Pharmacology
After reading this chapter, the student will be able to:
• Name and explain the various branches of pharmacology
• Describe administration, absorption, and distribution of drugs in the body
• Explain how biotransformation aids elimination of drugs from the body
• Describe the dose–response curve
• Describe and explain the time–response curve
• Discuss the variability of drug action
• Differentiate between adverse drug response and toxicity
• Describe the steps necessary for the development of new drugs
• Name and describe the schedules used for categorizing drugs
Introduction
HEALTHCARE APPLICATION
Examples of Drugs Used in the Various Organ Systems
Drug | System/Condition | Effect |
Methylphenidate | Central nervous | Stimulant |
Digitalis | Cardiovascular | Treats congestive heart failure |
Colchicine | Neuromuscular | Analgesic |
Quinidine | Cardiovascular | Antiarrhythmic |
Procainamide | Cardiovascular | Antiarrhythmic |
Phenytoin | Central nervous | Anticonvulsant |
Verapamil | Cardiovascular | Antianginal |
Nitroglycerin | Cardiovascular | Antianginal |
Acetazolamide | Renal | Diuretic |
Levothyroxine | Endocrine | Treats hypothyroidism |
Barbiturate | Central nervous | Sedative |
Benzodiazepine | Central nervous | Antianxiety |
Prednisolone | Respiratory | Inhibits inflammatory responses |
Sodium bicarbonate | Gastrointestinal | Increases stomach pH |
Sildenafil | Reproductive | Treats erectile dysfunction |
Sibutramine | Obesity | Inhibits reuptake of serotonin and norepinephrine that regulate food intake |
Somatotropin | Endocrine | Antipituitary to block IGF action on liver cells. |
Branches of Pharmacology
This chapter introduces some fundamentals of “physiological pharmacology” or “pharmacological physiology”—referring to the same anatomy, physiology, and biochemistry discussed in Chapter 2 (Chemistry of Life) and Chapter 3 (Cell Structure and Function). Although all drugs are potential poisons (substances that cause severe distress or death), when given in subtoxic doses they are medicinal tools. The goal of rational drug therapy is to aid and maintain the body and its biological systems in healthy working order. In therapy, drugs give living tissues added chemical support to prevent and to meet physical and pathological challenges to which biological systems are exposed. Pharmacology is organized into separate disciplines that describe actions of drugs:
• Pharmacodynamics addresses drug-induced responses of the physiological and biochemical systems of the body in health and disease.
• Pharmacokinetics addresses drug amounts at various sites in the body after their administration.
• Pharmacotherapeutics addresses issues associated with the choice and application of drugs to be used for disease prevention, treatment, or diagnosis.
• Toxicology is the study of the body’s response to poisons; their harmful effects, mechanisms of action, symptoms, treatment, and identification.
• Pharmacy, on the other hand, includes the preparation, compounding, dispensing of, and record keeping about therapeutic drugs, for which drug nomenclature is essential. In addition, pharmacy includes the following:
Drug Nomenclature
• Nonprescription: Over-the-counter (OTC) drugs approved by the Federal Drug Administration (FDA) and herbal supplements, not approved by the FDA
• Prescription: Drugs required by law to be prescribed by licensed practitioners (i.e., veterinarians, dentists, and physicians)
Individual drugs have three names: chemical, generic, and proprietary or trade (brand) name (Table 21.1):
TABLE 21.1
Trade or Brand (Proprietary) Name | Generic (Nonproprietary) Name | Chemical Name | Therapeutic Class |
Amoxil, Amoxicot, Trimox, DisperMox … | Amoxicillin | (2S,5R,6R)-6-[(R)-(–)-2-amino-2-(p-hydroxyphenyl)acetamido]-3,3-dimethyl-7-oxo-4-thia-1-azabicyclo[3.2.0]heptane-2-carboxylic acid trihydrate | Antibiotic |
Advil, Motrin, Midol, Nuprin … | Ibuprofen | (±)-2-(p-Isobutylphenyl) propionic acid | Antiinflammatory |
Tylenol, Anacin-3 … | Acetaminophen | N-Acetyl-p-aminophenol | Analgesic |
Dilantin, Dilantin KAPSEALS | Phenytoin | Sodium 5,5-diphenyl-2,4 imidazolidinedione | Anticonvulsants |
Allegra | Fexofenadine | (±)-4-[1 hydroxy-4-[4-(hydroxydiphenylmethyl)-1-piperidinyl]-butyl]-α,α-dimethyl benzeneacetic acid hydrochloride | Antihistamines |
• Chemical: A single accurate description of the chemical structure, which is often lengthy but is of value to synthetic chemists; for example, 3,5-dihydroxyphenylalanine
• Generic: Nonproprietary, a single name, usually indicating the relationship of a drug to a therapeutic class; for example,
• Proprietary or trade (brand) name: A legally licensed drug name assigned by manufacturers. If a drug is produced by many manufacturers the list of proprietary names can become lengthy; for example, the local anesthetic procaine has at least 24 different names
Principles of Drug Action
Drugs alter physiological activity and for them to be effective they must reach their intended target site at the appropriate concentration (Table 21.2). This involves several processes collectively called pharmacokinetics. These principles include the following:
TABLE 21.2
Drug Losses at Sites of Action Through Administration
Route of Administration | Drug Loss From: |
Enteral route only | Degradation in stomach |
First-pass effect | |
Small intestine | |
Failure to be absorbed | |
Binding to food or other contents | |
Liver | |
Secretion in bile | |
Biotransformation | |
Tissue binding | |
Enteral and parenteral routes | |
General blood circulation | Biotransformation |
Binding to plasma proteins | |
Distribution to body tissues | Drug too dispersed, not sufficient at site of action |
Tissue binding | |
Biotransformation | |
Metabolism | |
Excretion |
Administration
For direct local action, drugs may be given (Figure 21.1) in the following ways:
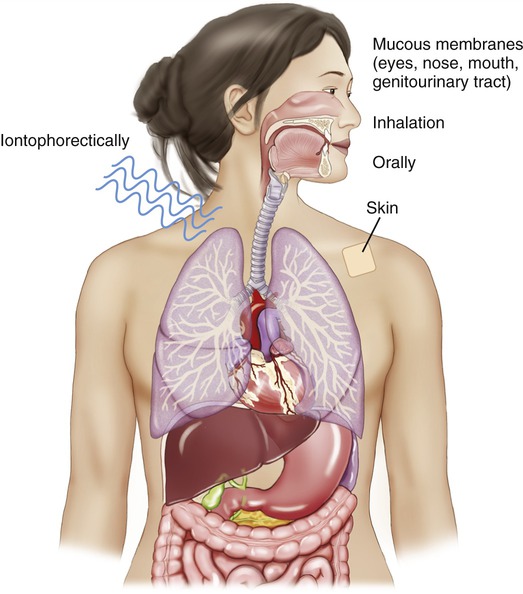
For direct administration, drugs may be administered to the skin or mucous membranes, orally, by inhalation, or iontophoretically through the skin by electrical charge to the given target.