circumstances the methods used for microbial control should be inexpensive and fast-acting. Several factors need to be considered before deciding which method is most appropriate in a given circumstance. These factors include the following:
• Site to be treated: The nature of the site or item to be treated will determine the method of treatment. It needs to be determined whether or not the item is treatable with harsh chemicals, heat, or radiation, or a combination thereof, without undue damage to the item.
• Environmental conditions: Environmental conditions have an impact on the effectiveness (efficacy) of the antimicrobial method. For example, warm disinfectants generally work better than cold ones, because chemical reactions usually occur faster at warm temperatures, therefore reducing the time of exposure. Furthermore, certain chemical agents will perform better under acidic or alkaline conditions. Items contaminated with organic substances such as feces, vomit, blood, and so on need to be cleaned before disinfection or sterilization is performed for optimal results. Biofilms notoriously prevent the penetration of chemicals into all layers of the biofilms, and physical methods may have to be used before chemical methods can be effective.
• Susceptibility: Susceptibility of the microorganisms needs to be considered for any of the treatments (see later).
In addition to everyday management of microbial control, emergency departments and emergency medical services are responsible for the management of potential biological and chemical disasters caused by accident or by terrorist activities. Details on this topic are provided in the section First Responders to Natural Disasters and Bioterrorism in Chapter 24 (Microorganisms in the Environment and Environmental Safety).
Resistance of Microbes
Contamination with microbes is a concern in many environments; industrial, home, and healthcare, to name a few. The adequate control of these contaminants is necessary to avoid infections and disease. Although physical and chemical methods of control are available, the resistance of microbes to these methods of control varies greatly, depending on the type of microbe as well as the life stage the microorganism is in. Resistance ranges from the least resistant organisms to those with highest resistance (Box 19.1).
• Least resistant organisms include most bacterial vegetative cells, most fungal spores and hyphae, yeasts, enveloped viruses, and protozoan trophozoites.
• Moderate resistance is shown by some fungal sexual spores, protozoan cysts, and some viruses—mostly naked ones. Some of the most resistant viruses include hepatitis B virus and poliovirus. The bacteria Pseudomonas spp., Mycobacterium tuberculosis, and Staphylococcus aureus also are moderately resistant organisms.
• Highest resistance is demonstrated by bacterial endospores and prions. Endospores are considered the most resistant microbial form and when killed other nonpathogenic organisms are killed as well.
Terminology for Microbial Control
All healthcare professionals, scientists, and government workers need to be familiar with, and use the precise terminology pertaining to, the control of microbial growth (Table 19.1). Some of this terminology is also explained in Chapter 4 (Microbiological Laboratory Techniques).
TABLE 19.1
Terminology in the Control of Microbial Growth
Term | Definition |
Asepsis | Technique to prevent the entry of microorganisms into sterile tissues |
Antisepsis | Destruction of pathogens on living tissue |
Commercial sterilization | Sufficient treatment with heat to kill Clostridium botulinum endospores; used in the food industry |
Decontamination | Destruction, removal, or reduction of the number of undesirable microbes |
Degermination | Removal of microbes from a limited area (i.e., area of skin being prepared for injection) |
Disinfection | Destruction of vegetative pathogens |
Sanitization | Treatment to reduce microbial counts on eating and drinking utensils to achieve safe public health levels |
Sterilization | The complete destruction of all forms of microbial life to include endospores and prions |
Pasteurization
Pasteurization was introduced by Louis Pasteur in 1857 (see Chapter 1, Scope of Microbiology), using heat to kill vegetative bacteria and therefore reducing the number of microorganisms that have the potential to spoil food. To this day, pasteurization is used in the food industry in the preparation of milk, fruit juices, wine, and beer. Pasteurization does not kill all the microorganisms but reduces their numbers, or eliminates dangerous pathogens enough to prevent foodborne diseases and food spoilage.
Microbial Death
The destructive forces of chemical or physical agents act on the individual cells and if exposed intensively and long enough, the cell structures become dysfunctional and the cells show irreversible damage. In general, cells in a given culture/environment vary in their susceptibility to antimicrobial agents depending on their level of metabolic activity. Young, rapidly dividing cells have the tendency to die more rapidly than older, less active cells. When microorganisms are killed by any method of control, they have the tendency to display exponential death curves. In other words, they die at some fractional rate per unit time. If 50% of microorganisms in a population die every minute, after 2 minutes 25% will still be alive, after 3 minutes 12.5% are still alive, and so on (Figure 19.1). It is therefore safe to say that the total number of organisms present when the disinfection process began determines the time required to eliminate all microbes. The effectiveness of an agent is influenced by other factors besides time, such as:
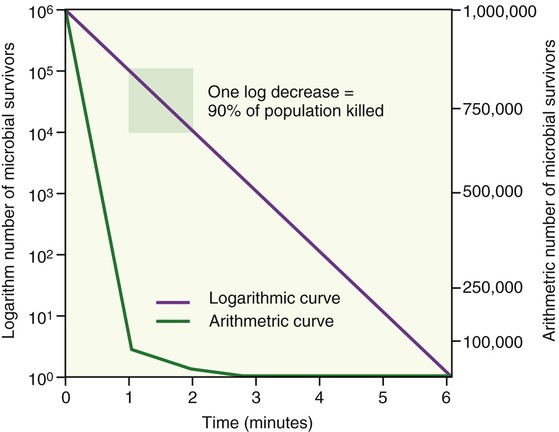
As this graph demonstrates, the microbial population shows an exponential death curve as the method of microbial control is applied.
• The number of microorganisms, which dictates the amount of time required for the destruction of all contaminants.
• The nature of the organism(s) to be destroyed. Biofilms, for example, include a number of different organisms and species. Other contaminants can include vegetative organisms as well as spores. Any target population that contains more than one organism can present a broad spectrum of resistance.
• The temperature and pH of the environment have an influence on the effectiveness of the antimicrobial agent.
• The overall concentration of the microbial control agent: Most are more active at higher concentrations.
• The presence of other materials, such as organic matter, solvents, and other substances, can inhibit or interfere with the actions of antimicrobial agents.
• Is the goal disinfection or sterilization?
• Will the item be discarded or is it reused?
• If dealing with a reusable item, which temperatures, pressures, chemicals, or types of radiation are acceptable?
• Will the treatment leave an undesirable residue?
• Is the agent able to penetrate to the necessary extent, that is, removal of biofilms?
• Is the process cost and labor efficient, in addition to being safe?
Physical Control
The optimal growth of most microorganisms depends on the most favorable environmental conditions for each species; most grow best in a narrow range of temperature, pH, osmotic pressure, and atmospheric conditions (Table 19.2). For example, obligate anaerobic bacteria will be killed instantly by exposure to oxygen. If the nature of the contaminant is known, the best agent of control can be determined rather easily and selectively. However, under most circumstances the exact nature of the microbe is unknown and rather stringent methods of decontamination will have to be employed.
TABLE 19.2
Optimal pH for the Growth of Some Bacteria
Bacteria | Minimal pH | Optimal pH | Maximal pH |
Thiobacillus | 1.0 | 2–2.8 | 4–6 |
Escherichia coli | 4.4 | 6–7 | 9.0 |
Clostridium sporogenes | 5.4 | 6–7.6 | 9.0 |
Pseudomonas aeruginosa | 5.6 | 6.6–7 | 8.0 |
Nitrobacter | 6.6 | 6.6–8.6 | 10.0 |
The nature of the contaminated item(s) or areas needs to be considered to efficiently and effectively remove the unwanted organisms without destroying the item. In the case of the food industry, nutrients might be inactivated by improper treatment (see Food Preservation, later in this chapter). A summary of physical methods for the control of microbial growth is provided in Table 19.3.
TABLE 19.3
Summary of Physical Methods Available for the Control of Microbial Growth
Method | Mechanism | Comments | Use |
Dry Heat | |||
Flaming | Burning to ashes | Sterilization | Inoculating loops |
Incineration | Burning to ashes | Sterilization | Dressings, wipes, animal carcasses |
Hot air sterilization | Oxidation | 170° C/2 h | Glassware, needles, glass syringes |
Moist Heat | |||
Boiling | Denaturation | Kills vegetative cells but not spores and prions | Equipment, dishes |
Autoclaving | Denaturation | Sterilization | Media, linens, equipment |
Tyndallization | Denaturation | Intermittent sterilization; free-flowing steam (30–60 min) for 3 d in a row | Substances that cannot withhold the high temperature of an autoclave |
Pasteurization | |||
LTLT (low temperature, long time) | Denaturation | 63° C for 30 min | Milk, milk products |
UHT (ultrahigh temperature) | Denaturation | 138° C for a fraction of a second | Milk, juices |
Low Temperatures | |||
Refrigeration | Slows down microbial growth | Bacteriostatic for most bacteria | Foods, drugs |
Deep freezing | Stops most microbial growth | Preservation | Foods, drugs, cultures |
Desiccation, Lyophilization, Osmotic Pressure | |||
Desiccation | Inhibits microbial growth | Loss of water; preservation | Foods |
Lyophilization | Inhibits growth | Loss of water; preservation; combination of drying and freezing | Storage of cultures, vaccines, cells |
Osmotic pressure | Plasmolysis | Loss of water | Food preservation |
Ionizing Radiation | |||
DNA destruction | Electron beams, gamma rays, x-rays | Sterilizing medical and dental supplies | |
Nonionizing | DNA damage | Ultraviolet light, visible light, infrared radiation, microwaves, radio waves | Disinfecting air; transparent fluids; germicidal lamps |
Temperature
Each species of microorganism has an optimal temperature range for growth, and any deviation from this will either slow down growth or stop growth altogether (Figure 19.2). For example, human pathogens prefer normal body temperature for optimal growth. In response to an infection, the human immune system (see Chapter 20, The Immune System) will increase the body temperature in an attempt to kill the invading microorganism. Microorganisms involved in the spoilage of food also generally prefer a moderate temperature for growth. Refrigeration commonly slows their growth, and appropriate cooking will kill the vegetative organisms. In other words, the majority of microbes that humans are most interested in controlling will be sensitive to abrupt changes in their environmental temperature.
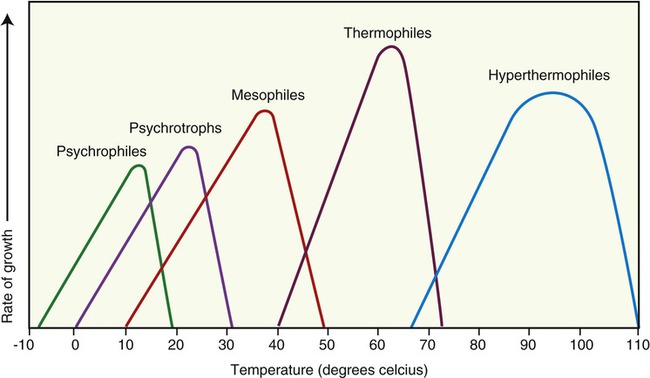
This chart shows the different classifications of microbes based on optimal growth characteristics. Note that although the classifications show definite differences in the temperature optimums for groups of organisms there is some overlap in the upper and lower limits of each classification.
Dry Heat
One of the simplest methods of dry heat sterilization is to expose the object to direct flaming or electric heating. If contaminated objects, such as wound dressings, are expendable, incineration is usually recommended, because it is the most rigorous of all heat treatments. Direct exposure to intense heat ignites substances and reduces them to ashes and gas. Incineration of microbial samples on inoculating loops or needles is a common practice in microbiology laboratories, but although it is fast and effective it is also limited to metals and heat-resistant glass materials. The method of flaming inoculating loops and needles also produces a potential hazard to the operator through the possible splattering of contaminants when the loop is placed into the flame. For this reason tabletop infrared incinerators (Figure 19.3) have replaced the traditionally used Bunsen burners in many laboratories. However, many clinical laboratories and also educational facilities are now using sterile, disposable, plastic inoculation loops and needles.
Moist Heat
Steam Under Pressure
The most dependable procedure to destroy all forms of microbial life with moist heat is steam sterilization under pressure. True sterilization using moist/wet heat requires temperatures higher than can be reached by boiling water. To achieve these higher temperatures pressure is applied to boiling water to increase the boiling point, and the temperature will proportionally increase with increasing pressure. A widely used instrument for pressurized steam sterilization is the autoclave (Figure 19.4).
Refrigeration and Freezing
• Refrigeration halts the growth of many pathogens because they are predominantly mesophiles, yet some pathogens are perfectly capable of reproduction in a refrigerated environment.
• Slow freezing forms ice crystals that can puncture cell membranes and therefore is generally more effective in inhibiting microbial metabolism than quick freezing; however, many bacterial cells, endospores, and viruses can survive ultralow temperature for years. Because they are able to become viable microbes given the appropriate temperature and medium, many bacteria and viruses can be stored in ultralow-temperature freezers for later use in the laboratory environment. Bacteria, viruses, and tapeworms can survive in frozen food for days and months, which needs to be considered when thawing and using frozen foods (later in this chapter).
Radiation
Ionizing Radiation
• Electron beams are highly energetic and very effective at killing microbes within just a few seconds. However, because they do not penetrate deep into matter they cannot be used to sterilize thick objects, or objects that are covered with a large amount of organic matter. They can be used for the sterilization of microbiology plastic ware, and medical supplies including gloves, syringes, and suturing material.
• Gamma rays penetrate deep into material but may require more time to sterilize large masses. Irradiation with gamma rays not only kills microbes, but also the larvae and eggs of insects. Gamma rays are often used for sterilization of disposable medical equipment, such as needles, syringes, cannulas, and intravenous sets. This type of radiation requires bulky shielding for the safety of the operators. Irradiation with x-rays or gamma rays does not make the materials radioactive. Irradiation is used by the United States Postal Service to sterilize mail in the Washington, DC area.
• X-rays penetrate the deepest but they have less energy than gamma rays and require too much time to be useful for the control of microbial growth.
Filtration
Liquids usually flow through filters by gravity, such as in coffeemakers, but in the laboratory environment a vacuum is often used to assist the movement of fluid through the filter (Figure 19.5). Filters originally were constructed from porcelain, glass, cotton, asbestos, and other special materials. Today’s scientists use membrane filters manufactured from nitrocellulose or plastic with specific pore and filter sizes. The pore sizes of these membrane filters range from 25 µm to less than 0.01 µm in diameter; these pore sizes are small enough to trap small viruses and even some of the larger protein molecules (Table 19.4).
TABLE 19.4
Pore Size (µm) | Microbes Retained |
5 | Multicellular algae and fungi |
3 | Yeasts and large unicellular algae |
1.2 | Protozoa and small unicellular algae |
0.45 | Largest bacteria |
0.22 | Largest viruses and most bacteria |
0.025 | Larger viruses, mycoplasmas, rickettsias, chlamydias, and some spirochetes |
0.01 | Smallest viruses |
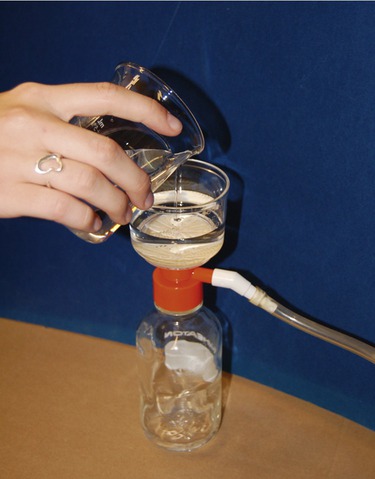
When supplements for media are not heat stable, the supplements may be sterilized by filtration through a membrane. This photograph shows a simple vacuum flask setup in which the liquid to be sterilized is pulled down through a funnel with a filter by a vacuum created in the lower collection flask. Filter pore size may vary, but the two sizes used frequently when filtering for bacteria/fungus and spores are 0.22 and 0.45 µm.
Chemical Control
Factors Influencing Antimicrobial Effectiveness
• Population size: Most agents kill organisms at a constant rate, and therefore require a longer period of time to kill or reduce a larger population.
• Population composition: As previously mentioned, the effectiveness of a chemical agent can vary greatly depending on the nature of the organisms being targeted. Because of differences in susceptibility, conditions in which there are a number of populations of different organisms may significantly decrease the overall effectiveness of the agent being used.
• Duration of exposure: As most agents kill at a constant rate, the longer the population of microbes is exposed to an agent, the more microbes are killed. Typically an exposure time long enough to reduce the probability of survival to 10–6 is required to achieve sterilization.
• Local environment: A bacterial population is surrounded and affected by various environmental factors that may alter the effectiveness of chemical agents. The formation of a biofilm is a good example of a population condition that could dramatically decrease the activity of a chemical agent.
• Concentration of the chemical agent: During the initial period of exposure, an increase in the concentration of a chemical agent will typically lead to a rise in the killing rate against the target organisms. Beyond a certain point, however, a concentration increase will not alter the killing rate. Sometimes a chemical agent is actually more effective at lower concentrations. For example, ethyl alcohol is more effective at 70% concentration than at 95% concentration because of the fact that as the alcohol denatures proteins, the higher concentration will cause the proteins to coagulate rapidly throughout the entire cell wall/membrane thus preventing a more complete penetration of the alcohol deeper into the cell. A lower concentration allows the denaturing process to proceed more slowly thus allowing a deeper penetration into the cell.
• Temperature: An increase in temperature often increases the effectiveness of a chemical agent, provided the chemical stability of the compound is not exceeded. It is frequently possible to use lower concentrations of a chemical agent if it is used at higher temperatures.
• Organic matter: The presence of organic matter such as feces, vomit, or sputum can decrease the effectiveness of chemical disinfectants.
• Type of microorganisms, numbers, possible presence of spores
• Physical situation, such as surface type and suspension
• Noncorrosive or nonstaining properties with regards to the surface being treated
• Available contact between disinfectant and microorganism
• Possible interaction between disinfectant and materials
• Concentration of the chemical: Remember, it is essential to follow the manufacturer’s recommendations for dilution of a concentrate
Evaluating Disinfectants
Disk-diffusion Method
In the disk-diffusion method a filter paper disk impregnated with a chemical disinfectant or antiseptic is placed on an agar plate on which a bacterial culture has been spread-plated. The chemical will diffuse from the disk into the agar around the disk. The size of the area of chemical infiltration around the disk is a function of the solubility of the chemical in agar, and of its molecular size. Microbes on the agar will not grow in the area around the disk if the organism is susceptible to the chemical. The area of no growth around the disk is called the “zone of inhibition” (Figure 19.6).
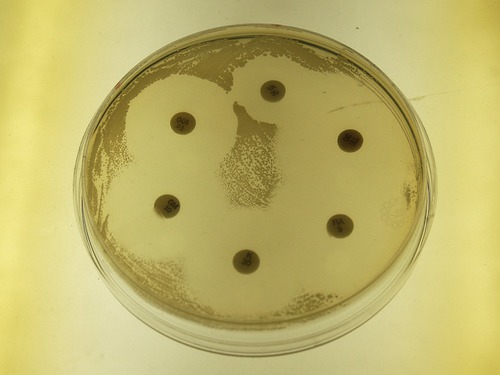
The Kirby-Bauer method for testing the effectiveness of antimicrobial agents uses a plate of Mueller Hinton agar on which a lawn of bacteria is spread. Disks impregnated with antimicrobial compounds are then placed on the surface of the plate, which is incubated for about 24 hours. The clear zones around the disks seen in this photograph are called zones of inhibition and are a relative measure of the effectiveness of the compound when used against a particular organism.
Antimicrobial Agents
A wide variety of chemical compounds is available to meet the varied requirements for control of microbial growth. The main groups of compounds include phenols and phenolics, halogens, alcohols, surfactants, quaternary ammonium compounds, heavy metals, and aldehydes (Table 19.5).
TABLE 19.5
Chemicals Used in the Control of Microbes
Chemical Compound | Effectiveness | Advantages | Disadvantages | Preferred Use |
Halogens | ||||
Chlorine | Kills most vegetative cells, some viruses and fungi | Good deodorant, inexpensive | Reduced by organic material; irritating odor and residue; solutions somewhat unstable | Purification of drinking water; disinfectant in food industry and hospitals |
Iodine | Kills vegetative cells, some spores and viruses, if used in high concentrations | Useful as skin disinfectant; can be used over a wide pH range | Irritating odor and residue, except with iodophors; toxic if ingested | Wound dressing, preoperative preparation, sterilization of dairy equipment |
Alcohols | Kill vegetative cells and many viruses and fungi | Unaffected by organic compounds; no residue; stable and easily handled; rapid effect | Flammable | Skin and surfaces |
Phenols and phenolics | Kill vegetative cells and some fungi; only moderately effective against spores | Stable to heating and drying; unaffected by organic compounds | Pure phenol is harmful to tissues; disagreeable odor; expensive; corrosive | In combination with halogens and detergents, make excellent disinfectants |
Surfactants | Disrupt bacterial attachment to surfaces | Inexpensive, usually nontoxic | Usually dislodge, not kill, bacteria | Surfaces including skin and counters |
Quaternary ammonium compounds | Kill bacteria (including staphylococci; Mycobacterium tuberculosis) and enveloped viruses | Stable in presence of organic compounds; easy to handle; no irritation residue | Hard water, detergents, and fibrous material can interfere with activity; can rust metals; low concentrations can support some bacterial growth | Small metal instruments |
Heavy metals | Kill some vegetative cells and viruses | Fast and inexpensive; no special equipment required | Inactivated by organic compounds and chemical antagonists | Rarely used except as preservatives and in fungal and protozoan infections |
Alkylating agents | ||||
Aldehydes | Readily kill staphylococci, TB, and viruses; spores killed on prolonged exposure | Wide range of activity; noncorrosive | Prolonged exposure may be required; irritating to tissue, can be toxic | Instrument disinfection |
Ethylene oxide (ETO) | Kills all microorganisms, including spores | Rapidly penetrates packing material | Extremely toxic, must be aerated; absorbed by porous materials, pure ETO is explosive | Sterilization of heat-sensitive materials and unwieldy objects in hospitals |
Alkylating Agents
• The aldehydes most often used in microbial control are glutaraldehyde, a liquid, and formaldehyde, a gas. Glutaraldehyde is a yellow acidic liquid and is one of the few chemicals that are accepted as a sterilant and high-level disinfectant. A 2% solution of glutaraldehyde will kill bacteria, viruses, and fungi within a 10-minute period of time for most objects. Spores and fungi can be killed within 3 hours, and an exposure time of 10 hours will achieve sterilization. Glutaraldehyde is less irritating and more effective than formaldehyde, but also more expensive. Formaldehyde is an irritating, strong-smelling gas that readily dissolves in water to form a solution called formalin. Although formalin is an intermediate to high-level disinfectant, it is extremely toxic (carcinogen), which limits its clinical usefulness.
• Ethylene oxide (ETO) is colorless, exists as a gas at room temperature, and is used as a sterilizing agent in hospitals and dental offices. It provides an effective method to sterilize and disinfect plastic materials and delicate, heat-sensitive instruments in hospitals and industries. Because of their toxicity and explosive properties, gaseous agents can be extremely hazardous to the people using them. Specially designed ETO sterilizers with safety features should be used by trained personnel only.
Food Preservation
Food poisoning is a common and usually mild illness; however, at times it can turn deadly. Symptoms include nausea, vomiting, abdominal cramps, and diarrhea, which occur within 48 hours of consuming a contaminated food or drink. Foodborne diseases (also see the Healthcare Application tables in Chapter 1, Scope of Microbiology) are caused by either the contaminating microbe itself or by its toxins. Infectious diseases can be spread through food or beverages and are a problem for millions of people in the United States and around the world. The Centers for Disease Control and Prevention (CDC) estimates that 76 million people annually suffer from foodborne illnesses in the United States alone, causing approximately 325,000 hospitalizations and more than 5000 deaths. According to the National Institutes of Health (NIH) the annual cost of all foodborne diseases in the United States is 5 to 6 billion dollars in direct medical costs and lost productivity. An increase in reported food poisoning cases has been noted by the CDC, and it is estimated that nearly 25% of the U.S. population suffers from some sort of food poisoning each year. It has been suggested that this increase is due to the mass production and distribution of processed foods, including raw vegetables, fruits, and meats. Any type of safety shortcuts and improper handling will lead to contamination of large masses of food with dirt, water, or animal wastes.
HEALTHCARE APPLICATION
Total Number of Foodborne Disease Outbreaks by Etiology: 2005 Summary Statistics*
Etiology | Number of Outbreaks | Number of Cases |
Bacterial | 188 | 4348 |
Chemical | 40 | 151 |
Parasitic | 6 | 739 |
Viral | 170 | 5018 |
Multiple | 6 | 525 |
Unknown etiology | 572 | 9398 |
Total: | 982 | 20,179 |
*Data assembled by the Centers for Disease Control and Prevention (CDC). Current updates from the CDC can be viewed at http://www.cdc.gov/foodborneoutbreaks/.
Pasteurization
Pasteurization (also see Chapter 1, Scope of Microbiology) is a method by which heat is applied to liquids to kill potential pathogens, as well as microbes that could lead to food spoilage, without changing the flavor and food value of the material. Pasteurization is designed to achieve a mathematical “log reduction” in the number of viable organisms to such a point that they are unlikely to cause disease. Although pasteurization does eliminate most pathogens, it does not sterilize, and heat-loving and heat-tolerant bacteria survive the procedure. These swimming organisms do not cause food spoilage over a relatively short time period, if appropriately refrigerated, and they are generally not pathogenic.
• HTST pasteurization can be achieved in two ways: by batch or continuous flow. In the batch process the fluid is held at 63° C for 30 minutes and then quickly cooled to about 4° C. In the continuous flow process, liquid is guided between metal plates or pipes that are heated from the outside by hot water.
• UHT pasteurization is done by holding the liquid at a temperature of 138° C for a fraction of second; products are accordingly labeled and can be stored at room temperature without microbial spoilage. After months the food prepared by this method may lose some of its flavor.
Other Methods
• Drying is probably the oldest method of preserving foods. The food will keep well because the water available for metabolism is so low that spoilage organisms cannot grow. Various methods of drying can be used, including sun drying, oven drying, and food dryers.
• Freezing does preserve foods and inactivate many microbes; however, once thawed these microbes can again become active and multiply under the right conditions, which then can lead to foodborne illnesses.
• Pickling is the process of preserving food by anaerobic fermentation in a salt solution to produce lactic acid. It also includes marinating and storing food in an acid solution, usually vinegar.
• Curing is the addition of some combination of salt, sugar, nitrite, and/or nitrate to meats for the purposes of preservation, flavor, and color. Salts inhibit microbial growth by plasmolysis. The concentration of salt needed to inhibit the growth of microorganisms is genus and species dependent.
• Fermentation typically refers to the conversion of sugar to alcohol by yeast under anaerobic conditions. Furthermore, fermentation implies that the action of microorganisms is desirable, and the process is used to produce products such as wine, beer, cider, and vinegar.
• Jams, jellies, and marmalades are thickened or gelled fruit products. Most of them are cooked and preserved with sugar, which creates an osmotic gradient inhibiting microbial growth.
Summary
• Most microorganisms are harmless, but many can cause infection and disease, water contamination, food spoilage, and other undesirable effects. Controlling them is a constant, never-ending concern in the healthcare environment, in laboratories, and in industry, especially in the food industry.
• Microbial control can be achieved by physical methods, chemical agents, or a combination of both in order to destroy, remove, or reduce microbes within a given area.
• Several factors need to be taken into consideration before a method of control is decided on, including the site or material to be treated, the type and number of microbes involved, the environmental conditions, the time available for management, as well as the effect desired.
• Sterilization is the elimination of all forms of microorganisms. Disinfection is the destruction of all vegetative microorganisms. The term disinfection when applied to living tissue is antisepsis. Degermination means the mechanical removal of most microbes within a given area, whereas sanitation provides a significant reduction of microbial numbers, and pasteurization kills enough microbes to prevent foodborne disease and food spoilage.
• Physical control of microbial growth involves different types of treatments such as temperature, osmotic pressure, radiation, and/filtration.
• Various types of chemical agents are used to control microbial growth on nonliving objects such as surgical instruments, laboratory and kitchen countertops, and toilets, as well as on living tissues, to mention a few.
• Antimicrobial chemicals are available in the form of liquids, gases, or solids, but few can achieve sterility; therefore chemical methods of control are aimed mainly at disinfecting nonliving items, and antisepsis of living tissues.
• Conditions such as the size of the microbial population and its composition, as well as the duration of chemical exposure, its concentration, the ambient environment, and temperature influence the effectiveness of antimicrobial agents.
• Formal testing of the effectiveness of antimicrobial agents is regulated by the Environmental Protection Agency (EPA) and Food and Drug Administration (FDA), and the efficacy of a disinfectant or antiseptic can be determined by the use-dilution test or the disk-diffusion method.
• Food preservation is achieved by a variety of techniques in handling, preparing, and treating food to prevent foodborne illnesses and to avoid or slow down food spoilage.
Review Questions
1. Decontamination is defined as the:
a. Killing of all microorganisms in a given area.
b. Reduction or removal of unwanted chemical or biological agents.
2. Which of the following organisms has higher resistance to most physical control methods than the others?
3. The cleaning of glassware and tableware in restaurants falls in the category of
4. Which of the following methods is the simplest heat-related method to sterilize metal?
5. Boiling items in water for __________ minutes will kill most vegetative bacteria and viruses.
6. The technique that combines freezing and drying to preserve microbes and other cells is:
7. Ionizing radiation involves all of the following except:
8. The term “zone of inhibition” is used in which of the following procedures?
9. Chlorine belongs to which of the following chemical groups?
10. All of the following are methods for food preservation except:
11. The destruction of vegetative organisms by chemical or physical methods is called __________.
12. The term used to describe an agent that causes microbial growth to come to a standstill is __________.
13. The lowest temperature by which all microorganisms in a particular liquid will be killed within 10 minutes is the __________.
14. Antimicrobial agents that are specifically designed to be used on living tissues are referred to as __________.
15. Agents that kill microbes are classified as __________.
16. Explain and differentiate between sterilization and commercial sterilization.
18. Name two organisms that are moderately resistant to antibiotics.
19. Describe the factors influencing the antimicrobial effectiveness of chemical agents.