CHAPTER 29 Percutaneous Catheter-Based Treatment of Coronary and Valvular Heart Disease
Although catheter-based therapy for the management of obstructive vascular disease in humans began with the pioneering work of Dotter and Judkins,1 the extension and application of catheter-based therapy to the management of obstructive coronary artery disease (CAD) is properly credited to Gruntzig, working in Switzerland, and Myler, working in the United States. The mid-to-late 1970s were a period of intense efforts toward the miniaturization of catheter design along with feasibility studies beginning with a canine model and extending to the operating room setting and, finally, the cardiac catheterization laboratory.2,3 With Gruntzig’s move from Zurich, Switzerland, to Atlanta, Georgia, in 1980, the technique and technology of what was then termed percutaneous transluminal coronary angioplasty (PTCA) developed rapidly.
First-generation angioplasty balloon catheters were crude (and hazardous) by today’s standards—generally 2 mm in diameter and “guided” into the target vessel by large-bore (9F to 10F) stiff guide catheters. The range of these early PTCA catheters was severely limited by the lack of optimal directional control and the large profile of the balloon catheter itself. Consequently, over the next several decades, the promise of this noninvasive approach to the management of patients with symptomatic coronary heart disease was realized with an explosion in the technology of catheter materials and manufacturing. Without such advances, the indications for this procedure would remain limited to clinically stable patients who are suitable for coronary artery bypass graft (CABG) surgery, and in whom a discrete, proximal, concentric, noncalcified, nonangulated stenosis in a large epicardial vessel was identified as the cause of disease (Fig. 29-1A).4
The early double-lumen design allowed for distal coronary artery pressure monitoring (Fig. 29-1B through D), enabling immediate assessment of the hemodynamic result of PTCA, and the large-bore guide catheter allowed for the injection of contrast material to assess the result fluoroscopically (Fig. 29-1E). As the popularity of PTCA grew, and the clinical and anatomic spectrum of disease encountered in diagnostic angiography laboratories expanded, it became clear, however, that (1) “lower profile” PTCA catheters would be necessary to treat many stenoses, and (2) improved “manipulability” and “directionality” were mandatory. PTCA catheter systems were modified with quantum leaps in technology—elimination of the double-lumen design (with consequent loss of ability to monitor distal pressure) and the creation of a (re)movable PTCA guidewire (in contrast to the fixed balloon–short wire design of prototypic catheters). These two fundamental changes, along with continued modifications in catheter and guidewire design, allowed for the explosive growth in PTCA procedures over the last 30 years. The development of “niche” devices,5,6 each designed for a specific anatomic situation, allowed for broader application in a wider spectrum of lesions and patients. During this explosive growth period in primarily nonballoon catheter technology, the procedure assumed a new name—percutaneous coronary intervention (PCI).
PROCEDURE
Description and Special Anatomic Considerations
Birfurcation-Type Lesions
When plaque is present at, around, or within the ostium of a significant side-branch (i.e., bifurcation [Fig. 29-2]), the chances of short-term and long-term ischemic complications are increased. There is much debate on the best way to approach these lesions. When the side-branch is small (<1.5 mm in diameter or subtending a small vascular territory), simply stenting over that side-branch is sufficient. In this situation, the chances of possible ischemic complications of displacing plaque into the side-branch, causing acute occlusion, are relatively small, and do not warrant increased procedural complexity and duration. When the side-branch approaches the diameter of the main branch or has a large vascular territory that it supplies, the ischemic complications of such plaque shift become significant. Myriad approaches to catheter-based management of bifurcation lesions have been described,7,8 which reflect the anatomic diversity of this category. Despite the use of stents and potent anticoagulant and antiplatelet regimens, however, clinical outcomes in patients with such anatomy remain suboptimal.9
Saphenous Vein Bypass Grafts
With the half-life of saphenous vein bypass grafts generally around 8 to 10 years, the number of post-CABG patients needing intervention continues to grow. Lesions in such older grafts (Fig. 29-3) present difficult issues: The veins themselves are characterized by diffuse fibrointimal hyperplasia even when nonobstructive—the vascular disease process continues to varying degrees, and a complex plaque consisting of platelets, thrombin, fibrin, inflammatory cells, and cholesterol crystals adheres to the endoluminal surface. Debris from large, bulky atherosclerotic plaque during PCI is frequently “liberated” downstream10; this often results in a “no-flow” phenomenon wherein such embolization results in occlusion of the distal vasculature with dramatic clinical consequences. Subsequently, it was found that the risk of peri-procedural ischemic complications during saphenous vein graft PCI can be reduced by up to 50% with the use of distal protection devices (Fig. 29-4).11
Complex Lesions, Angulated Lesions, and Lesions Distal to Proximal Vessel Tortuosity
Complex lesions, angulated lesions, and lesions distal to proximal vessel tortuosity (Figs. 29-5 through 29-7) also characterize “high-risk” anatomic subsets. Although especially true in the PTCA era, negotiation of severe tortuosity and proximal curvatures is still a challenge for even the most “low-profile” devices. Similarly, complex lesions, generally encountered in the setting of acute coronary syndromes, remain unpredictable in their response to PCI.
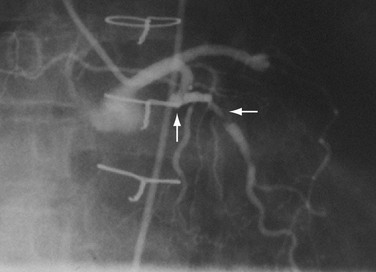
FIGURE 29-7 Complex lesion in circumflex marginal artery (horizontal arrow) distal to a sharp bend in artery (up arrow).
A key to working in tortuous arteries is having proper guide catheter support (i.e., adequate support to enable the PCI instrument to reach, and cross, the distal or postcurvature target lesion). The hazard here is dissection of the proximal vessel by the tensile forces exerted by the guide and, in some instances, propagation of the proximal vessel with complete occlusion. Advances in guidewire technology have vastly improved the ability to deliver various devices into or through excessively angulated arteries. The additional support provided by stiffer wires or wires specifically designed to decrease friction (by moving the balloon catheter or stent away from the arterial wall) has been a major advance in these difficult situations. Perhaps the most important improvements in these situations have been in stent design. Over the past decade, coronary stents have gone from very stiff and bulky catheter designs to designs that provide more flexibility and, consequently, deliverability. Not only are stents easier to pass to the lesion distal to a tortuous segment, but they are now also able to conform to angulated lesions with a minimal loss of radial force (Fig. 29-8).
Chronic Total Occlusions
Chronic total occlusion (Fig. 29-9), one of the last frontiers of interventional cardiology, refers to a native coronary artery (or vein graft) that has been occluded for at least 2 weeks. Such totally occluded vessels are commonly found during diagnostic catheterization. Although they may or may not be associated with a discernible myocardial infarct in the subtended territory, such anatomy still may predispose to symptoms of coronary insufficiency that medical therapy cannot treat adequately. In this setting, an attempt at recanalization is often contemplated.
When a wire is across the occluded segment, there can still be difficulty in getting the catheter into position. Even with immediate procedural success, chronic total occlusions are characterized by higher rates of restenosis compared with similar procedures in initially patent arteries. Currently, the presence of a chronic total occlusion is often the deciding factor in the decision to refer a patient to surgery rather than attempt a PCI, particularly when complete revascularization is desired in the setting of multivessel disease.12
Long Lesions and Diffuse Disease
The presence of diffuse disease (i.e., >20 mm in length [Fig. 29-10]) within a vessel was always one of the biggest dilemmas for PCI, and remains one of the largest contributing factors for restenosis. Restenosis rates after PTCA in long lesions can be 80%. Although stenting these lesions was often necessary for more angiographically acceptable immediate results, persistent rates of (in-stent) restenosis of 40% temper enthusiasm for this subset of lesions. The advent of drug-eluting stents has enabled these lesions to be successfully treated with dramatically reduced restenosis rates. These stents have successfully reduced restenosis rates and the need for repeat revascularization in patients with diffuse disease to the 9% to 20% range.13
Left Main Coronary Artery Disease
Traditionally, significant disease of the left main coronary artery (Figs. 29-11 and 29-12) has been one of the firmest indications for CABG surgery.14 When “protected” by a previous bypass graft to either the left anterior descending artery or the circumflex artery, these procedures can be done with a minimum of risk and high likelihood of success. In the unprotected state, however, the complexity of anatomy (typically a bifurcation or trifurcation) and the amount of myocardium at risk make these procedures high risk for the short-term and mid-term. In the United States, Canada, and most of Europe, left main coronary artery PCI remains a procedure used only for patients who have a prohibitive risk for CABG surgery. In parts of Asia and certain other countries in Eastern Europe and South America, these procedures have continued to be used on a wider basis.15 The main issues that have continued to limit wider acceptance of this procedure are the ramifications of the complications of in-stent restenosis and stent thrombosis (see later). When ongoing procedural and technologic modifications (e.g., designs to “fit” a bifurcation specifically) are established, PCI extended to this previously contraindicated group may become more frequent.16
ST Segment Elevation Myocardial Infarction
“Primary” PCI is considered the gold standard for treatment and has been compared with thrombolytic therapy multiple times.17 Traditionally, the preferred procedural method has been to perform an initial balloon angioplasty followed by stent placement for definitive recanalization. Only when a large thrombus burden was obvious was thrombectomy with either an aspiration or rheolytic device considered necessary (Fig. 29-13). Poor myocardial tissue level perfusion despite the apparently “normal flow” in the epicardial vessel has continued to be a significant problem, however, which has been linked to distal embolization of thrombus, plaque, or both. This problem has led to a resurgence in the use of aspiration or rheolytic thrombectomy devices (Fig. 29-14) as a means of initial reperfusion followed by stenting for definitive recanalization.18
Stent Thrombosis
Stent thrombosis (Fig. 29-15) is a dramatic event that can occur immediately, acutely (within 24 hours), subacutely (within 30 days), late (within 1 year), or very late (after 1 year). Stent thrombosis can be a devastating event, which frequently manifests with STEMI. Risk factors for early, acute, and subacute stent thrombosis include inadequate (or resistance to) antithrombotic or antiplatelet therapy, untreated dissection at the site of implant, prothrombotic substrate, and premature cessation of dual antiplatelet therapy. The treatment is emergent recatheterization, removal of thrombus with aspiration thrombectomy, or balloon angioplasty. It is also generally recommended to perform intravascular ultrasound to ensure that no mechanical complications, such as undetected dissection, undersized or underdeployed stents, or residual obstructive plaque proximal or distal to the stent, are present. These complications must be dealt with appropriately by ensuring proper expansion, using further stenting, maximizing medical therapy, and ensuring compliance. With the present armamentarium of devices, medications, and adjunctive procedures, stent thrombosis is a rare event (overall risk by 1 year approximately 1%).
Catheter Modification for Specific Lesion Subsets
As alluded to earlier, specific balloon catheter modifications were required for unique anatomic situations in which conventional technology frequently failed. These situations included lesions that were heavily calcified or characterized by extensive fibrosis. In either situation, the response to balloon expansion was either inadequate or excessively disruptive. The concept of “lesion modification” was introduced along with a technique called percutaneous transluminal coronary rotational atherectomy.19 Employing a high-speed rotating (160,000 to 180,000 rpm) diamond-studded “burr” mounted on a traditional balloon catheter, “pretreatment” of such lesions frequently enabled definitive lesion treatment with excellent immediate results (Fig. 29-16).
Persistently unacceptable rates of restenosis,20 the complexity (and risk) of the procedure, and further refinements in traditional catheter technology have reduced the use of percutaneous transluminal coronary rotational atherectomy from a significant minority of all PCI to a niche indication at present. The development of the less cumbersome “cutting balloon” (see Fig. 29-12) with the similar philosophy of plaque/lesion “modification” as percutaneous transluminal coronary rotational atherectomy has led to more widespread acceptance at present in these situations.21
Another limitation of traditional balloon angioplasty was the inability to treat eccentric lesions or lesions characterized by substantial plaque mass. An innovative catheter design, directional coronary atherectomy, allowed for more precise directional control, allowing for more specific lesion targeting and physical removal of the plaque (Fig. 29-17).22 Despite the intellectual attractiveness of this concept, and the often pristine angiographic appearance of the result, the technology was bulky and cumbersome. In addition, an excess hazard of directional coronary atherectomy was identified in one of the early randomized trials of device evaluation,23 further limiting the application of this truly innovative technique.
Indications
The indications for coronary revascularization procedures have been generally accepted through a consensus of opinion from the various academic societies and organizations that give direction to and advise the cardiology profession. The strongest and most agreed on indications (class I and IIa) are summarized from the American College of Cardiology/American Heart Association Clinical Guidelines on PCI from 2005 and the update from 2007.24,25
Stable Coronary Disease
Acute Coronary Syndromes
ST Elevation Myocardial Infarction
Outcomes and Complications
Largely as a result of the foresight of Gruntzig, Myler, and others, the assessment of procedural outcomes (short-term and long-term) was considered to be an inherent aspect of PTCA. The National Heart, Lung and Blood Institute Registry was established in 1979 and served as a rich source of clinical and procedural data.26 Detailed clinical and anatomic information provided the basis for the estimation of procedural risk.27 Although less applicable in the current “stent” era, the prediction of risk for adverse procedural outcomes could be linked to lesion morphology.28 Anatomic subsets that are associated with an increased risk for procedural failure, complication, or restenosis are bifurcation lesions, vein graft lesions, complex lesions, chronic total occlusions, and left main coronary artery disease. Examples of each of these anatomic subsets and the nontraditional catheter technology developed for such situations are discussed next.
The major adverse cardiac events after PCI are death, myocardial infarction, and emergent CABG surgery. Although the rates of these events, individually and in composite, decreased consistently throughout the PCI era, it was not until the advent of coronary stents and advances in adjunctive pharmacotherapy that procedural major adverse cardiac event rates overall declined to current levels of approximately 5%.29,30 Generally, the risk of adverse procedural outcomes can be assessed using a few easily identifiable preprocedural clinical variables.31,32 Important clinical subsets that are associated with an increased risk for procedural failure, complication, or restenosis are diabetes, left ventricular dysfunction, chronic kidney disease, and acute myocardial infarction. Additionally, the compounding and confounding effects of age must always be taken into account because there are biologic and statistical interactions between advanced age and each of the latter subsets.33
Diabetes
Diabetes mellitus not only increases the risk for coronary atherosclerosis, but also, when diagnosed, increases the risk for poor outcomes. Dyslipidemia is more prevalent and often more severe, renal disease is more frequent, and the atherosclerotic plaque burden is often more extensive and more diffuse than in nondiabetics. In addition, increased inflammatory markers, widespread endothelial dysfunction, and a prothrombotic state are linked to the diabetic condition. These factors conspire not only to make the acute outcomes of PCI less successful, but also contribute to the inferior long-term outcomes compared with nondiabetic patients.34 These inferior outcomes are particularly significant in patients who are receiving insulin therapy.
Diabetic patients clearly do better with revascularization, however. PCI and CABG surgery can provide important benefits, including symptomatic relief and, in many patients, improved survival. To date, randomized trials suggest that diabetics with multivessel disease fare better with CABG surgery than with PTCA with respect to survival and the need for repeat revascularization procedures.34 Ongoing trials comparing multivessel PCI (stent) with CABG surgery should help in defining which procedure offers greater benefit, and in which populations a specific strategy can be defined as the procedure of choice.
Long-term outcomes in diabetic patients are further compromised by increased risk of restenosis. The introduction of drug-eluting stents has had a great impact on the treatment of CAD in all patients, but more so in diabetic patients.35 The findings of lower restenosis and major adverse cardiac event rates when using drug-eluting stents compared with bare-metal stents36 have led to more diabetic patients being treated with PCI.
Left Ventricular Dysfunction
Left ventricular dysfunction is frequently found in patients with, and is often a result of, CAD. With proper medical treatment and, in many cases, revascularization therapy, left ventricular function may significantly improve. Although poor left ventricular function is often one of the indications for recommending a revascularization procedure, it also increases the risk for PCI and CABG surgery. The lack of cardiac reserve and the possibility of causing a decrease or loss of blood flow in arteries subtending large areas of myocardium or in collaterals supporting these areas are some of the purported mechanisms that increase the risk with left ventricular dysfunction.31,37,38 Various strategies have been employed to lessen procedural risk in very-high-risk patients with advanced left ventricular dysfunction,39–41 but data are uncontrolled and inconsistent in supporting the need for such extreme measures.
Chronic Kidney Disease
The presence of preexisting renal disease in patients undergoing any revascularization procedure is one of the strongest predictors of poor outcome.42–44 Temporary or permanent worsening of renal function is frequently seen in patients with chronic kidney disease and portends poor short-term and long-term outcomes. Despite the use of low osmolar nonionic contrast agents, an increased risk of in-hospital death or myocardial infarction and repeat revascularization procedures is significant after PCI.42,45
Perhaps the most disheartening long-term adverse outcome is the development of restenosis at the PTCA site. Although a detailed discussion of the biology of restenosis and the many pharmacologic trials designed to decrease the incidence of restenosis are beyond the scope of this chapter, to understand better the importance and timing of the “stent era,” a brief review of the restenosis process is in order. Histopathologic studies in the early years of PTCA suggested a “response to injury” as a mechanism for the benefit of the procedure and the risk of restenosis.46–48 Developments in molecular and cell biology provided further insight into the complex cellular, autocrine, and paracrine responses to vascular disruption.49,50 Although some degree of intimal hyperplasia and lumen compromise is noted in all patients after PTCA,51,52 the final common pathway for patients with restenosis is the development of excessive neointimal hyperplasia. The time course for this process in humans is similar to wound healing. With the incidence of restenosis after conventional PTCA ranging from 30% to 50%, and the failure of specific devices or pharmacologic therapies to reduce the risk of restenosis, the stage was set for the stent era.
STENT ERA
As with balloon angioplasty, much of the seminal work in coronary stent design and materials technology was done in collaboration with vascular and interventional radiologists. Although the first coronary stent technology applied in humans was designed to treat occlusive dissections (Fig. 29-18) after PTCA,53,54 it was not until the pivotal STRESS55 and BENESTENT56 randomized controlled trials showed a reduction in the incidence of restenosis compared with PTCA that the use of coronary stents exponentially increased. Although the risk of restenosis was reduced with stents, the main driver for the rapid acceptance of this technology was the predictable final angiographic result (Fig. 29-19) and the diminished risk of abrupt vessel closure. As with all new technologies, however, important limitations to this addition to the interventional cardiologists’ armamentarium rapidly became apparent. The first limitation, relating to the complex and prolonged in-hospital anticoagulation and antithrombotic regimen required after the placement of a coronary stent, was ultimately addressed with the use of high-pressure deployment techniques to optimize stent expansion and apposition to the vessel wall and thienopyridine derivatives for platelet antagonism.57–59
The second limitation to the use of metallic stents was not only the persistence of the restenosis problem, albeit of lessened absolute magnitude though still of clinical consequence, but also the high rate of failure of conventional means of treating in-stent restenosis (Fig. 29-20). Another rapid period of innovative catheter-based technologies ensued, each with its own limitations and, importantly, each characterized by persistently unacceptable rates of recurrent in-stent restenosis. In the mid-1990s, the introduction of vascular brachytherapy for the treatment of in-stent restenosis offered some promise,60,61 although the technique was logistically complex and labor intensive.
Coincident with the declining enthusiasm for vascular brachytherapy for the treatment of patients with in-stent restenosis was the introduction of drug-eluting stents. Early reports from small series of selected patients documenting essentially zero restenosis after implantation of a sirolimus-eluting stent were met with considerable relief and enthusiasm.62 In short order, the pivotal randomized trials with sirolimus-eluting stents63 and paclitaxel-eluting stents64 showed short-term safety and efficacy with virtually single-digit restenosis rates. The rapid acceptance of this revolutionary technology led to the increasing realization, however, that the inhibition of the normal vascular healing process might increase the risk of inadequate re-endothelialization with an increased risk of stent thrombosis.65,66 Results from numerous nonrandomized studies and uncontrolled case series suggested an increased risk compared with bare-metal stents, although the magnitude of this risk was believed to be small and the time course delayed.67 These same studies failed to identify an increased risk of death and myocardial infarction, which generally accompany stent thrombosis. Larger and longer term studies continue to note a slightly increased risk of late (>1 year) stent thrombosis without an increased risk of death or myocardial infarction.
Current recommendations for postprocedural management call for extended dual antiplatelet therapy (thienopyridine plus aspirin) for 1 year after implantation of a drug-eluting stent68 and, of equal importance, careful consideration of the relative merits of a drug-eluting stent compared with a bare-metal stent in each individual case.69
Major adverse periprocedural events, most of which are associated with adverse clinical events and some of which are interrelated, are significant coronary dissection, coronary perforation, thromboembolization, and abrupt vessel closure. Examples of each are shown in Figures 29-21 through 29-23. In the present era of very low profile balloon catheters, potent antithrombotic agents, near-universal use of stents, and increased operator experience, the incidences of these undesirable events have decreased individually to less than 1%.
CATHETER-BASED MANAGEMENT OF VALVULAR HEART DISEASE
Beginning with the dramatic hemodynamic and clinical improvement in patients with congenital pulmonic valve stenosis,70 the era of nonsurgical management of congenital and acquired valvular heart disease began. Because the management of patients with congenital heart disease is discussed elsewhere, the current discussion focuses on catheter-based management of adults with acquired valvular heart disease.
Balloon catheter valvotomy was first applied to patients with rheumatic heart disease—most commonly mitral stenosis71—and has now become the preferred treatment for such patients in the absence of specific contraindications.72 In properly selected patients and with experienced operators, the results are generally highly predictable (Fig. 29-24) with low complication rates.
Balloon catheter valvotomy in adult patients with calcific aortic valve stenosis was first reported in 1985 by the group from Rouen.73 An immediate reduction in gradient and an increase in aortic valve area (Fig. 29-25) were thought to connote procedural success and, it was hoped, longer term overall clinical improvement. Mid-term to long-term follow-up indicated a high incidence of “restenosis,” however, and a persistently elevated increased risk of death.74 The current role of aortic valvuloplasty in the management of elderly patients or patients at high risk for surgical aortic valve replacement is unclear because there are no randomized trials to assess comparative outcomes. Nevertheless, aortic valvuloplasty represents a reasonable palliative option for “end-stage” patients at increased surgical risk or increased risk from progressive heart failure. In such patients, the procedure may be viewed as a “bridge” to surgical valve replacement, or as a compassionate procedure with clearly prespecified expectations. The emerging era of percutaneous catheter-based valve replacement (see next) may have a significant impact on treatment options for such patients.
In adults, balloon pulmonic valvotomy is generally performed in patients who are symptomatic or in patients who have progressed through adolescence and early adulthood without signs or symptoms of hemodynamic deterioration, but in whom critical values for right ventricular pressure and transvalvular gradient are documented.72 Rarely, the procedure may be successfully performed on a noncongenitally stenosed pulmonic valve as well (Fig. 29-26). Data in the literature on longer term outcomes after native pulmonic valvuloplasty in adults are scarce.75
Finally, rapid developments in stent and catheter technology now allow for “hybrid devices” (balloon/stent/ancillary device) to be used in the management of stenotic or insufficient valves. Beginning with the nonsurgical replacement of malfunctioning pulmonic valves,76 the concept of stent-mounted valves has attracted great interest. Although this field is in its infancy,77 early reports from ongoing clinical trials for mitral valve repair and aortic valve replacement are encouraging.78
Babaliaros V, Block P. State of the art percutaneous intervention for the treatment of valvular heart disease: a review of the current technologies and ongoing research in the field of percutaneous valve replacement and repair. Cardiology. 2007;107:87-96.
Bravata DM, Gienger AL, McDonald KM, et al. Systematic review: the comparative effectiveness of percutaneous coronary interventions and coronary artery bypass graft surgery. Ann Intern Med. 2007;147:703-716.
Gunn J, Crossman D, Grech ED, et al. New developments in percutaneous coronary intervention. BMJ. 2003;327:150-153.
Holmes DRJr, Kereiakes DJ, Laskey WK, et al. Thrombosis and drug-eluting stents: an objective appraisal. J Am Coll Cardiol. 2007;50:109-118.
Kereiakes DJ. Effects of GP IIb/IIIa inhibitors on vascular inflammation, coronary microcirculation, and platelet function. Rev Cardiovasc Med. 2006;7(Suppl 4):S3-S11.
Nallamothu BK, Bradley EH, Krumholz HM. Time to treatment in primary percutaneous coronary intervention. N Engl J Med. 2007;357:1631-1638.
Rajagopal V, Kapadia SR, Tuzcu EM. Advances in the percutaneous treatment of aortic and mitral valve disease. Min Cardioangiol. 2007;55:83-94.
Routledge HC, Lefevre T, Morice MC, et al. Percutaneous aortic valve replacement: new hope for inoperable and high-risk patients. J Invasive Cardiol. 2007;19:478-483.
Sarkar K, Sharma SK, Sachdeva R, et al. Coronary artery restenosis: vascular biology and emerging therapeutic strategies. Expert Rev Cardiovasc Ther. 2006;4:543-556.
Soon KH, Selvanayagam JB, Cox N, et al. Percutaneous revascularization of chronic total occlusions: review of the role of invasive and non-invasive imaging modalities. Int J Cardiol. 2007;116:1-6.
1 Dotter CT, Judkins MP. Transluminal treatment of arteriosclerotic obstruction: description of a new technique and preliminary report of its application. Circulation. 1964;30:654.
2 Gruentzig AR, Senning A, Siegenthaler WE. Non-operative dilatation of coronary artery stenosis: percutaneous transluminal coronary angioplasty. N Engl J Med. 1979;301:61.
3 Stertzer SH, Myler RK, Bruno MS, et al. Transluminal coronary artery dilation. Pract Cardiol. 1979;5:25.
4 Myler RK, Gruentzig AR, Stertzer SH. Coronary angioplasty. In: Rapaport E, editor. Cardiology Update. New York: Elsevier Biomedical; 1983:1-66.
5 King SB, Yeh W, Holubkov R, et al. Balloon angioplasty versus new device intervention: clinical outcomes. A comparison of the NHLBI PTCA and NACI Registries. J Am Coll Cardiol. 1998;31:558-566.
6 Waller BF. “Crackers, breakers, stretchers, drillers, scrapers, shavers, burners, welders and melters”—the future treatment of atherosclerotic coronary artery disease? A clinico-morphologic assessment. J Am Coll Cardiol. 1989;13:969.
7 Iakovou I, Ge L, Colombo A. Contemporary stent treatment of coronary bifurcations. J Am Coll Cardiol. 2005;46:1446-1555.
8 Assali AR, Assa HV, Ben-Dor I, et al. Drug-eluting stents in bifurcation lesions: to stent one branch or both? Catheter Cardiovasc Interv. 2006;68:891-896.
9 Al Suwadi J, Yeh W, Cohen HA, et al. Immediate and one year outcome in patients with coronary bifurcation lesions in the modern era. Am J Cardiol. 2001;87:1139-1144.
10 Topol EJ, Yadav JS. Recognition of the importance of embolization in atherosclerotic vascular disease. Circulation. 2000;101:570-580.
11 Baim DS, Wahr D, George B, et alon behalf of the SAFER trial investigators. Randomized trial of a distal embolic protection device during percutaneous intervention of saphenous vein aorto-coronary bypass grafts. Circulation. 2002;105:1285-1290.
12 Aziz S, Ramsdale DR. Chronic total occlusions—a stiff challenge requiring a major breakthrough: is there light at the end of the tunnel? Heart. 2005;91(Suppl 3):iii-42-iii-48.
13 Stone GW, Ellis SG, Cannon L, et alTAXUS V investigators. Comparison of a polymer-based paclitaxel-eluting stent with a bare metal stent in patients with complex coronary artery disease: a randomized controlled trial. JAMA. 2005;294:1215-1223.
14 Smith SC, Feldman TE, Hirshfeld JWJr, et al. ACC/AHA/SCAI 2005 guideline update for percutaneous coronary intervention—summary article: a report of the American College of Cardiology/American Heart Association Task Force on Practice Guidelines (ACC/AHA/SCAI Writing Committee to Update the 2001 Guidelines for Percutaneous Coronary Intervention). Circulation. 2006;113:156-175.
15 Seung KB, Park D-W, Kim Y-H, et al. Stents versus coronary artery bypass grafting for left main coronary artery disease. N Engl J Med. 2008;358:1781-1792.
16 Buszman PE, Kiesz SR, Bochenek A. Acute and late outcomes of unprotected left main stenting in comparison with surgical revascularization. J Am Coll Cardiol. 2008;51:538-545.
17 Keeley EC, Boura JA, Grines CL. Primary angioplasty versus intravenous thrombolytic therapy for acute myocardial infarction: a quantitative review of 23 randomised trials. Lancet. 2003;361:13-20.
18 Svilaas T, Vlaar PJ, van der Horst IC, et al. Thrombus aspiration during primary percutaneous coronary intervention. N Engl J Med. 2008;358:557-567.
19 Bertrand ME, Van Belle E. Rotational atherectomy. In: Topol EJ, editor. Textbook of Interventional Cardiology. Philadelphia: Saunders; 2003:549-557.
20 Reifart N, Vandormael M, Krajcar M, et al. Randomized comparison of angioplasty of complex coronary lesions at a single center. Excimer Laser, Rotational Atherectomy, Balloon Angioplasty Comparison (ERBAC) study. Circulation. 1997;96:91-98.
21 Popma JJ, Lansky AJ, Purkayastha DD, et al. Angiographic and clinical outcome after cutting balloon angioplasty. J Interv Cardiol. 1996;8(Suppl):12A-19A.
22 Safian RD. Coronary atherectomy: directional and extraction techniques. In: Topol EJ, editor. Textbook of Interventional Cardiology. Philadelphia: Saunders; 2003:523-548.
23 Topol E, Leya F, Pinkerton C, et al. A comparison of directional atherectomy with coronary angioplasty in patients with coronary artery disease. N Engl J Med. 1993;321:221-227.
24 King SB3rd, Smith SCJr, Hirshfeld JWJr, et al. 2007 focused update of the ACC/AHA/SCAI 2005 guideline update for percutaneous coronary intervention: a report of the American College of Cardiology/American Heart Association Task Force on Practice guidelines. J Am Coll Cardiol. 2008;51:172-209.
25 Smith SCJr, Feldman TE, Hirshfeld JWJr, et alAmerican College of Cardiology/American Heart Association Task Force on Practice GuidelinesACC/AHA/SCAI Writing Committee to Update 2001 Guidelines for Percutaneous Coronary Intervention. ACC/AHA/SCAI 2005 guideline update for percutaneous coronary intervention: a report of the American College of Cardiology/American Heart Association Task Force on Practice Guidelines. Circulation. 2006;113:e166-e286.
26 Detre K, Holubkov R, Kelsey S, et al. Percutaneous transluminal coronary angioplasty in 1985-1986 and 1977-1981. N Engl J Med. 1988;318:265.
27 Ryan T, Faxon DP, Gunnar RP, et al. Guidelines for percutaneous transluminal coronary angioplasty. Circulation. 1988;78:486.
28 Ellis SG, Guetta V, Miller D, et al. Relation between lesion characteristics and risk with percutaneous intervention in the stent and glycoprotein IIb/IIIa era: an analysis of results from 10,907 lesions and proposal for new classification scheme. Circulation. 1999;100:1971-1976.
29 Williams DO, Holubkov R, Yeh W, et al. Percutaneous coronary intervention in the current era compared with 1985-1986: the National Heart, Lung and Blood Institute registries. Circulation. 2000;102:2945-2951.
30 Kimmel SE, Localio AR, Krone RJ, et al. The effects of contemporary use of coronary stents on in-hospital mortality. Registry Committee of the Society for Cardiac Angiography and Interventions. J Am Coll Cardiol. 2001;37:499-504.
31 Kimmel SE, Berlin JA, Strom BL, et alfor the Registry Committee of the Society for Cardiac Angiography and Interventions. Development and validation of a simplified predictive index for major complications in contemporary percutaneous transluminal coronary angioplasty practice. J Am Coll Cardiol. 1995;26:931-938.
32 Block PB, Peterson EC, Krone R, et al. Identification of variables needed to risk adjust outcomes of coronary interventions: evidence-based guidelines for efficient data collection. J Am Coll Cardiol. 1998;32:275-282.
33 Wennberg DE, Makenka DJ, Sengupta A, et al. Percutaneous transluminal coronary angioplasty in the elderly: epidemiology, clinical risk factors, and in-hospital outcomes. The Northern New England Cardiovascular Disease Study Group. Am Heart J. 1999;137:639-645.
34 The BARI Investigators. Influence of diabetes on 5-year mortality and morbidity in a randomized trial comparing CABG and PTCA in patients with multivessel disease: the Bypass Angioplasty Revascularization Investigation. Circulation. 1997;96:1761-1769.
35 Marroquin OC, Selzer F, Mulukutla SR, et al. A comparison of bare-metal and drug-eluting stents for off-label indications. N Engl J Med. 2008;358:342-353.
36 Babapulle MN, Joseph L, Belisle P, et al. A hierarchical Bayesian meta-analysis of randomised clinical trials of drug-eluting stents. Lancet. 2004;364:583-591.
37 Singh M, Lennon RJ, Holmes DRJr, et al. Correlates of procedural complications and a simple integer risk score for percutaneous coronary intervention. J Am Coll Cardiol. 2002;40:387-393.
38 Keelan PC, Johnston JM, Koru-Sengul T, et al. Comparison of in-hospital and one-year outcomes in patients with left ventricular ejection fractions ≤40-49% and ≥50% having percutaneous coronary revascularization. Am J Cardiol. 2003;91:1168-1172.
39 Aragon J, Lee MS, Kar S, et al. Percutaneous left ventricular assist device: “TandemHeart” for high-risk coronary intervention. Catheter Cardiovasc Interv. 2005;65:346-352.
40 Henriques JP, Remmelink M, Baan JJr, et al. Safety and feasibility of elective high-risk percutaneous coronary intervention procedures with left ventricular support of the Impella Recover LP 2.5. Am J Cardiol. 2006;97:990-992.
41 Vogel RA, Shawl F, Tommaso C, et al. Initial report of the National Registry of Elective Cardiopulmonary Bypass Supported Coronary Angioplasty. J Am Coll Cardiol. 1990;15:23-29.
42 Best PJ, Lennon R, Ting HH, et al. The impact of renal insufficiency on clinical outcomes in patients undergoing percutaneous coronary interventions. J Am Coll Cardiol. 2002;39:1113-1119.
43 Naidu SS, Selzer F, Jacobs A, et al. Renal insufficiency is an independent predictor of mortality after percutaneous coronary intervention. Am J Cardiol. 2003;92:1160-1164.
44 Sadeghi HM, Stone GW, Grines CL, et al. Impact of renal insufficiency in patients undergoing primary angioplasty for acute myocardial infarction. Circulation. 2003;108:2769-2775.
45 Rihal CS, Textor SC, Grill DE, et al. Incidence and prognostic importance of acute renal failure after percutaneous coronary intervention. Circulation. 2000;105:2259-2264.
46 Block PC, Myler RK, Stertzer S, et al. Morphology after transluminal angioplasty in human beings. N Engl J Med. 1981;305:382-385.
47 Block PC. Mechanism of transluminal angioplasty. Am J Cardiol. 1984;53:69C-71C.
48 Schwartz RS. Animal models of human coronary restenosis. In: Topol EJ, editor. Textbook of Interventional Cardiology. Philadelphia: Saunders; 2003:391-414.
49 Forrester JS, Fishbein M, Helfant R, et al. A paradigm for restenosis based on cell biology: clues for the development of new preventive therapies. J Am Coll Cardiol. 1991;17:758.
50 Libby P, Simon DI, Rogers C. Inflammation and arterial injury. In: Topol EJ, editor. Textbook of Interventional Cardiology. Philadelphia: Saunders; 2003:381-389.
51 Nobuyoshi M, Kimura T, Nosaka H, et al. Restenosis after successful percutaneous coronary transluminal coronary angioplasty: serial angiographic follow-up of 229 patients. J Am Coll Cardiol. 1988;12:616.
52 Kuntz RE, Gibson M, Nobuyoshi M, et al. Generalized model of restenosis after conventional balloon angioplasty, stenting and directional atherectomy. J Am Coll Cardiol. 1993;21:15.
53 Sigwart U, Puel J, Mirkovitch V, et al. Intravascular stents to prevent occlusion and restenosis after transluminal angioplasty. N Engl J Med. 1987;316:701-706.
54 Roubin GS, Cannon AD, Agarwal SK, et al. Intracoronary stenting for acute and threatened closure complicating percutaneous transluminal angioplasty. Circulation. 1992;85:916-927.
55 Fischman DL, Leon MB, Baim DS, et al. A randomized comparison of coronary stent placement and balloon angioplasty in the treatment of coronary disease. N Engl J Med. 1994;331:496-501.
56 Serruys PW, de Jaegere P, Kiemeneij F, et al. A comparison of balloon-expandable stent implantation with balloon angioplasty in patients with coronary artery disease. N Engl J Med. 1994;331:489-495.
57 Colombo A, Hall P, Nakamura S, et al. Intracoronary stenting without anticoagulation accomplished with intravascular ultrasound guidance. Circulation. 1995;91:1676-1688.
58 Schomig A, Neumann FJ, Kastrati A, et al. A randomized comparison of antiplatelet and anticoagulant therapy after the placement of coronary-artery stents. N Engl J Med. 1996;334:1084-1089.
59 Leon MB, Baim DS, Popma JJ, et al. A clinical trial comparing three antithrombotic drug regimens after coronary artery stenting. N Engl J Med. 1998;339:1665-1671.
60 Teirstein PS, Massullo V, Jani S, et al. Catheter-based radiotherapy to inhibit restenosis after coronary stenting. N Engl J Med. 1997;336:1697-1703.
61 Popma JJ, Suntharalingham M, Lansky AJ, et alfor the Stents and Radiation Therapy (START) investigators. Randomized trial of 90Sr/90Y(beta) radiation versus placebo control for treatment of in-stent restenosis. Circulation. 2002;106:1090-1096.
62 Morice MC, Serruys PW, Sousa JE, et al. Randomized study with the sirolimus-coated Bx velocity balloon-expandable stent in the treatment of patients with de novo native coronary artery lesions: a randomized comparison of a sirolimus-eluting stent with a standard stent for coronary revascularization. N Engl J Med. 2002;346:1773-1780.
63 Moses JW, Leon MB, Popma JJ, et al. Sirolimus-eluting stents vs. standard stents in patients with stenosis in a native coronary artery. N Engl J Med. 2003;349:1315-1323.
64 Stone GW, Ellis SG, Cox DA, et al. A polymer-based, paclitaxel-eluting stent in patients with coronary artery disease. N Engl J Med. 2004;350:221-231.
65 Holmes DRJr, Kereiakes DJ, Laskey WK, et al. Thrombosis and drug-eluting stents: an objective appraisal. J Am Coll Cardiol. 2007;50:109-118.
66 Maisel WH. Unanswered questions—drug-eluting stents and the risk of late thrombosis. N Engl J Med. 2007;356:981-984.
67 Laskey WK, Yancy CW, Maisel WH. Thrombosis in coronary drug-eluting stents: report from the meeting of the Circulatory System Medical Devices Advisory Panel of the Food and Drug Administration Center for Devices and Radiologic Health, December 7-8, 2006. Circulation. 2007;115:2352-2357.
68 Grines C, Bonow R, Casey DJr, et al. Prevention of premature discontinuation of dual antiplatelet therapy in patients with coronary artery stents. A science advisory from the American Heart Association, American College of Cardiology, Society for Cardiovascular Angiography and Interventions, American College of Surgeons and American Dental Association, with representation from the American College of Physicians. J Am Coll Cardiol. 2007;49:734-739.
69 Hodgson JMcB, Bottner RK, Klein LW, et alDrug-Eluting Stent Task Force. Final report and recommendations of the working committees on cost effectiveness/economics, access to care and medico-legal issues. Cathet Cardiovasc Interv. 2004;62:1-17.
70 Kan JS, White RI, Mitchell SE, et al. Percutaneous balloon valvuloplasty: a new method for treatment of congenital pulmonary valve stenosis. N Engl J Med. 1982;307:540.
71 Inoue K, Owaki T, Nakamura T, et al. Clinical application of transvenous mitral commissurotomy by a new balloon catheter. J Thorac Cardiovasc Surg. 1984;87:394-402.
72 Bonow RO, Carabello BA, Chatterjee K, et al. ACC/AHA 2006 guidelines for the management of patients with valvular heart disease: a report of the American College of Cardiology/American Heart Association Task Force on Practice Guidelines. Circulation. 2006;114:e84-e231.
73 Cribier A, Savin T, Saoudi N, et al. Percutaneous transluminal valvuloplasty of acquired aortic stenosis in elderly patients: an alternative to valve replacement? Lancet. 1986;1:63-67.
74 O’Neill WW, Mansfield Scientific Aortic Valvuloplasty Registry Investigators. Predictors of long-term survival after percutaneous aortic valvuloplasty: report of the Mansfield Scientific Aortic Valvuloplasty Registry. J Am Coll Cardiol. 1991;17:909-913.
75 Chen CR, Cheng TO, Huang T, et al. Percutaneous balloon valvuloplasty for pulmonic stenosis in adolescents and adults. N Engl J Med. 1966;335:21-25.
76 Bonhoeffer P, Boudjemline Y, Saliba Z, et al. Percutaneous replacement of pulmonary valve in a right ventricle to pulmonary artery prosthetic conduit with valve dysfunction. Lancet. 2000;356:1403-1405.
77 Vassiliades TA, Block PC, Cohn LH, et al. The clinical development of percutaneous heart valve technology. A position statement of the Society for Thoracic Surgeons, the American Association for Thoracic Surgery and the Society for Cardiovascular Angiography and Interventions. J Thorac Cardiovasc Surg. 2005;129:970-976.
78 Rosengart TK, Feldman T, Borger MA, et al. Percutaneous and minimally-invasive valve procedures: a scientific statement from the American Heart Association Council on Cardiovascular Surgery and Anesthesia, Council on Clinical Cardiology, Functional Genomics and Translational Biology Interdisciplinary Working Group and Quality of Care and Outcomes Research Interdisciplinary Working Group. Circulation. 2008;117:1750-1767.