Pharmacology and pharmacokinetics during the perinatal period
Pharmacokinetics refers to the processes involved in drug absorption, distribution, metabolism, and biotransformation and excretion (Figure 7-1). Site of absorption depends on route of administration (e.g., oral, intramuscular, intravenous, topical, inhaled). Drugs are carried in the blood, either bound to plasma proteins or as free drug. Protein-bound drugs provide a reservoir for future use, whereas free drug is the active component available to interact with the target cell. Biotransformation occurs primarily in the liver with a series of enzymatic reactions to modify and convert the drug into more polar (water-soluble) compounds. Intermediary steps in biotransformation may result in active metabolites. For example, codeine is demethylated to morphine, and in the neonate theophylline is methylated to caffeine.32 Biotransformation also takes place in the lungs, intestinal mucosa, and kidneys. Hepatic biotransformation occurs in two phases. Phase I (nonsynthetic reactions) takes place primarily in the microsomes, although some occurs in the mitochondria and cytosol. Phase I reactions modify the activity of a drug. Phase I enzymes include the cytochrome P450 (CYP450) monooxygenase system. Phase II (synthetic) reactions require adenosine triphosphate (ATP) and, except for glucuronidation, are extramicrosomal.32 Phase II reactions generally inactivate the drugs by converting them to more polar substances. Table 7-1 provides examples of drugs metabolized by various phase I and phase II reactions. Not all drugs undergo hepatic metabolism before elimination; some drugs are excreted directly via the kidneys.
Table 7-1
REACTION | EXAMPLES OF DRUG SUBSTRATES |
PHASE I (NONSYNTHETIC REACTIONS) | |
Oxidation | Phenytoin, phenobarbital, ibuprofen, acetaminophen, morphine, codeine, diazepam, cimetidine |
Reduction | Prontosil, chloramphenicol, ethanol |
Hydrolysis | Acetylsalicylic acid, indomethacin |
PHASE II (SYNTHETIC REACTIONS: CONJUGATIONS) | |
Glucuronide conjugation | Morphine, acetaminophen |
Glycine conjugation | Salicylic acid |
Sulfate conjugation | Acetaminophen, α-methyldopa |
Glutathione conjugation | Ethacrynic acid |
Methylation | Dopamine, epinephrine |
Acetylation | Sulfonamides, clonazepam |
Adapted from Chemtob, S. (2004). Basic pharmacologic principles. In R.A. Polin, W.W. Fox, & S.H. Abman (Eds.). Fetal and neonatal physiology (3rd ed.). Philadelphia: Saunders.
Variations in drug pharmacokinetics and pharmacodynamics occur among individuals at all ages, even with similar doses of a given medication, due to extrinsic (diet, environment, other therapies) and intrinsic (development, genetic constitution, diseases) factors.95 Currently much research focuses on understanding these factors, especially the contributions of individual genetic polymorphisms (see Chapter 1). This has led to new fields of study such as pharmacogenetics and pharmacogenomics. Pharmacogenetics is the study of “genetic variations that give rise to interindividual responses to drugs,”95, p.256 while pharmacogenomics integrates pharmacology and genomics into “the broader application of genome-wide technologies and strategies to identify both disease processes that represent new targets for drug development and factors predictive of therapeutic efficacy and risk of adverse drug reactions.”95, p.256 Genetic polymorphisms are small variations in an individual’s genome that may either be nonfunctional (have no effect on the individual) or modify expression of a protein that may alter the effects of exposures to environmental health hazards or result in individual differences in responses to drugs (changing efficacy, risk of adverse effects, or therapeutic dosing regimens).76,95 Understanding these variations, which is just beginning, can individualize pharmacologic management. During pregnancy, these individual differences may occur in the other, fetus and/or placental, or in any combination of these to influence the expression of specific genes involved in biotransformation, amount and activity of enzymes produced and thus levels of the drug and potential risks.76
The term drug is used generically in this chapter to refer to prescription and nonprescription pharmacologic agents, herbal agents, and vitamins, as well as drugs of abuse. In addition, the principles that govern placental transfer of drugs are also applicable to transfer of chemicals, food additives, and environmental agents. Definitions of common terms used in pharmacokinetics are summarized in Box 7-1 on p. 185.
Pharmacokinetics during pregnancy
Drug use during pregnancy
A survey of drug utilization by 14,000 pregnant women in 22 countries found that 86% of these women took some medication during pregnancy, with an average woman taking 2.9 medications (range, 1 to 15).73 A longitudinal survey from the United States surveyed 578 women throughout pregnancy. These women took a mean of 1.14 prescribed drugs per person, excluding iron and vitamins, with the most frequent being antibiotics, respiratory agents, gastrointestinal (GI) agents, and opioids. Of these women, 59.7% used at least one prescribed drug; 3.1% used more than five. In addition, these women took a mean of 2.95 over-the-counter drugs per person (92.6% of the women studied used at least one; 20.8% used more than five), with the most frequent being analgesics and agents for gastrointestinal or upper respiratory symptoms. Herbal agents were used by 45.2% of study participants, with a mean of 0.77 herbal agents per person; most frequently used were peppermint tea, cranberry juice, aloe, and other herbal teas.49 A retrospective chart review of eight U.S. health maintenance organizations (n = 152,531 women) from 1996 to 2000 found that 59% of the women received drugs other than vitamin or mineral supplements after their first prenatal care visit (39% in the first, 34.4% in the second, and 37.9% in the third trimester). Approximately one third of the women were on prescribed drugs for which there is evidence of some fetal risk (FDA category C, D, or X).8 More recently Mitchell and associates reviewed drug use during pregnancy between 1976 and 2008. They found that (1) average use of prescribed and over the counter drugs increased over these years from 2.5 to 4.2; (2) use of prescribed drugs increased 63% during the first trimester and by 2008, 94% of women took at least one prescribed drug; and (3) <1% of pregnant women took an antidepressant in 1988-90 versus 7.5% in 2006-08.89 Petersen et al. reported a four-fold increase in use of antidepressants during pregnancy from 1992 to 2006.104
Adherence to prescribed drug regimens is a problem during pregnancy, with up to 50% of women either not taking a prescribed drug or stopping before receiving a full course of the drug.27,72,104 This can lead to maternal risks, especially in women with chronic diseases.72,104 Peterson et al. found that antidepressants were more likely to be discontinued by pregnant than nonpregnant women, often leading to increased depression and other complications.104 Problems with adherence stem primarily from concerns about teratogenic effects. Most women overestimate the teratogenic risks of common agents.69 For example, in one study women personally estimated that the risk of having a baby with a birth defect was 25% (which is the risk associated with a potent teratogen such as thalidomide). After counseling, however, they more correctly estimated the risk at 5%.69 The background risk of a birth defect for the general population is 3% to 5%. However, the risk may be higher or lower for an individual woman depending on factors such as her health status, teratogen exposures, and genetic makeup.
Several principles have been identified to guide drug therapy during pregnancy: (1) avoid medications in the first trimester whenever possible; (2) anyone prescribing drugs for a woman of childbearing age must consider a potential pregnancy before prescribing; (3) women receiving long-term drug therapy require counseling before pregnancy of the potential implications and risks; (4) a necessary treatment should not be stopped without good reason; (5) drugs may have altered effects during pregnancy (e.g., the increased metabolism of methicillin or increased renal elimination of digoxin may result in decreased levels, resulting in the need for higher doses); (6) use single action, short-acting medications rather than long-acting or combination drugs; (7) use the lowest effective dose of the safest available medication; (8) use drugs only if the benefits outweigh the risks; (9) use alternate routes of administration if available (i.e., topical or inhaled rather than systemic agents); (10) select drugs that have a history of use during pregnancy without adverse effects, rather than the “latest” drug; and (11) use doses at the low end of the normal dose range while recognizing that for some drugs (e.g., digoxin, phenytoin, lithium), the woman’s usual dose may need to be increased due to increased volume of distribution (Vd) and clearance of these agents during pregnancy.73,109 These principles also apply to all women of childbearing age and to preconceptional and interconceptional use of drugs, in that approximately half of all pregnancies are unplanned and critical developmental processes occur before most women realize they are pregnant.73
Alterations in drug absorption, distribution, metabolism, and excretion during pregnancy
Maternal responses to drugs during pregnancy are influenced by both maternal physiology and the presence of the placental-fetal unit.81 Variations in handling of specific drugs by the pregnant woman may occur due to effects of the normal physiologic changes of pregnancy on drug absorption, distribution, metabolism, and excretion (Table 7-2). The presence of the placental-fetal compartment can also alter maternal kinetics.81 For example, many antibiotics are lipid-soluble and may readily cross the placenta and become sequestered in the fetal compartment and thus be unavailable to the mother. This can lead to lower maternal levels.
Table 7-2
Influence of Pregnancy on Physiologic Aspects of Drug Disposition
PHARMACOKINETIC PARAMETER | CHANGE IN PREGNANCY |
ABSORPTION | |
Intestinal motility | Decreased |
Ventilation | Increased |
Cardiac output | Increased |
Blood flow to skin | Increased |
DISTRIBUTION | |
Plasma volume | Increased |
Total body water | Increased |
Plasma proteins | Decreased |
Body fat | Increased |
METABOLISM | |
Hepatic metabolism | ± |
Extrahepatic metabolism | ± |
EXCRETION | |
Uterine blood flow | Increased |
Renal blood flow | Increased |
Glomerular filtration rate | Increased |
Ventilation | Increased |
From McClary, J., Blumer, J.L., & Aranda, J.V., et al. (2011). Developmental pharmacology. In R.J. Martin, A.A. Fanaroff, & M.C. Walsh (Eds.). Fanaroff and Martin’s neonatal-perinatal medicine: Diseases of the fetus and infant (9th ed.). Philadelphia: Mosby Elsevier, p. 711.
Evidence regarding effects, effectiveness, and safety of most pharmacologic agents during pregnancy is limited.69,80,87 Pharmacokinetic data on specific drugs during pregnancy is often minimal because pregnant women are excluded from drug trials. As a result, many drugs are not labeled for use in pregnancy, because the effects of the drug on the fetus are unknown.70,131 This is protective for the fetus but may also limit benefits to the pregnant women from these drugs. The net result of changes in drug handling during pregnancy depends on the individual drug, with both increases in levels (with need for a lower dose or less frequent dosing) and decrease in levels (with a need for a higher dose or more frequent dosing) seen.78 In general, both peak and steady-state serum concentrations are lower or unchanged in the pregnant woman.20 Although changes may not be clinically significant the efficacy and toxicity of drugs can be harder to predict, because of the physiologic changes during pregnancy that have the potential to alter pharmacokinetics.20,57
Little analyzed data on pharmacokinetics of drugs in pregnancy and found that across all agents (1) drug oral bioavailability decreased during pregnancy in 47% of studies of different agents; (2) Vd increased in 39%, with no change in 48%; (3) peak plasma levels decreased in 33%, with no change in 57%; (4) steady-state plasma levels decreased in 45%, with no change in 45%; (5) half-life decreased in 41%, with no change in 44%; (6) clearance increased in 55%, with no change in 34%; and (7) protein binding decreased in 86%.78 Therefore during pregnancy, there are considerable variations in the direction of pharmacokinetic changes among different drugs. This increases the complexity for the practitioner in choosing a specific pharmacologic agent and monitoring its effects. Many studies have significant methodologic problems, for example, sample sizes may be small, the composition of the control group may vary (e.g., the group may include nonpregnant women, adult males, or the same subjects postpartum), women of various gestational ages may be grouped together, and dosing methods may vary.36,78 Pregnancy drug registries have been established to collect experiences with specific drugs or groups of drugs (such as antiepileptic agents) during pregnancy and fetal and neonatal outcomes (see Box 7-2). Three types of drug registries have been established: individual academic sites; pharmaceutical drug company sites; and population-based sites (from individual countries or a group of countries).24,128
Drug absorption
Pulmonary, GI, and peripheral blood flow changes during pregnancy can alter absorption of drugs from the lungs, gut, and skin (see Chapters 9, 10, and 12). The increased minute ventilation that occurs in pregnancy can increase the rate of drug uptake across the alveoli.81,86 As a result, the pulmonary system may have a greater role in drug and metabolite excretion during pregnancy.91 Alveolar uptake of inhalation agents is also influenced by changes in cardiac output, which leads to increased pulmonary blood flow and thus increased alveolar uptake. As a result, doses of volatile anesthetics (e.g., halothane, isoflurane, methoxyflurane) may need to be decreased to compensate for these changes in the pregnant women.81,102 Inhaled aerosols such as anti-inflammatory and bronchodilators may have enhanced absorption.106
Absorption of oral medications is influenced by gastric acidity, gastric motility, presence of bile acids or mucus, nausea and vomiting of pregnancy, and intestinal transit time. During pregnancy there is decreased gastric acid (hydrochloric acid [HCl]) secretion, altered intestinal emptying time, increased mucus, and decreased gastric and intestinal motility.36,106 Decreased gastric motility can increase the oral bioavailability of slowly absorbed drugs (such as digoxin) by prolonging transit time, and can decrease peak plasma levels of rapidly absorbed drugs. Factors altering GI function in pregnancy may initially delay, then prolong absorption of oral medications. Peak plasma levels may be lower, and the time to peak levels may be later than in nonpregnant women. These changes tend to peak in the third trimester.68 Changes in pH affect ionization, and absorption of drugs.36,81 For example, decreased gastric pH slows the rate at which weak acids (e.g., aspirin) are absorbed and increases absorption of weak bases (e.g., narcotic analgesics).102 Decreased GI motility may alter initial absorption of oral antibiotics and lead to unpredictable patterns of intestinal absorption. Absorption of hydrophilic drugs may be enhanced due to the longer intestinal transit time (from delayed intestinal emptying and decreased motility).83 Increased cardiac output and peripheral blood flow may increase the rate of absorption of drugs from the stomach and small intestine.78 Iron chelates with some drugs taken concurrently, preventing absorption. However, even with these changes in GI function, the clinical effect for most drugs is not significant. This is because therapeutic windows are usually wide enough for drugs administered orally that these factors do not significantly alter therapeutic effects.91
Cardiovascular and integumentary changes during pregnancy may lead to more rapid absorption of agents delivered via transdermal, intranasal, intravascular, epidural, and subcutaneous routes.102 The increased extracellular water and peripheral blood flow to the skin during pregnancy may enhance absorption and alter distribution of topical agents. For example, topical and vaginal iodine-based preparations are not recommended during pregnancy because of the increased absorption of iodine. Iodine is of concern because it is actively transported across the placenta to the fetus, whose thyroid gland has a high avidity for this substance (see Chapter 19). Increased peripheral perfusion secondary to decreased peripheral vascular resistance and vasodilation may increase absorption of intramuscular drugs.36
Drug distribution
The distribution of drugs within the body depends on many factors, including the amount of body water, which affects Vd; degree to which a drug is bound to plasma proteins or body tissues; and the presence of the fetal-placental unit. For many drugs the Vd is increased during pregnancy because of the increased plasma volume, blood volume, cardiac output, total body water, and body mass.20,36,91 The increased plasma and blood volumes, and thus Vd, may result in decreased drug levels in the central compartment, reduce serum levels of drugs, and necessitate larger loading doses.83,103 Increases in total body water during pregnancy affect primarily water-soluble (polar) drugs that tend to stay in the extracellular space. Initial doses may need to be increased to achieve therapeutic concentrations; however, maternal doses for most drugs are not significantly altered since drug steady state is influenced by bioavailability and clearance as well as Vd.68 Due to changes in the Vd, some pregnant women may notice that their prepregnancy dose of a drug taken regularly, such as digoxin or phenytoin, may not achieve the same therapeutic effect during pregnancy.
Some lipid-soluble drugs (e.g., caffeine, diazepam, thiopental) have a longer half-life in the pregnant woman, whereas polar drugs (e.g., oxazepam, ampicillin) tend to have a shorter half-life.52,130 The Vd for lipophilic drugs is increased during pregnancy because of the accumulation of fat, which may serve as a reservoir for some drugs via tissue binding.36,78 Tissue binding is a mechanism by which a drug is removed from circulation and stored in tissue such as hair, bone, teeth, and adipose tissue. This mechanism can result in storage of significant quantities of a drug, because tissue storage sites may need to be saturated before there is sufficient free drug to be effective at receptor sites. Factors reducing maternal serum drug levels increase the risk of subtherapeutic drug levels. When the drug is discontinued, tissue deposits may give up their stores slowly, resulting in persistent drug effects. Because lipid-soluble drugs are stored in adipose tissue, the increased adipose tissue during pregnancy can lead to a slight decrease in the amount of free lipid-soluble drugs, such as sedatives and hypnotics, and persistence of drug effects (“hangover”) after the drug has been discontinued.
Drugs in plasma are either unbound (free) or bound to plasma proteins, primarily albumin or α1-acid glycoprotein. The reduction in plasma proteins, especially albumin, during pregnancy may increase plasma levels of free (active) drug. Albumin is the major binding protein for acidic drugs such as salicylates, anticonvulsants, nonsteroidal anti-inflammatory agents, and some neutral drugs such as warfarin and diazepam.81 Serum albumin levels fall during pregnancy, increasing the unbound or free drug fraction. Total plasma drug concentration stays the same during pregnancy, but the unbound fraction of albumin-bound drugs is greater; therefore the drug may be cleared faster or transported more rapidly across the placenta.81 Increases in free drug do not necessarily lead to an increase in plasma levels of that drug if the increase in free drug is balanced by more rapid hepatic biotransformation or renal elimination.36,81,102 However, with regard to doses of some drugs that are bound to albumin (such as many antiepileptic drugs), total and free drug concentrations may be altered, and doses may need to be adjusted during pregnancy. For example, phenytoin total plasma concentration is decreased by 55% to 65% and levels of free drug by 18% to 31% during pregnancy; carbamazepine total concentration is reduced 0% to 43% and free drug 0% to 28%; phenobarbital total concentration is reduced by 50% to 55%.102 Thus when monitoring serum concentrations of drugs such as phenytoin during pregnancy, free versus total levels should be evaluated (many laboratories report free levels if specified when ordering).36
Free fatty acids and steroid hormones, both of which are increased in pregnancy, may compete with drugs for albumin binding sites, further increasing levels of unbound drug. The decrease in albumin, coupled with increases in free fatty acids and steroid hormones, are most prominent in late pregnancy. This change can result in unpredictable transient increases in free levels of some drugs, such as phenytoin, valproate acid, carbamazepine, sulfisoxazole, theophylline, and phenobarbital as well as the potential for more rapid elimination increases the risk of subtherapeutic maternal levels.36,46 α1-Acid glycoprotein is the major protein for binding basic drugs such as local anesthetics, most opioids, and β-blockers. α1-Acid glycoprotein is decreased slightly during pregnancy.6,106
Changes in protein binding during pregnancy not only influence availability of free drug in the mother but also the amount of drug available to cross the placenta. This may alter fetal-to-maternal drug ratios, thus further altering placental transfer. Drugs with hepatic blood flow–limited biotransformation (e.g., propranolol, lidocaine) are rapidly eliminated in their first pass through the liver, regardless of whether they are bound or unbound.20,103 Therefore even with the decreased protein binding in pregnancy, hepatic clearance and total drug concentration generally are not significantly altered, although concentrations of free drug, Vd, and half-life may increase. Conversely, with drugs whose hepatic biotransformation is independent of hepatic blood flow (e.g., warfarin, phenytoin), only the unbound fraction is removed by the liver. For these drugs, total drug concentrations may be lower than usual, with a decreased half-life and greater fluctuations in peak and trough levels.
Hepatic drug metabolism
The hepatic changes during pregnancy (see Chapter 12) can alter biotransformation of drugs by the liver and clearance of drugs from the maternal serum. Drugs that are primarily (greater than 70%) metabolized in their first pass through the liver (high extraction ratio) are usually cleared rapidly by the liver, because clearance of these drugs is dependent on hepatic blood flow. Hepatic elimination of drugs with high extraction ratios is generally unchanged in pregnancy. Drugs with low (less than 30%) first-pass hepatic clearance (low extraction ratio) are more dependent on liver enzyme systems. The rate of elimination of these drugs (e.g., theophylline, caffeine, and diazepam) is related to free drug levels and tends to be decreased in pregnancy with increased half-lives. Hepatic elimination of other drugs—including many antibiotics, pancuronium, phenytoin, and acetaminophen—is increased in the pregnant woman.
Some liver enzymatic processes may be slower during pregnancy, delaying drug metabolism and degradation; other processes have increased activity. The cytochrome P450 (CYP450) monooxygenase system is a group of phase I enzymes essential for hepatic metabolism of drugs. The enzymes of this system are indicated by the prefix CYP (cytochrome) followed by a series of numbers and letters, (e.g., CYP1A2, CYP3A4, CYP2D6). Activity of the following are increased during pregnancy: CYP3A4 (50% to 100%), CYP2A6 (54%), CYP2D6 (50%), and CYP2C9 (20%).5,57,72,123 Up regulation of CYP2C9 decreases plasma concentrations and increases clearance of phenytoin, nonsteroidal anti-inflammatory agents and some oral antidiabetic agents.57,68 Changes to CYP3A4 may increase the metabolism of methadone, nifedipine, and indinavir during pregnancy.102,123 However, activity of CYP1A2, which is responsible for hepatic elimination of many drugs, is decreased (33% in the first, 50% in the second, and 65% in the third trimester); activity of CYP2C19 is also decreased (50%).5,6,78,123 CYP1A2 is also involved in clearance of caffeine so decreased activity may lead to slower clearance and enhance effects during pregnancy.72,102
Activity of phase II enzymes is also altered during pregnancy, including some of the uridine 5′-diphosphatase glucuronosyltransferase (UGT) enzymes.5,102,106 For example, UGT1A4 is increased 200% in the first and second trimesters and 300% in the third trimester; UGT2B7 is increased 50% to 200% by the third trimester.6,123 Some extrahepatic enzymes, particularly cholinesterase, are also decreased, which may alter the woman’s response to neuromuscular agents.28,36,131 For example, the decrease in pseudocholinesterase during pregnancy has been enough to impair breakdown of suxamethonium with subsequent prolonged paralysis after anesthesia in some women. The relative increase in hepatic metabolism and blood flow during pregnancy (as a consequence of the increased cardiac output), may increase clearance of polar drugs.36 In general, slowly metabolized drugs that are cleared primarily by the liver tend to be cleared more slowly in pregnancy due to decreased enzymatic activity and the net decrease in liver blood flow. This may also increase the length of time potentially teratogenic intermediary metabolites remain in circulation.
Clearance and metabolism of drugs by the liver during pregnancy may also be influenced by the increases in steroid hormones that stimulate hepatic microsomal enzyme activity. For example, progesterone may induce enzyme activity for phenytoin clearance, whereas progesterone and estradiol alter hepatic elimination of caffeine and theophylline by competitive inhibition of microsomal oxidase.36,81 Estrogens have cholinergic effects and may interfere with clearance of drugs such as rifampin that are excreted into the biliary system.81 Smoking and alcohol may also induce hepatic enzymes.
Renal drug excretion
Many drugs are excreted primarily by the kidneys. Thus the increased glomerular filtration rate (GFR) in pregnancy (see Chapter 11) alters renal excretion of drugs, especially water-soluble drugs such as penicillin G, digoxin, aminoglycosides, lithium, and pancuronium. These drugs are eliminated more rapidly, leading to lower and potentially subtherapeutic blood and tissue levels.36,78,91 These effects are most prominent in late pregnancy.72 In many cases the changes are not clinically significant enough to require alteration in drug dose.36 However, this is not always the case. For example, pregnant women with paroxysmal atrial tachycardia may experience an increased frequency of attacks when taking usual doses of digoxin. These women may require up to a 50% increase in their digoxin dose during pregnancy.81 In addition, shorter dosage intervals are recommended for nifedipine and labetalol due to a threefold increase in clearance in the pregnant woman.81 The half-life of atenolol, digoxin, and peripheral myorelaxants is shorter during pregnancy.102 Because lithium is eliminated entirely by GFR, the pregnant woman may require a higher dose of this drug, especially during the third trimester.88 More rapid clearance and shorter half-lives are also seen with many penicillins, cephalosporins, aminoglycosides, and sulfonamides as a consequence of the changes in renal function during pregnancy.102 Morphine metabolites may be cleared more quickly in late pregnancy and may lead to a lowered analgesic effect.72
Alterations in drug absorption, distribution, metabolism, and excretion during the intrapartum and postpartum periods
Drug handling is further altered during the intrapartum period. GI absorption may decrease to a greater extent than seen in pregnancy. Gastric emptying is reported to decrease during labor (see Chapter 12), although this may be secondary to use of opiate agents such as meperidine and pentazocine. Administration of antacids to decrease the risk of acid aspiration with anesthesia can alter ionization by altering gastric pH, prolonging gastric emptying, or complexing with drugs, reducing their absorption. Cardiac output increases in the intrapartum period further increase the Vd and may transiently alter hepatic and renal blood flow.101 Blood flow to the lower extremities may be reduced, especially if the woman is in a supine position. This may result in a slower and more unpredictable rate of absorption of drugs given intramuscularly in the buttock or thigh.101 Plasma nonesterified fatty acids rise during labor and may compete for albumin binding sites, with an increase in free drug levels.101 Both supine positioning and oxytocin may reduce renal blood flow and delay renal excretion of drugs and drug metabolites during labor. Most analgesics and anesthetics used in labor, especially opioids, tend to be low-molecular-weight, lipid-soluble, weak bases that enhance movement across the placenta to the fetus once they enter maternal circulation. The clinical effects depend on the timing of the dose, half-life, drug physicochemical characteristics, placental blood flow, and maternal and fetal drug metabolism and excretion.79
During the immediate postpartum period, the pattern of pharmacokinetics and drug distribution is similar to that of late pregnancy and remains so until the pregnancy-induced physiologic alterations of each system return to nonpregnant status.42 During this transition, drug handling by the woman may remain altered to some extent for some drugs. Transfer of drugs to nursing infants is discussed later in this chapter in “Drugs and Lactation.”
Transfer of drugs across the placenta
Dancis suggested that in thinking of placental transfer one should “Ask not whether a maternal [substance] crosses the placenta. Ask rather, how, how much, and how fast. Ask also as to fetal need.”35 There are few compounds, endogenous or exogenous, that are unable to cross the placenta in detectable amounts given sufficient time and sensitivity of detection.20,23,35,87,91 Drugs with similar physiodynamic properties can differ in placental transfer.87,105 Plonait and Nau suggest that if no other information is available and a group of drugs is not known to have teratogenic effects, then a comparison of lipid solubility, molecular weight (MW), ionization, and degree of protein binding might assist the clinical selection of an appropriate drug for more efficient transfer (fetal drug therapy) or least efficient transfer (limited fetal exposure).105 For example, drugs that are lipid soluble have an extremely low (50 daltons) or low (51 to 599 daltons) MW, and are nonionized with low protein binding in maternal plasma cross the placenta more rapidly and in higher concentrations than drugs that are water soluble (polar), have a high MW (greater than 600 daltons), and are ionized and highly bound to protein in maternal plasma. Transfer of drugs across the placenta varies with developmental changes in the placental, and thus gestational age.121
Patterns of transfer kinetics
Three major patterns of transfer pharmacokinetics are seen: (1) complete transfer (drugs rapidly equilibrate between mother and fetus, with blood flow being the rate limiting step); (2) exceeding transfer (levels are higher in the fetus than in the mother); and (3) incomplete transfer (levels are higher in the mother than the fetus).99 These patterns are illustrated in Figure 7-2. Examples of drugs with each pattern are listed in Table 7-3.
Table 7-3
Examples of Drugs with Transplacental Kinetics*
MODEL A OR B | MODEL C | MODEL D | MODEL E | MODEL F |
Some barbiturates: Thiopental Pentobarbital Secobarbital Antipyrine Promethazine Ritodrine Magnesium sulfate Digoxin |
Some benzodiazepines: Diazepam Lorazepam Desmethyl-diazepam Oxazepam Valproate Salicylate Nalidixic acid Nicotine Urea Some penicillins: Ampicillin Penicillin G Methicillin |
Ascorbate Colistimethate† Furosemide Meperidine Some cephalosporins: Cephalothin Cefazolin Cephapirin Cephalexin Some aminoglycosides: Gentamycin Kanamycin |
Amide-type local anesthetic agents: Lidocaine Bupivacaine Some β-adrenoceptor blockers: Propranolol Sotalol Labetalol Dexamethasone Cimetidine Ranitidine Methadone Some sulfonamides‡ |
Heparin Quaternary ammonium compounds: Tubocurarine Succinylcholine Vecuronium Pancuronium Elementary ions: Chlorthalidone Dicloxacillin Erythromycin Nitrofurantoin |
*According to models A through F in Figure 7-2. Differentiation between models A and B, C and D, and E and F is often uncertain because of incomplete data on the initial phase of drug distribution across the placenta. Model B is rarely applied.
†Polypeptide antibiotic, with a molecular weight of 1200 daltons.
Modified from Plonait, S.L. & Nau, H. (2012). Physiochemical and structural properties regulating placental drug transfer. In R.A. Polin, W.W. Fox, & S.H. Abman (Eds.). Fetal and neonatal physiology (4th ed.). Philadelphia: Saunders.
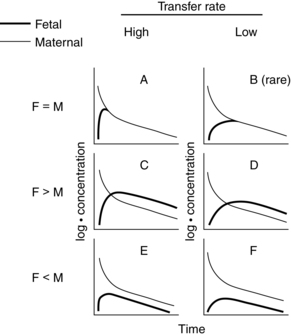
There is a great deal of variability even among drugs with similar pharmacokinetic characteristics. However, in general, complete transfer tends to be seen with drugs that have a high pKa and MW less than 600 daltons, whereas incomplete transfer is seen with drugs that have a high pKa and MW greater than 600 daltons. Strongly ionized drugs generally have incomplete transfer, although exceptions occur. For example, ampicillin and methicillin are strongly ionized but have lipophilic side groups, so they have a complete transfer pattern.98,101 Valproate and salicylates are ionized at the normal (physiologic) pH level but rapidly cross the placenta.81
Factors influencing placental transfer

Placental transfer is influenced by the following: (1) maternal and fetal drug handling; (2) surface area of the placenta; (3) diffusing distance between maternal and fetal blood; (4) physicochemical characteristics of the drug; (5) gradients (drug concentration, electropotential [normally 20 mV], and pH) between mother and fetus; (6) degree of binding of a substance to proteins in maternal and fetal circulations; (7) concentrations of binding proteins and protein binding gradients between mother and fetus; (8) permeability of the placental barrier (primarily the syncytiotrophoblast); (9) rates of maternal and fetal blood flow through the intervillous space and villi; and (10) placental influx and efflux transporters (Table 7-4).58,68,86,98,99,102,126
Table 7-4
Factors Influencing the Distribution of Drugs and Other Substances in the Fetus
FACTOR | POSSIBLE INFLUENCES |
Type of drug | Factors that increase transfer include lipid solubility, low molecular weight (less than 500 to 600 daltons for lipid soluble and less than 100 daltons for polar substances), nonioinized, and unbound. |
Amount of drug | Transfer is increased by greater maternal-to-fetal gradient, especially for drugs transferred by diffusion. |
Membrane permeability | Diffusion of substances increases with increasing gestation and greater placental efficiency. Some drugs have greater affinity for specific fetal tissues (e.g., tetracycline in teeth, warfarin in bones, phenytoin in the fetal heart [because this is a highly lipid organ in the fetus, whereas in adults the central nervous system has a high lipid content owing to myelin sheaths], streptomycin in the optic nerve). |
Fetal body water compartment | Drug distribution and dilution change as the total body water compartment decreases with increasing gestation. With an increased volume of distribution, peak volumes of drugs are reduced and excretion may be delayed. |
Fetal circulation | Maternal and fetal blood flow rates will influence transfer. Upon reaching the fetal circulation, drugs may be shunted by the ductus venosus past the liver (thus missing an opportunity for detoxification), with highest concentrations of these substances in blood going to the heart and upper body. |
Serum protein binding | Protein binding of a drug in the maternal system limits the amount of free drug available for transfer to the fetus. Binding of drugs to macromolecules in the fetal circulation may increase the maternal-to-fetal transfer by maintaining a concentration gradient from the mother to the fetus. |
Receptor function | Functional ability of receptors on cell membranes increases with gestation, leading to increased specificity to respond to or exclude certain drugs. |
Placental enzymes | Enzymes produced by the placenta (e.g., insulinase) may detoxify drugs and reduce transfer to the fetus. |
Gestational age | Many of the factors identified above are altered with advancing gestation and maturity of the fetus and placenta. The size and efficiency of the placental exchange area increase with increasing gestational age. |
Fetal pH during labor | The fetal pH is usually 0.1 to 0.15 units below that of the mother. A decrease in the fetal pH may increase the transfer of acidophilic agents from the mother to fetus. Hypoxia alters blood flow and thus drug distribution, metabolism, and excretion. For example, with hypoxia, blood flow to the liver and kidneys may be reduced with preferential flow to the brain. Albumin binding of drugs may also be reduced, resulting in more free drug in the fetal circulation. |
Diffusing distance and surface area.
Increased surface area for exchange, increased concentration gradient, and decreased diffusing distance enhance transfer across the placenta. With increasing gestation and placental growth, efficiency of the placental transfer increases as a result of the increased surface area. As the placenta matures, the distance between maternal and fetal blood decreases due to thinning of the syncytiotrophoblast and connective tissue and increases in the size and number of capillaries in the villi (see Chapter 3). Thus more drug passes to the fetus in the third trimester than in the first trimester. Transfer may be reduced with decreased surface area (small placenta, placental infarcts) or increased diffusing distance (placental edema, infection). Edema with fetal hydrops increases the diffusing distance and decreases transfer so drugs such as digoxin may be less effective in treating arrhythmias in the fetus with hydrops.71
Physiochemical characteristics and concentration gradients.
Physiochemical characteristics that influence movement across the placenta include lipid solubility, MW, degree of ionization, and protein binding. These characteristics can increase, decrease, or deter movement of potentially harmful drugs and other substances from the maternal to the fetal circulation.105 For example, placental transfer may be increased or enhanced if a substance is lipid soluble (e.g., diazepam, lipoproteins, most sedatives) or nonionized (e.g., phenobarbital), has a low MW (e.g., alcohol), or has a lower albumin binding (e.g., ampicillin). A substance will have reduced, slower, or no transport across the placenta if it has a certain charge or molecular configuration (e.g., heparin) or certain size (e.g., heparin, IgM), is altered or bound by enzymes within the placenta (e.g., amines, insulin), or is firmly and highly bound to the maternal red blood cell (e.g., carbon monoxide) or plasma proteins (e.g., dicloxacillin).
Lipid-soluble substances cross the placenta more readily than water-soluble substances, because cell membranes are made primarily of lipids that enhance diffusion. Lipid-soluble substances dissolve in the lipid membranes of the tissues separating maternal and fetal blood, primarily the syncytiotrophoblast. Polar substances cross slowly unless they have low MW and can move through pores. If the syncytiotrophoblast has a high lipid affinity for a drug (e.g., buprenorphine), the drug may be sequestered in the placenta with decreases in both maternal and fetal concentrations.120
The exact MW limit for placental transfer is unknown, although transfer of substances with MW >500 to 600 daltons is limited.105 Insulin (with an MW of more than 6000) does not cross the placenta because of its size and configuration and because of the effects of placental insulinase enzymes.36,99 Substances with higher MW tend to cross more slowly and are influenced by maternal concentrations; for example, if maternal concentrations are high, the substance is more likely to cross. These substances may also cross via active transport if a carrier is present. Immunoglobulin G (IgG) with an MW of 150,000 daltons is transported across the placenta via endocytosis and exocytosis (see Chapters 3 and 13).37 Water-soluble substances with an MW of less than 100 daltons diffuse readily; those with an MW greater than 100 diffuse more slowly. Small lipid-soluble substances (MW less than 500 to 600 daltons) cross rapidly and are blood flow–dependent; those with an MW of more than 500 to 600 daltons cross more slowly and may have incomplete transfer.20,91,120 However, MW is of limited value clinically in that most drugs have MWs less than 600 daltons. Exceptions include thyroid hormones (MW 889), insulin (MW greater than 5000), heparin (MW 20,000 to 40,000), and low-MW heparin (MW 3000 to 5000).52,68,99,102
Maternal-to-fetal concentration or electrochemical gradients also influence transfer. The maternal-fetal pH gradient influences transfer of drugs and can result in “ion trapping,” with higher concentrations of some drugs in the fetus than in the mother. Nonionized substances cross the placenta more efficiently than ionized substances.36 Normally the fetus and amniotic fluid are slightly more acidotic than the mother by 0.1 to 0.15 pH units.99 As a result of this difference, basic drugs may accumulate in the fetus and amniotic fluid with levels higher than in the mother. In the mother, a weak base tends to remain nonionized in her plasma and cross the placenta rapidly. As this weak base comes into contact with the more acidic fetal blood, the drug becomes more ionized, increasing the flow gradient from mother to fetus. The now-ionized drugs cannot return across the placenta to the mother as readily, thus becoming trapped in the fetus.20,81,99,120 For example, most opioids are weak bases that may accumulate in the fetus, which is one reason that effects of these drugs may persist in the neonatal period. The opposite effect is seen with acidic drugs: that is, these drugs are more likely to be ionized in the mother and thus not cross the placenta as readily.20 For nonionized, lipophilic drugs, the rate-limiting step for placental transfer is blood flow; for polar drugs, the rate-limiting step is diffusion.81 Hypoxia and acidosis in the fetus can influence transplacental passage of drugs. For example, local anesthetics are weak bases whose degree of ionization depends on pH. If the fetus becomes acidotic, ionization of these drugs increases with accumulation (“ion trapping”) in the fetus and neonate.47,99,102
Drug metabolites also cross the placenta to the fetus and vice versa. The majority of fetal drug elimination is by transfer back to the mother across the placenta. However, some elimination may occur by fetal metabolism, placental metabolism, or excretion into amniotic fluid.91 This transfer is also based on physicochemical characteristics, primarily ionization and lipid solubility. Most drug metabolites are polar, because polarity enhances biliary and renal elimination. Once polar substances are transferred to the fetus, elimination back across the placenta is slow and may result in accumulation in the fetus or amniotic fluid.91 In addition the fetus may metabolize a drug producing polar metabolites. These metabolites can also become trapped and accumulate in the fetus or be excreted into amniotic fluid and reswallowed by the fetus. Examples of drugs that tend to concentrate in amniotic fluid are the β-lactams, such as penicillin G, dicloxacillin, and amiodarone.36,91
Protein binding.
Drugs that are protein bound, usually to albumin or α1-acid glycoprotein, in the mother or fetus do not cross the placenta. Therefore plasma protein gradients between mother and fetus can also influence transfer. For example, ampicillin has a lower protein binding to albumin, thus increasing the amount of free drug that can cross to the fetus, and resulting in decreased maternal levels. Protein binding of dicloxacillin is 96%. Therefore dicloxacillin would be an appropriate choice to treat maternal infections, but not to treat intrauterine or fetal infection. Another example is propranolol, which is highly protein bound so it crosses much slower than atenolol, which has minimal binding.71
Maternal albumin levels fall during pregnancy and are about 15% to 20% lower than those in the fetus at term; α1-acid glycoprotein levels in the fetus are only about 37% to 40% of maternal levels.91,99,120 Thus at term, albumin (or fetal albumin macromolecule) bound drugs may be more highly bound in the fetus, whereas α1-acid glycoprotein drugs are more highly bound in the mother.91 Binding to proteins in the fetus may increase transfer of the drug by maintaining a concentration gradient across the placenta. Plasma protein binding and thus concentrations of drugs in the mother versus the fetus changes with increasing gestational age for some drugs. The decreased maternal albumin with increasing gestation increases the amount of free drug in the mother available for placental transfer. For example, diazepam binds to albumin. Albumin binding of diazepam is low in the fetus in the first half of gestation. However, in late gestation, as maternal serum albumin levels decrease, more free diazepam is present in maternal serum and, because this is a lipophilic drug, readily crosses the placenta. Thus by term, fetal diazepam levels are greater than maternal levels (Table 7-5).105 This may increase the risk of neonatal hyperbilirubinemia in that diazepam competes with bilirubin for albumin binding (see “Neonatal Pharmacokinetics”).
Table 7-5
DRUG | PRIMARY BINDING PROTEIN | BOUND IN MOTHER (%) | BOUND IN FETUS (%) | FETUS/MOTHER TOTAL PLASMA CONCENTRATION RATIO |
Betamethasone | AGP | 60 | 41 | 0.33 |
Bupivacaine | AGP | 91 | 51 | 0.27 |
Diazepam | ALB | 97 | 98.5 | 1.6 |
Lidocaine | AGP | 64 | 24 | 0.66 |
Mepivacaine | AGP | 55 | 36 | 0.71 |
N-desmethyl diazepam | ALB | 95 | 97 | 1.7 |
Phenobarbital | ALB | 41 | 36 | 1.0 |
Phenytoin | ALB | 87 | 82 | 1.0 |
Salicylate | ALB | 43 | 54 | 1.2 |
Valproic acid | ALB | 73 | 88 | 1.7 |
AGP, α1-Acid glycoprotein; ALB, albumin.
From Plonait, S.L. & Nau, H. (2012). Physiochemical and structural properties regulating placental drug transfer. In R.A. Polin, W.W. Fox, & S.H. Abman (Eds.). Fetal and neonatal physiology (4th ed.). Philadelphia: Saunders.
Placental blood flow.
The rate of maternal blood flow to and through the intervillous space and fetal blood flow to and through the villi influence placental transfer, particularly of nonionized, lipophilic substances such as anticonvulsants, alcohol, and opioid derivatives. Contractions decrease transfer so drugs that cross rapidly and are given during a contraction may demonstrate decreased transfer to the fetus. Contractions may also delay clearance of drugs from the fetus.120 In addition to uterine contractions, factors that may alter uteroplacental blood flow include maternal position; anesthesia; nicotine, β-lactams, ototoxics and other drugs; emotional or physical stress; and degenerative changes within the placenta that are seen with hypertension, prolonged pregnancy, diabetes, or renal disease.71
Route of administration.
The route of administration can also affect placental transfer. For example, nalbuphine that is given intramuscularly results in fetal blood levels that are 80% of maternal venous levels; if it is given intravenously, fetal levels are three to six times greater than maternal levels.81,99 Peak drug concentrations in the fetus (and the infant, if delivered after maternal administration) do not necessarily occur at the same time as in the mother. For example, meperidine peaks at 30 to 50 minutes in the mother, but 90 minutes to 3 hours after maternal administration in the fetus as a result of transfer kinetics across the placenta. Therefore meperidine levels are highest in the infant if given to the mother 90 minutes to 3 hours before delivery; on the other hand, fentanyl is higher in the neonate than mother if given shortly before delivery. Therefore it is reasonable to give meperidine if birth is expected within the hour.
Antibiotics are some of the most commonly prescribed drugs in pregnancy. Because antibiotics cross the placenta primarily by simple diffusion, rate-limiting factors include maternal-fetal concentration gradients, MW, and other physicochemical characteristics of the drug, placental surface area, diffusing distance, and the degree to which the drug is bound to maternal plasma proteins (see Table 7-5). A general pattern of antibiotic distribution to the fetus has been described. After an intravenous dose, peak maternal serum concentrations are seen within 15 minutes then fall exponentially. Peak umbilical cord values are seen in 30 to 60 minutes followed by an exponential fall. Peak amniotic fluid levels are seen in 4 to 5 hours. This lag results from slow excretion in fetal urine due to fetal renal immaturity.114
Placental transporters.
Although many drugs cross the placenta by simple diffusion, some drugs use placental influx or efflux transporters. These transporters are located along the apical membrane of the microvillus brush border facing maternal blood in the intervillous space and on the syncytiotrophoblast basal membrane (facing the fetal connective tissue matrix and blood vessels in the villi) or fetal capillary epithelium to facilitate movement of nutrients and other physiologic substances across the placenta.44,58,91,98,102,106,120,126 Some drugs may also be transferred across the placenta by nutrient (influx) transporters, including glucose, carnitine, monoamine, and organic ion carriers.102 Nonphysiologic substances—some drugs, environmental pollutants, and toxins—if structurally similar to nutrients, may compete for nutrient transporters. The drugs that seem to be particularly able to use this method to cross the placenta include amphetamines, cocaine, cannabinoids, and nicotine and other substances from cigarettes.44,129 By successfully competing with nutrients for these transporters, nutrient transfer is reduced and fetal growth and development altered. In addition, cephalosporins, ganciclovir, and corticosteroids cross by facilitated diffusion, probably by using carriers that normally transport dipeptides, hormones, and steroids.98
The placenta also contains a variety of efflux transporters whose role is to remove steroids and other potentially toxic substances and thus protect the fetus.68,71,102,120,129,135 Originally most of the transporters were identified in studies of multidrug resistance in tumors as is reflected in some of their names. Examples of drug efflux transporters in the placenta include members of the APT-binding cassette (ABC) group such as multidrug-resistant gene protein (MRP) 1, commonly called P-glycoprotein (P-gp), MRP-2, MRP-3, and breast cancer resistance protein (BCRP).58,68,102,121,126,129,135 P-gp, MRP-2, and BCRP are located on the apical membrane of the syncytiotrophoblast cells facing maternal blood; MRP-1 and MRP-3 are on the basal or fetal side of the trophoblast (see Figure 7-3).47,68,126 Efflux transporters are also found on the gut, lung, and blood brain barrier and help protect entry of substances into the body and brain. Efflux transporters are under hormonal regulation, for example, BCRP by estradiol, and progesterone and P-gp by glucocorticoids.126 Inflammation and infection may alter regulation of these transporters.89
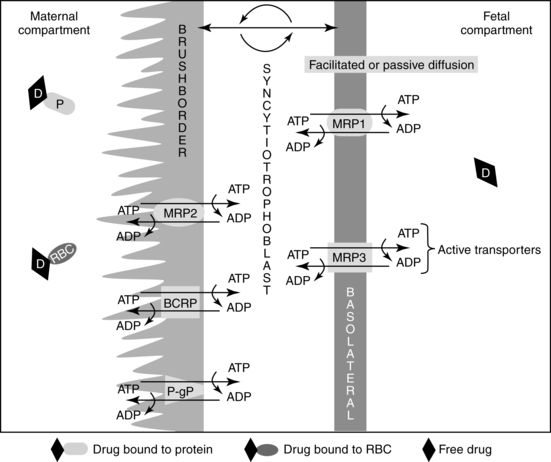
Each transporter appears to have specific substrates for which they provide protection. For example, studies of P-gp suggest that it exports cations, steroids, cytotoxic drugs, some antibiotics, opioids, phenobarbital, erythromycin, phenytoin, verapamil, and other substances that enter the placenta back into maternal blood, thus reducing fetal exposure.20,58,102,135 BCRP is involved in efflux of flavinoids, zidovudine, cimetidine, glyburide, nitrofurantoin, and the food-borne chemical carcinogen PhiP.58,68,102 MRP 1 and 3 prevent entry of organic anions and promote excretion and reduce entry of glutathione/glucuronide metabolites into the fetus.47 Some transporters may have bidirectional flow which can allow xenobiotics to be transported across the placenta to the fetus.68 Thus BCRP and P-gp cannot only decrease the amount of drug reaching the fetus but enhance removal of any drug reaching the fetal circulation.129 A drug that is a P-gp or BCRP substrate would be preferred for treating a maternal disorder with minimal fetal drug exposure, whereas a drug that is not a P-gp or BCRP substrate would be preferred for fetal pharmacotherapy.120
Deficiency of these transporter proteins increases the risk of teratogenesis from fetal drug and chemical exposure.58,135 Gene polymorphisms for the ABC efflux transporters may increase or decrease placental transfer of these substances and thus alter fetal susceptibility to effects of maternal drugs.58
Placental metabolism of drugs
The placenta has many enzyme systems, including various peptidases that can metabolize drugs, thus influencing transfer of specific substances from mother to fetus or fetus to mother.120 Both phase I and phase II drug metabolizing enzymes are present.106,129 Several isoforms of the CYP450 system (phase I reactions) are found in the placenta, although at levels about half of those seen in the liver. The primary CYP450 isoforms seen in the placenta are CYP1A1/1A2, although other isoforms, including CYP2E1, CYP3A4, CYP3A7, and CYP4B1, have also been reported, although with low activity.20,55,91,101,106,112,120