20 Pelvis
Pelvic Anatomy
The pelvis is an osseous ring supported by musculature and connective tissue and contains reproductive organs, the lower urinary tract, parts of the small bowel, the rectosigmoid colon, and related blood vessels, nerves, and lymphatics. Fig. 20-1 illustrates the normal pelvic anatomy as seen on axial CT images.
An imaginary oblique line passing from the sacral promontory to the superior aspect of the symphysis pubis divides the pelvis into the false or greater pelvis superiorly and the true or lesser pelvis inferiorly. The false pelvis is related to the abdominal cavity anteriorly and superiorly and contains most of the small bowel, parts of the colon, and the common iliac vessels. The true pelvis contains some small bowel, the rectum, the urinary bladder, and the reproductive organs. Levator ani muscles define the inferior border of the lesser pelvis and separate it from the perineum. The pelvic inlet is the superior opening of the lesser pelvis, while the pelvic outlet is the inferior opening.1
The peritoneal reflections within the pelvis form several important recesses that are important in treatment planning, because these recesses may harbor fluid collections or metastases. The rectovesical space is the most caudal portion of the peritoneal cavity and is formed by the peritoneal reflection between the rectum and the urinary bladder. In males, the layers of peritoneum lining the rectovesical space are fused inferiorly to form Denonvilliers fascia, which lies between the prostate and the rectum. However, in females, the rectovesical space is divided by the indentation of the uterus into a vesicouterine recess anteriorly and a rectouterine cul-de-sac (pouch of Douglas) posteriorly. In females, the inferior portion of the peritoneum lining the rectouterine recess is fused and forms the rectovaginal septum. The pararectal fossae are lateral extensions of the rectovesical space. Indentation of the urinary bladder to the pelvic peritoneum causes formation of the supravesical space cranially and paravesical spaces laterally on each side.1
The prevesical space, also known as the space of Retzius, is the largest extraperitoneal recess in the pelvis and lies between the anterior abdominal wall anteriorly and the umbilicovesical fascia posteriorly. It extends cranially to the level of the umbilicus and its caudal boundary is formed by the puboprostatic ligament in the male and the pubovesical ligament in the female. The prevesical space is laterally related with the paravesical connective tissue. The perivesical space, which is bounded by the umbilicovesical fascia, contains the urinary bladder, obliterated umbilical arteries, and urachus running from the apex of the bladder to the umbilicus.1 The perineum is a diamond-shaped space located inferior to the pelvic floor muscles. The boundaries of the perineum are the symphysis pubis and the arcuate pubic ligament anteriorly; the inferior pubic ramus, ischial ramus, and ischial tuberosity laterally; and the coccyx posteriorly. The superficial transversus perinei muscle divides the perineum into two triangles: the urogenital triangle anteriorly and the anal triangle posteriorly. The urogenital triangle contains external urogenital organs and the anal triangle contains the anus.1
Blood Vessels
The common iliac bifurcation is located approximately at the level of the third to fourth lumbar vertebrae. The common iliac arteries run anterior to the common iliac veins. The common iliac arteries are divided into internal and external iliac arteries at the level of the fifth lumbar to first sacral vertebrae. Two branches of the external iliac artery that can be identified on imaging are the inferior epigastric artery and the deep circumflex iliac artery. The internal iliac arteries course posteroinferiorly and at the lower margin of the sacroiliac joints divide into anterior and posterior trunks.1
Pelvic Lymph Nodes
Lymphatics from pelvic organs drain to pelvic nodal chains bilaterally and to the retroperitoneum. Familiarity with the lymphatic drainage pathways is of great importance for staging pelvic tumors. Fig. 20-2 illustrates pelvic lymph node groups on axial CT images.
The most peripheral lymph nodes draining the pelvis are the inguinal nodes (see Fig. 20-2D). The inguinal lymph nodes are divided into superficial and deep groups. The superficial inguinal nodes drain the anus, the perianal skin, and the round ligament of the uterus. The lymph from the gluteal region and the anterior abdominal wall below the level of the umbilicus also drain to lateral nodes in this group. The medial group of superficial lymph nodes receives lymphatics from the perineal genitalia. The lower group of superficial inguinal lymph nodes receives superficial lymphatics from the lower extremity. The deep inguinal lymph nodes are located on the medial side of the femoral vein. They receive efferents from the deep lymphatics of the lower extremity, a small number of efferents from the superficial inguinal nodes, and lymphatic drainage from the glans penis or clitoris. The superficial and deep inguinal nodes drain to the external iliac lymph nodes.1
The external iliac lymph nodes accompany the external iliac vessels and are divided into medial, posterior, and lateral groups (see Fig. 20-2B and C). The medial group of the external iliac lymph nodes drains the urinary bladder, prostate, membranous part of the urethra, cervix, and upper part of the vagina. The posterior group receives lymphatics from the internal iliac nodes via the obturator lymph nodes. The lateral group drains lymph from the superficial and deep inguinal nodes. The external iliac lymph nodes drain to the posterior and lateral common iliac nodes.1
The internal iliac nodes accompany the internal iliac vessels and drain lymph from all of the pelvic viscera such as the body of the uterus, the prostate gland, the upper part of the vagina, the seminal vesicles, the vas deferens, the lower part of the ureters, and the bladder (see Fig. 20-2B). They also receive lymphatic drainage from the deeper parts of the perineum, the buttock muscles, and the posterior aspect of the thigh. They send efferents to the external iliac and common iliac chains. The sacral lymph nodes, which drain directly into lumbar lymphatics, and the anatomic obturator lymph nodes, which are occasionally present in the obturator canal, are members of the internal iliac group.1
The common iliac lymph nodes accompany the common iliac vessels and are divided into lateral, medial, and posterior groups (see Fig. 20-2A). The lateral group directly drains the external iliac lymph nodes. The posterior group receives efferents from both the internal and external iliac lymph nodes. The medial common iliac group receives lymph from the internal iliac nodes. The common iliac nodes drain into the left and right lateral aortic nodal chains, which are part of the lumbar nodes.1
The lumbar lymph nodes are divided into right lateral aortic, left lateral aortic, and preaortic lymph node groups. The right lateral aortic chain includes paracaval and retrocaval lymph nodes. The preaortic chain also includes precaval lymph nodes.1
Bones and Muscles
The pelvic skeleton is composed of two paired innominate bones, the sacrum, and the coccyx. The innominate bones are formed by three separate bones: the ilium, ischium, and pubis. The acetabulum, which is a socket for the head of the femur, is formed by the fusion of these three bones on each side laterally. The two innominate bones unite anteriorly at the symphysis pubis and posteriorly at the sacrum.1
The psoas muscles originate from the vertebral bodies and transverse processes of the 12th thoracic to 4th lumbar vertebrae. At the level of the first sacral vertebra, the psoas muscle unites with the iliacus muscle, which originates from the upper two thirds of the iliac fossa and sacral ala. Then, the iliopsoas muscle inserts on the lesser trochanter of the femur.1
The levator ani and coccygeus muscles are the main muscles of the pelvic diaphragm. The levator ani muscle originates from the pubis, ischium, and the fascia of the obturator internus muscle and inserts on the coccyx and the anococcygeal raphe. The coccygeus muscle arises from the ischial spine and attaches to the coccyx posterior to the levator ani. The other pelvic floor muscles are the deep and superficial transversalis perinei muscles, the ischiocavernosus and bulbocavernosus muscles, and the external anal sphincter muscle.1
The obturator internus muscle arising from the anterolateral wall of the true pelvis and the piriformis muscle arising from the lateral aspect of the sacrum insert on the greater trochanter of the femur and form part of the pelvis sidewall.1
Uterus and Cervix
The uterus is located posterior to the urinary bladder and anterior to the rectum (Fig. 20-3A-D). In women of reproductive age, the uterus is normally 6 to 9 cm in length. The uterine volume varies with the menstrual cycle and is greatest during the secretory phase.2
On T2-weighted magnetic resonance images, three distinct uterine layers can be differentiated: the endometrium, junctional zone, and myometrium. The endometrium is of high signal intensity on T2-weighted images and occupies the central portion of the uterus. The myometrium, which is of medium signal intensity on T2-weighted images, is separated from the endometrium by a low-signal-intensity region called the junctional zone. There is no histologic equivalent to the junctional zone. It just represents inner myometrium, which has lower water content than the outer myometrium (see Fig. 20-3A and C).2,3
In premenarchal females, the endometrium is either very thin or absent, and the junctional zone is indistinct. The myometrium has lower signal intensity than in postmenarchal females. In postmenopausal women, the zonal anatomy is again indistinct, the endometrium is less than 3 mm thick, and the myometrium is of lower signal intensity compared with that of premenopausal females.2
The parametrium is a cellular connective tissue contiguous with the bare areas of the uterus that are not covered by the peritoneum. The parametrium is found adjacent to the lateral margins of the uterus, where the peritoneum reflects to form the broad ligaments and anteriorly between the uterus and the bladder beneath the peritoneal reflection of the vesicouterine pouch. Parametrial tissue contains ureters, blood vessels, and lymphatics (see Fig. 20-3D).2,4,5
The broad ligaments of the uterus are formed by the reflections of the peritoneum as it passes over the fallopian tubes and enclose the fallopian tubes, round ligaments, uterine vessels, and ovarian ligaments. The round ligaments originate at the lateral angles of the uterus and pass through the inguinal canal to insert the labia majora. The suspensory ligaments of the uterus are the vesicouterine, cardinal, and uterosacral ligaments. The vesicouterine ligaments are located between the cervix and the urinary bladder base. The cardinal ligaments originate from the lateral pelvic wall, divide into anterior and posterior slips, and surround the cervix. The uterosacral ligaments pass between the cervix and sacrum and encircle the rectum. The uterine suspensory ligaments are clinically significant because they form local pathways for tumor spread, particularly in cervical carcinoma.2
The cervix is the inferior portion of the uterus (see Fig. 20-3A and D). On T2-weighted magnetic resonance images, the cervix demonstrates a high-signal-intensity inner area with a surrounding low-signal-intensity stroma. The inner zone represents epithelium and mucus. The stroma of the cervix, which has a high concentration of elastic fibrous tissue within its inner portion, demonstrates low signal intensity on T2-weighted images. Smooth muscle strands predominate toward the periphery of the cervix, resulting in an area of medium signal intensity similar to that of myometrium.2,4,5
Endometrial Cancer
Endometrial carcinoma is the fourth most common cancer in females and the most common invasive gynecologic malignancy in the United States.6 Up to 90% of endometrial cancers are adenocarcinomas. Although the exact causal factors of endometrial cancer are still unknown, two distinct mechanisms may play a role in its origin: (1) unopposed estrogen stimulation, causing endometrial hyperplasia, which then progresses to carcinoma; and (2) spontaneous carcinoma arising from atrophic or inert endometrium.7
The most important prognostic factors in endometrial cancer include the histologic grade of the tumor, the stage of the tumor, depth of myometrial invasion, and the lymph node status.8 Because of the inability of clinical staging to assess the depth of myometrial invasion or the presence of lymphadenopathy, the International Federation of Gynecology and Obstetrics (FIGO) revised the staging of endometrial cancer to incorporate surgical findings. Surgical staging, however, is not suitable for elderly and obese patients and those with additional medical problems that make them poor candidates for surgery. Noninvasive cross-sectional imaging is particularly helpful in such cases to depict the depth of myometrial invasion, tumor extent, and presence of lymphadenopathy. Pretreatment imaging improves patient care by affecting the type and extent of surgery or radiation treatment or both.3
Ultrasound is a simple technique that can depict endometrial tumors (Fig. 20-4). The use of transabdominal ultrasonography in staging of endometrial cancer is not advocated. However, transvaginal ultrasonography is helpful in the evaluation of myometrial invasion, with accuracy rates varying between 68% and 99%.3 Myometrial invasion is suggested when the tumor disrupts the subendometrial halo and extends asymmetrically into myometrium.9 The limitations of ultrasound include: (1) poor soft-tissue contrast resolution, which hampers delineation between tumor, adjacent myometrium, and coexisting pathologic conditions such as uterine leiomyomas; (2) a relatively small field of view, which precludes assessment of the cervix, parametrium, and the lymph nodes, especially in patients with large tumors or large body habitus or both.3
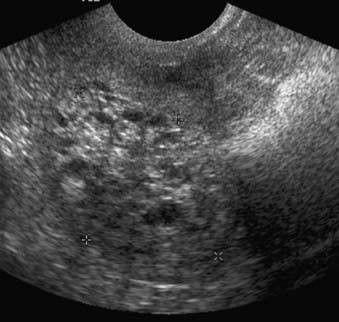
FIGURE 20-4 • Transvaginal ultrasound depicts large heterogeneous mass containing cystic areas within the endometrial cavity.
CT is widely used for the preoperative evaluation of endometrial carcinoma to assess the extent of disease and lymph node status.3 The accuracy of preoperative staging with conventional CT for endometrial cancer varies between 84% and 88%.10,11 However, even when helical CT was used, magnetic resonance imaging (MRI) was reported to be more sensitive and specific than CT for preoperative staging of endometrial cancer.12
At present, magnetic resonance is the most accurate imaging modality for the pretreatment evaluation of endometrial cancer. The reported staging accuracy of MRI for endometrial cancer is between 83% and 92%.13–15 A suggested MRI protocol includes orthogonal T2-weighted; transverse T1-weighted; and sagittal, dynamic, contrast-enhanced, T1-weighted sequences.3 This protocol is optimal for detection of primary tumor, myometrial and cervical involvement, local spread, and lymphadenopathy.3 Current National Comprehensive Cancer Network (NCCN) guidelines do not suggest a role for fluorodeoxyglucose (FDG)-PET in the management of endometrial cancer.16 Yet FDG-PET appears highly-sensitive for the detection of metastatic endometrial cancer.17 When added to MRI or CT, FDG-PET may improve detection of malignant lymphadenopathy18,19 and visceral20 metastases, changing clinical management in approximately one third to one half of patients.19,21 FDG-PET combined with CT appears to be more reliable than FDG-PET alone for detecting adenopathy.18,20,22,23 Evaluation of the primary tumor by FDG-PET is limited, as FDG-PET cannot precisely determine the extent of tumor invasion, and MRI is clearly superior for that purpose. Endometrial tumors are isointense to myometrium on T1-weighted sequences and demonstrate a variable appearance on T2-weighted sequences (Fig. 20-5). Following dynamic administration of contrast, they enhance less than the normal myometrium, which is more marked on early phases.3 Stage 0 (carcinoma in situ) tumors appear as normal or widened endometrium. Stage I endometrial cancers are confined to the uterine corpus. In stage IA, the tumor is limited to endometrium and may demonstrate normal, diffuse, or focal abnormal signal intensity with a smooth endometrial-myometrial interface. Stage IB tumors penetrate into myometrium less than 50% of myometrial thickness with partial or full-thickness disruption of the junctional zone and an irregular endometrial-myometrial interface. In stage IC, the tumor extends into more than 50% of the myometrial thickness; however, the outer stripe of myometrium is intact. Stage II endometrial cancers extend beyond the uterine corpus into the cervix. In stage IIA, the internal os and endocervical canal are widened; however, the low-signal-intensity, fibrous cervical stroma is intact. In stage IIB, fibrocervical stroma is disrupted. Stage III endometrial cancers extend beyond the uterus but not to the true pelvis. In stage IIIA, the outer myometrium is irregular and disrupted. The ovaries may be involved by direct extension or as metastases. Parametrial involvement is seen as disruption of the serosa and direct tumor extension into parametrial fat. Stage IIIB tumors involve the upper vagina. Tumor involvement of the vagina is seen as disruption of the low-signal-intensity vaginal wall. In stage IIIC disease, there is lymph node involvement, which can be diagnosed when the short axis of lymph nodes exceeds 1 cm. Unfortunately, metastatic and hyperplastic lymphadenopathy cannot be distinguished based on signal intensity characteristics. Stage IV tumors extend beyond the true pelvis. In stage IVA there is disruption of tissue planes with loss of the low-signal-intensity of the bladder wall or the rectal wall, or both, indicating invasion of these organs. In stage IVB, there is distant organ metastasis.3
Cervical Cancer
Cervical cancer occurs more commonly in young women with low socioeconomic status. Risk factors include early age at first intercourse, several sexual partners, nulliparity, smoking, and a history of sexually transmitted diseases. There is strong evidence that the human papilloma virus is a main cause of cervical cancer. Cervical cancer arises at the squamocolumnar junction. The two main histologic types are squamous cell carcinoma, which accounts for 80% to 90% of cases, and adenocarcinoma, which has a worse prognosis. Tumor prognostic factors include the histologic grade of the tumor, the location of the tumor (exocervix versus endocervix), tumor volume, depth of stromal invasion, adjacent organ invasion, and lymph node status.5
The FIGO staging system includes findings obtained from physical examination, examination under anesthesia, cystoscopy, rectosigmoidoscopy, barium enema, biopsy, intravenous pyelography, and chest radiography. There is a discrepancy between clinical staging and intraoperative or pathologic findings in 20% to 35% of cases depending on the stage of the tumor.5,24–26 In addition, lymph node metastases and extension into adjacent organs are difficult to detect clinically.
Ultrasound has a low diagnostic value in cervical cancer staging. The patient’s habitus, lack of soft-tissue contrast, and operator dependence are limitations of ultrasound in the staging of pelvic malignancies.5 CT cannot differentiate normal cervical tissue from tumor and is therefore also limited for evaluating tumor size and stromal invasion5 (Fig. 20-6). In the staging of cervical carcinoma, CT was found to be 65% accurate and MRI was found to be 90% accurate. In evaluating lymph node metastasis, both modalities had an accuracy of 86%.27 For detection of metastatic adenopathy, FDG-PET has been found more sensitive than CT28 or MRI,29,30 with equivalent or superior specificity (approximately 95% in all stages).31,32 FDG-PET is recognized as superior to CT for the detection of abdominal paraaortic lymph node metastases in either early-stage or advanced cervical cancer.28,31 Paraaortic lymph node metastases carry an adverse prognostic implication, necessitating detection of these sites of disease.33,34 The superiority of FDG-PET for evaluation of paraaortic lymph node metastases has led to its use in the planning of IMRT for cervical cancer,35,36 and FDG-PET has been used in planning doses to the primary tumor as well.37,38 The initial clinical experience suggests that FDG-PET–guided radiotherapy improves and increases tumor irradiation without increasing doses to adjacent organs.38 No large studies of FDG-PET/CT have been reported in presurgical staging of cervical cancer—only FDG-PET has been studied; FDG-PET/CT is expected to be more sensitive and specific than FDG-PET alone, as it has proven in other types of cancer.23 Possibly, PET/CT findings may be able to limit the extent of surgical lymph node sampling, although the assay is unlikely to be sufficiently sensitive to obviate surgical lymph node staging altogether.29 In a retrospective study, Grigsby and colleagues39 found that patients with pretreatment FDG-PET who showed no evidence of metastases received no benefit from adjuvant chemotherapy as compared with pelvic radiotherapy alone. Whether pretreatment FDG-PET findings are a reliable basis for omitting adjuvant chemotherapy remains to be validated prospectively, in a randomized trial. FDG-PET appears very sensitive for detection of distant metastases, providing a potentially useful means of excluding patients from futile surgery.40 The NCCN recommends FDG-PET as part of the pretreatment assessment for patients with cervical cancer of clinical stage IB2 or higher.41 In disease of a lesser stage and as part of tumor surveillance, the NCCN recommends use of FDG-PET “as clinically-indicated”41 (Fig. 20-7). In the setting of initial staging or suspected recurrence, FDG-PET (or PET/CT) changes clinical management of cervical cancer patients in approximately 21% to 55% of cases.42–44
Cervical cancer appears as a relatively high-signal-intensity mass within low-signal-intensity cervical stroma on T2-weighted sequences (Fig. 20-8). The contrast enhancement of cervical cancer varies considerably. Because contrast enhancement makes the tumor isointense with the surrounding cervical and parametrial tissue on T1-weighted images, it does not increase the ability of MRI to depict the tumor or stromal or parametrial invasion.45–49 Contrast enhancement may help in the evaluation of tumor extension into the pelvic wall or adjacent organs.45
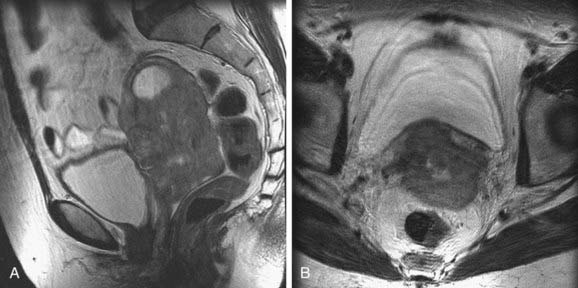
FIGURE 20-8 • Sagittal (A) and axial (B) T2-weighted magnetic resonance images demonstrate a large cervical mass growing into the uterus.
Stage 0 (carcinoma in situ) tumors are not visible on MRI. Stage I tumors are confined to the cervix. Depending on their size, these tumors may be identified by the high signal intensity they display within a low-signal-intensity cervical ring on T2-weighted images. The depth of stromal invasion can be determined easily. Preservation of a low-signal-intensity cervical stripe is a good indicator for the exclusion of parametrial invasion. Complete disruption of the cervical ring, indicating full-thickness stromal involvement, may hamper exclusion of parametrial involvement. Stage II tumors extend beyond the cervix. In stage IIA tumors, less than two thirds of the upper vagina is involved, which is seen as loss of normal low signal intensity and thickening of the vagina. The tumor is classified as stage IIB when there is parametrial invasion. The tumor extends directly through low-signal-intensity cervical stroma into the parametrium. Stage IIIA tumors extend into the lower third of the vagina, as indicated by the loss of low signal intensity and thickening of the lower part of the vagina. In stage IIIB tumors, there is pelvic side-wall invasion, and hydronephrosis can be seen if there is ureteral involvement. Stage IV tumors invade the bladder or rectum, as indicated by the loss of normal low signal intensity and thickening of the walls of these adjacent structures.45–49
Signal intensity characteristics are not helpful in differentiating between malignant and hyperplastic lymph nodes. MRI has a sensitivity of 62%, a specificity of 98%, and an accuracy of 93% in detecting metastatic lymphadenopathy in cervical cancer if the upper limit of normal lymph nodes is accepted as 1 cm in the short axis.50 MRI and CT are not significantly different in the detection of metastatic lymphadenopathy in cervical cancer.51 Schwarz and colleagues52 prospectively studied 92 cervical cancer patients, with stages ranging from IB1 to IVA, correlating clinical outcomes (progression-free and cause-specific survival) to FDG-PET/CT findings obtained 8 to 16 weeks after completion of treatment (brachytherapy, external beam radiotherapy, and chemotherapy). The post-treatment FDG-PET/CT findings were more predictive of outcomes during the 3- to 5-year follow-up period than was the pretreatment lymph node status. At the 8- to 16-week post-therapy point, patients with a complete response by FDG-PET (i.e., a resolution of all PET evidence of metastatic disease) had a 5-year cause-specific survival rate of 84%. Patients with progressive disease detected by FDG-PET (i.e., new metastatic sites) had a survival rate of 10%. Patients with a partial PET response (i.e., no new disease, with no resolution or partial resolution of the old disease) had a survival rate of 33%. These specific results cannot be generalized to other therapeutic regimens, of course, but they highlight the fact that in patients with cervical cancer (as in patients with numerous other types of cancer), FDG-PET is effective in predicting long-term response to therapy.53–58 The sensitivity and specificity of FDG-PET for the detection of recurrent cervical cancer appears to be similar in asymptomatic and symptomatic patients.59 Data from more than 600 patients, cumulatively, have been reported regarding FDG-PET and the detection of recurrent cervical cancer.42–44,59–61 In studies with 40 or more patients,43,44,59,61 FDG-PET has overall sensitivity ranging from 80% to100% and overall specificity ranging from 76% to 100% for the detection of recurrent cervical cancer.
Vagina and Vulva
On cross-sectional images, the vagina is located between the bladder and urethra anteriorly and the rectum and anal canal posteriorly (see Fig. 20-3A). The upper vagina is attached to the uterine cervix near the external os and forms anterior and posterior fornices. The posterior vaginal fornix is deeper than the anterior fornix.2,62
Cancer of the Vagina
Most of the primary vaginal malignancies are squamous cell carcinomas. Adenocarcinomas are only 5% to 10% of primary vaginal malignancies. Clear cell adenocarcinoma of the vagina is a subtype of adenocarcinoma associated with maternal ingestion of diethylstilbestrol during pregnancy. Vaginal melanomas and vaginal sarcomas are other rare primary vaginal malignancies. In children younger than 6 years of age, the most common primary vaginal malignancy is embryonal rhabdomyosarcoma (sarcoma botryoides). Metastases to the vagina are more common than primary vaginal tumors and usually occur by direct extension from adjacent organs such as the endometrium, cervix, rectum, or bladder.62
The most important prognostic factors in vaginal malignancies are tumor stage and tumor size. Staging of vaginal cancer according to FIGO includes clinical examination, chest x-ray examination, complete blood count, and the biochemical profile. Often cystoscopy, sigmoidoscopy, barium enema, and intravenous pyelography are also included in FIGO staging.62
Cross-sectional imaging has been increasingly used in the evaluation of patients with vaginal cancers. Ultrasound is limited in the evaluation of the cervix and vagina. Both ultrasound and CT have low soft-tissue contrast and are therefore not good enough in depicting early vaginal cancer. CT is useful in the evaluation of advanced disease and in the assessment of lymph node status. MRI has excellent soft tissue resolution and is therefore very useful in evaluating the tumor size, location, and extent of disease.62
On T2-weighted magnetic resonance images, vaginal carcinomas appear as intermediate- to high-signal-intensity masses. When the tumor is confined to the vaginal wall (stage I), the normal low-signal-intensity vaginal wall is intact on T2-weighted sequences. Stage II lesions invade paravaginal tissues but do not extend to the pelvic side wall. On T2-weighted sequences, the interface between fat and tumor is not well defined and there is medium to high signal intensity similar to tumor intensity within paravaginal tissues. In stage III tumors there is increased signal intensity within pelvic floor muscles on T2-weighted images, indicating extension into the pelvic wall. Stage IVA tumors invade the bladder or rectum or extend beyond the true pelvis. Direct tumor extension into the bladder or rectum can be seen as disruption of soft-tissue planes and increased signal intensity within the bladder or rectal wall.62
Little has been written about the use of FDG-PET in vaginal cancer. Vaginal cancer is an FDG-avid disease.63 FDG-PET appears to be more sensitive than conventional CT for detection of metastatic adenopathy.63 In a small study40 combining vaginal and cervical cancer patients, FDG-PET was found 100% sensitive for detection of extrapelvic metastases, thus posing FDG-PET as an attractive means for excluding patients from treatments futile in that setting (e.g., pelvic exenteration). One potential false-positive result worth noting is the presence of excreted FDG often found in the vaginal vault; small spots of contamination can mimic soft-tissue disease.
Cancer of the Vulva
Vulvar cancer is a rare malignancy occurring most commonly in women older than age 60. The most common histologic type is squamous cell carcinoma. The other vulvar cancers consist of melanoma, Bartholin gland cancer, Paget’s disease, sarcomas, basal cell carcinoma, and adenocarcinoma.62
Prognosis of vulvar carcinoma depends on tumor size, depth of tumor invasion, and lymph node metastases. CT is useful in the evaluation of advanced disease and lymph node status. MRI is superior to both ultrasound and CT in evaluating local extension of the tumor.62
Stage I vulvar cancers are less than 2 cm in size and can be seen as high-signal-intensity lesions on T2-weighted sequences. Stage II tumors are larger than 2 cm but confined to the vulva. In stage III tumors, there is invasion of the lower urethra with or without involvement of the vagina and anus. A high-signal-intensity mass extending into these structures can be seen on T2-weighted magnetic resonance images. In stage IVA, tumor extension to the upper urethra, bladder, rectal mucosa, or pelvic bones can be seen as areas of intermediate- to high-signal-intensity within these structures.62
Although, to date, no large studies have examined the usefulness of FDG-PET in cancer of the vulva, FDG-PET can provide useful information for treatment planning and post-treatment follow-up in patients with vulvar cancer (Fig. 20-9).
Ovaries
The ovaries lie in the lateral pelvic sidewall recesses called ovarian fossae, between the obliterated umbilical artery anteriorly and the internal iliac artery and the ureter posteriorly (see Fig. 20-3B).64
The size and appearance of the ovary varies with age. At birth the ovary measures 1.5 × 0.5 × 0.3 cm and is situated within the false pelvis. During adolescence the ovaries enlarge to reach adult size and move to the true pelvis. In the adult, the ovary measures approximately 3 × 1.5 × 1 cm and weighs 2 to 8 grams. After menopause, the ovary becomes atrophic, measuring less than 2 cm in diameter and weighing only 1 to 2 grams.64
The ovarian suspensory ligament extends from the lateral pelvic wall to the tubal aspect of the ovary and contains the ovarian artery and vein. The tubo-ovarian ligament originates from the lateral uterine angle and attaches to the uterine aspect of the ovary.64
The ovarian arteries originate directly from the aorta. A small ovarian branch of the uterine artery supplies the ovary in addition to the ovarian artery. The ovarian veins drain to the inferior vena cava on the right side and to the left renal vein on the left side.64
Ovarian Cancer
Ovarian cancer is the fifth most common cause of cancer-related deaths in women and the most common cause of death from gynecologic malignancy.6 Approximately 90% of ovarian cancers are of epithelial origin, with subtypes such as serous, mucinous, endometrioid, clear cell, and undifferentiated. Nonepithelial cancers of the ovary include malignant granulosa cell tumor, dysgerminoma, immature teratoma, endodermal sinus tumor, and metastases.65,66
The FIGO staging system of ovarian cancer reflects the three primary mechanisms of ovarian cancer spread: local, peritoneal, and lymphatic. Stage I ovarian cancer refers to tumor confined to one or both ovaries. Stage II indicates ovarian cancer with peritoneal metastases confined to the true pelvis. Stage III refers to ovarian cancer with extrapelvic peritoneal metastases or nodal disease. Stage IIIA indicates that there are microscopic implants. Stage IIIB refers to implants smaller than 2 cm and stage IIIC indicates that the implants are larger than 2 cm. Stage IV consists of ovarian cancer with distant metastases such as malignant pleural effusion and intrahepatic metastases. The management of ovarian cancer is closely related to stage. The standard of care for FIGO stage I, II, IIIA, and IIIB ovarian cancer is an exploratory staging laparotomy. The standard of care for resectable FIGO stage IIIC ovarian cancer is primary surgical cytoreduction (i.e., debulking) followed by adjuvant chemotherapy. Optimal debulking refers to the reduction of all tumor sites to a maximal diameter of less than 1 cm. The management of FIGO stage IV disease is primary chemo and cytoreduction. Considering different management options, the importance of imaging is in the distinction among FIGO stages IIIA, IIIB, and stage IIIC disease. Furthermore, in the category of FIGO stage IIIC disease, the importance of imaging is in the detection of nonresectable disease. The assignment of FIGO stage IV disease is also important for patient management. There are two potential pitfalls in assigning FIGO stage IV disease. On imaging, the presence of pleural effusion can be benign or malignant. The findings of pleural thickening or nodularity in addition to the presence of pleural effusion indicate the malignant nature of effusion. In the absence of those findings, thoracentesis is essential. The other important distinction is to differentiate liver surface implants (peritoneal spread and therefore stage III disease) from true intraparenchymal metastases (as a result of hematogenous spread and therefore stage IV disease).65,67 Current NCCN guidelines suggest use of FDG-PET “as clinically-indicated” for monitoring of patients with epithelial ovarian cancer (at any stage) that has demonstrated a complete response to treatment.68 Ovarian cancer is composed of several histologic subtypes, possibly with differing levels of FDG-avidity; however, in general, ovarian cancer is FDG-avid.69–71 FDG-PET and FDG-PET/CT appear superior to conventional CT for staging of newly-diagnosed ovarian cancer.69,72 For detection of recurrent disease, FDG-PET/CT and MRI appear to be grossly equivalent, overall, in sensitivity and specificity,73,74 although MRI is superior for detection of peritoneal recurrence after cytoreductive surgery.73 FDG-PET appears to be superior to CT75–77 for the detection of recurrent disease. These generalities remain to be confirmed by well-controlled prospective trials comparing the modalities. Recurrent disease is best detected by FDG-PET in the setting of a rising cancer antigen (CA)125 value (PET sensitivities reported are approximately 85%, whereas reported PET/CT sensitivities are approximately 95%).78–80 Only lesions smaller than 6 mm are regularly missed.81,82 Hence, FDG-PET/CT is capable of detecting “macroscopic” disease amenable to cytoreductive surgery.81 Rising tumor marker serum levels cannot distinguish local recurrence from spreading disease, so a clear rationale for tumor localization is present in that setting. The lesion-based accuracy of FDG-PET/CT in recurrent ovarian cancer seems superior to that of PET alone.83 Direct comparisons of FDG-PET/CT with CT or MRI in the setting of recurrent ovarian cancer have suffered from patient selection bias (for example, in some studies FDG-PET/CT was performed only in cases in which MRI or CT had already provided negative or equivocal results74,78,81,84) or have used PET techniques that are no longer state-of-the-art.85 Nevertheless, it appears that the addition of FDG-PET/CT to conventional imaging modalities frequently alters therapeutic management (>50% of cases, in some series) in patients with persistent disease or suspected recurrence, usually by detecting unresectable disease or unsuspected distant metastases.75,78,83,86
In patients with an adnexal mass, ultrasound is very accurate in the assessment of tumor location (e.g., differentiation of uterine from adnexal masses), as well as distinguishing between a benign and a malignant adnexal lesion (Fig. 20-10). In addition to the morphologic features of the mass depicted on gray-scale ultrasound, Doppler ultrasound findings are also very helpful.87,88 If ultrasound findings are inconclusive, MRI is recommended for further evaluation.89 The use of contrast media in MRI allows better characterization of solid nodules within a cystic lesion or the presence of necrosis within a solid lesion. In the characterization of adnexal masses, MRI also demonstrates high inter- and intraobserver agreement.90,91 CT is not commonly used in the characterization of adnexal masses. Characterization of an adnexal mass on CT relies on the depiction of morphologic features such as enhancing mural nodularity or heterogeneity and necrosis within the solid lesion (Fig. 20-11). The presence of ancillary findings such as ascites and peritoneal carcinomatosis are strong indicators of a malignant adnexal mass on ultrasound, CT, or MRI. For characterizing ovarian tumors, FDG-PET/CT appears at least equivalent to other modalities in sensitivity69,71 and possibly superior to them in specificity.69 The University of Ulm group found FDG-PET (not PET/CT) inferior.89,92,93 Yet the Ulm reports must be reviewed critically: no reference is made to patients’ menstrual cycles. and PET/CT is generally superior to PET alone in both sensitivity and specificity, especially in the pelvis.23,76,84 Normal ovaries can demonstrate prominent FDG uptake that is physiologic and variable,94 related to the menstrual cycle;94,95 hence, premenopausal patients with an adnexal mass must be scheduled for FDG-PET at an appropriate day in the cycle to avoid false-positive results; in general, the week before and after midcycle ought to be avoided.
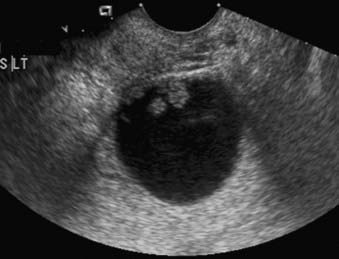
FIGURE 20-10 • Ovarian serous adenocarcinoma. Transvaginal ultrasound depicts a cystic ovarian mass with nodular solid components.
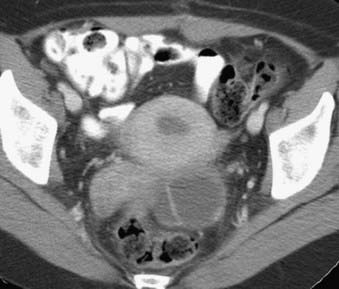
FIGURE 20-11 • Contrast-enhanced axial computed tomography reveals a left ovarian cystic mass with thick nodular wall.
Ovarian cancer can also spread to lymph nodes. Most commonly, nodal disease is seen along the aortocaval and paraortic retroperitoneal sites. Second, nodal spread along the broad ligament can reach the internal iliac, obturator, and external iliac vessels. Third, lymph node involvement can occur via the round ligament to the external iliac and inguinal nodes. Superior diaphragmatic lymphadenopathy is considered distant nodal disease and indicates advanced ovarian cancer. Extra-abdominal lymph node metastases, at presentation, have traditionally been thought to be rare in ovarian cancer based on clinical examination. Yet using FDG-PET/CT, Risum and colleagues96 found extra-abdominal nodal metastases in 37% of patients with newly diagnosed ovarian cancer.
FDG-PET has demonstrated a considerable ability to predict and monitor the response of ovarian cancer to therapy. For example, detection of large-bowel mesenteric implants by pretreatment FDG-PET/CT was an independent predictor of incomplete cytoreduction in a multivariate analysis (primarily of patients with stage IIIC disease).96 In a study of patients with stage IIIC and IV disease, reductions in tumor FDG uptake, induced by presurgical carboplatin-based chemotherapy, were predictive of significantly prolonged survival, whereas clinical response data (including CA125 response) and histopathologic tumor response data were not.97
Prostate and Seminal Vesicles
The normal adult prostate in a young man measures approximately 4 × 3 × 3 cm, and weighs 15 to 20 grams. It is shaped like an inverted pyramid and surrounds the prostatic urethra (Fig. 20-12).98,99 The prostate is histologically composed of glandular and nonglandular elements. The prostatic urethra and the anterior fibromuscular stroma are the major nonglandular components. The glandular portion of the prostate consists of inner and outer components, both of which are divided into two. The inner prostate consists of periurethral glandular tissue and the transition zone, and the outer prostate consists of the central and peripheral zones.98,99
The prostatic urethra is divided into proximal and distal parts by the verumontanum, which lies midway between the prostatic apex and the bladder neck. The proximal half of the prostatic urethra is surrounded by a cuff of smooth muscle known as the preprostatic sphincter, which prevents the passage of major ducts into the proximal part of the prostatic urethra.98,99
The periurethral glands and the transition and central zones are related exclusively to the proximal prostatic urethra. The peripheral zone surrounds all three components as well as the distal prostatic urethra. The ducts of the peripheral zone drain exclusively to the distal prostatic urethra.98,99
The zonal description of prostate anatomy is clinicopathologically more meaningful than the older lobar nomenclature. First, the zones are clearly defined on histologic sections. In addition, prostatic pathologic conditions have a zonal distribution. Of prostatic adenocarcinomas, 70% arise in the peripheral zone, 20% in the transition zone, and 10% in the central zone. In contrast, nodules of benign prostatic hyperplasia (BPH) arise either in the transition zone or the submucosal periurethral glands of the proximal urethra. Prostatitis is a disease of the glandular prostate, with a predilection for the peripheral zone.98–100
From an imaging viewpoint, the glandular prostate is arbitrarily divided into two components: the peripheral zone and the central gland. The term central gland refers collectively to the periurethral, transition, and central zones. In young men the central gland comprises mainly the central zone, while in older men with BPH, the central gland comprises mainly the transition zone.98–100
The true or anatomic capsule of the prostate is a 2- to 3-mm-thick fibromuscular tissue layer surrounding the prostate. The anatomic capsule should not be confused with the surgical or pseudocapsule, which develops around the central gland in the aging hyperplastic prostate.98,99 Anteriorly, the prostate is separated from the symphysis pubis and the pubic bones by vessels and connective tissue. The puboprostatic ligaments run from the prostatomembranous junction to the pubic bones. Posteriorly, the prostate is separated from the rectum by areolar tissue and Denonvilliers fascia. Posterosuperiorly, the seminal vesicles lie between the bladder base and rectum. Laterally, the prostate is surrounded by the periprostatic venous plexus and the muscles of the pelvic floor. The lateral venous plexus, arteries, and nerves collectively compose the neurovascular bundles, which pass craniocaudally along the posterolateral aspect of the prostate. The neurovascular bundles pierce the capsule; therefore, extracapsular extension of tumor occurs preferentially along them. Inferiorly, the apex of the prostate abuts the genitourinary membrane. The urethra exits the prostate to enter the genitourinary membrane and becomes the membranous urethra. The genitourinary membrane is suspended between the inferior pubic rami just below the symphysis pubis.98–100
The seminal vesicles are paired accessory glands located superior to the prostate gland, posterior to the urinary bladder, and anterior to the rectum. Each seminal vesicle consists of a single tube coiled on itself. In its medial portion, the tube is constricted and forms an excretory duct. The excretory duct of the seminal vesicle and the ductus deferens join to form an ejaculatory duct. The ejaculatory ducts pass obliquely within the prostate gland and at the verumontanum join to the prostatic urethra.98,101,102
Prostate Cancer
Prostate cancer is the most common cancer seen in men and the second most common cause of cancer-related deaths after lung cancer. It is estimated that 186,320 new cases of prostate cancer occurred among men in the United States in 2008. However, 91% of these new cases were expected to be diagnosed at local and regional stages, which have 5-year relative survival rates of nearly 100%.6 Autopsy studies showed that 30% to 46% of men older than age 50 have microscopic prostate cancer, but less than 20% of men develop clinically overt prostate cancer in their lifetime.103,104 Prostate cancer has less predictable behavior than do other aggressive cancers. Prognostic factors include the size, the histologic grade, the stage of the tumor, and age.
Prostate cancer is suspected because of an abnormal digital rectal examination or elevated serum prostate-specific antigen (PSA) level, which are generally recommended in yearly check-ups for men older than age 50. Diagnosis of prostate cancer is made by histopathologic examination of tissue specimens obtained from the prostate, which also allows estimation of tumor grade. Imaging findings have no role in the early detection and diagnosis of prostate cancer, but provide valuable information in staging the disease. Another role of imaging is guiding biopsy.100
Transrectal ultrasound provides high-resolution images of the prostate gland because a high-frequency (5- to 7.5-MHz) probe can be placed close to the prostate. Ultrasound also allows examination of the prostate in multiple planes and provides very accurate measurements of the prostate gland. The other major use of transrectal ultrasound is guidance for biopsy.100 Only prostate cancers located in the peripheral zone can be reliably detected by ultrasound. Prostate cancer is usually seen as a hypoechoic area relative to the peripheral zone (Fig. 20-13). However, up to 40% of prostate cancers are isoechoic, hampering their detection with ultrasound.105,106 The finding of a hypoechoic area within the peripheral zone is not specific for prostate carcinoma and can also be seen in benign processes such as prostatitis.100,105 Distortion of the normal internal anatomy of the prostate is another important sign that may help in detection of prostate carcinoma, especially in advanced cases.105
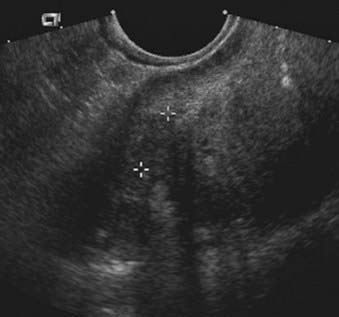
FIGURE 20-13 • Transrectal ultrasound of the prostate depicts hypoechoic tumor within the peripheral zone.
CT, even with contrast enhancement, lacks the soft tissue resolution for the detection of prostate cancer within a normal prostate. Additionally, CT has no advantages over ultrasound in providing guidance for biopsy.100 However, CT may be useful in the detection of distant metastases and lymph nodes.100
MRI provides the best images of the normal zonal anatomy of the prostate.100 On T2-weighted magnetic resonance images, prostate cancer is seen most commonly as a low-signal-intensity area within the high-signal-intensity normal peripheral zone. The detection of prostate cancer on MRI, as on transrectal ultrasound, may be hampered by postbiopsy changes, prostatitis, dystrophic changes, or changes secondary to radiation; or hormonal ablation therapy can be seen as low-signal-intensity areas mimicking tumor.100 It has been shown that MRI should be performed at least 3 weeks after biopsy to prevent under- or over-staging of tumor extent on MRI. The role of MRI in evaluating prostate cancer is mainly to demonstrate extracapsular and seminal vesicle invasion (Fig. 20-14). MRI findings of extracapsular extension include irregular bulge of the prostate margin, contour deformity with step-off or angulated margin, overt disruption of the capsule with direct tumor extension, obliteration of the recto-prostatic angle, and asymmetry of neurovascular bundles. Seminal vesicle invasion is diagnosed when contiguous low-signal-intensity tumor extension into and around seminal vesicles is demonstrated, or when tumor extension along the ejaculatory duct results in nonvisualization of the ejaculatory duct, decreased signal intensity of seminal vesicles, and loss of the seminal vesicle wall on T2-weighted images.107,108
Magnetic resonance spectroscopy is a technique used in prostate imaging that provides metabolic information by detection of cellular metabolites: citrate, creatine, and choline. In tumor localization, combined use of MRI and magnetic resonance spectroscopy provides the highest specificity (91%) among noninvasive methods. Combined use of MRI and magnetic resonance spectroscopy also improves the detection of extracapsular extension.109–111
Current NCCN and American College of Radiology guidelines do not include FDG-PET in the management of prostate cancer.112 Initially, FDG-PET was thought to be useless in prostate cancer, as FDG-PET scans of prostate cancer patients frequently failed to detect sites of viable tumor.113,114 Schöder and colleagues115 provided evidence that PSA values and PSA kinetics can be used to determine the likelihood that FDG-PET imaging will detect tumors in the setting of PSA relapse; the authors suggested threshold values of PSA 2.4 ng/mL and PSA velocity 1.3 ng/mL/year for predicting an abnormal FDG-PET scan. Seltzer and colleagues116 found similar findings in a smaller group of patients. In castrate patients with progressive disease, prostate cancer is most often FDG-avid.54 In prostate cancer patients with a high likelihood of FDG-avid disease, FDG-PET has a role in distinguishing local from distant recurrence and serves as a marker of tumor response to therapy. Tumor FDG-avidity portends a worse clinical outcome for the prostate cancer patient in terms of relapse-free survival.117 This phenomenon has been observed in thyroid cancer,118 leukemia, and lymphoma119 wherein “hypermetabolism” of glucose, visualized by FDG-PET, appears associated with an aggressive tumor phenotype. Hence, lack of FDG uptake, by tumors—although frustrating from a diagnostic perspective—can be reassuring from a prognostic perspective, even when disease is known to be present. Shreve and colleagues noted an apparent association between high FDG-avidity and hormone-refractory disease.114 Animal models demonstrate that tumor FDG-avidity correlates with changes in systemic hormone levels.120,121
Evaluation of the prostate gland is often limited by the presence of excreted FDG in the bladder; some centers have taken a logical approach of catheterizing and draining the bladder before imaging.113,117,122–124 The primary tumor of untreated prostate cancer is usually FDG-avid117 and may be detected as an incidental finding.125 FDG uptake in a prostate lesion does not distinguish malignant from benign disease (e.g., BPH 113 or 123, or focal prostatitis). Nor can FDG-PET differentiate local recurrence from scar tissue after radical prostatectomy, according to one study.122
FDG-PET appears sensitive for metastatic pelvic adenopathy if it is not a subcentimeter lesion.114,124,126 In the detection of metastatic pelvic adenopathy from prostate cancer, as in the detection of other pelvic cancers, FDG-PET/CT is expected to have greater sensitivity and specificity than FDG-PET.23 In early studies, bladder radioactivity sometimes obscured evaluation of pelvic lymph nodes, but this is less of a problem with contemporary data-processing algorithms.114
FDG-PET is reportedly less sensitive for osseous metastases than is bone scintigraphy (e.g., 99mTc-methylene diphosphonate or 99mTc-hydroxymethylene diphosphonate),114 yet this finding is controversial.54,127 The reliability of bone scintigraphy and CT as assays of skeletal tumor burden is generally recognized as poor: metastases centered in the marrow of a bone often go undetected; and these marrow metastases, when dying of anticancer therapy, can appear as “new” lesions on CT and bone scintigraphy (the so-called “flare response phenomenon”). Therapy-induced changes in tumor FDG uptake are concordant with standard clinical measures in approximately 95% of cases54; in assaying therapy response, FDG-PET likely has a role as a substitute for PSA in patients whose tumors produce scanty levels of PSA; to verify the absence of an emerging non-PSA–producing tumor phenotype; and as an arbiter when PSA and conventional imaging are discordant.54
NCCN guidelines suggest scintigraphy with the radiolabeled antibody capromab pendetide (ProstaScint) as an option for diagnostic imaging in the salvage work-up of prostate cancer patients.112 This Food and Drug Administration–approved radioimmunoscintigraphy assay detects glutamate carboxypeptidase II, also known as prostate-specific membrane antigen (PSMA), on its intracellular domain, which in vivo is usually exposed only by dead or dying prostate cells. Early clinical studies suggested PSMA-immunoscintigraphy was superior to CT and MRI for disease staging. After its wider dissemination into routine clinical settings, however, enthusiasm seems to have waned considerably; the reliability of PSMA scanning for tumor detection in routine clinical settings did not appear to live up to the expectations of some. The added value of radioimmunoscintigraphy to other available imaging modalities appears questionable.116 Because capromab pendetide is a murine antibody, it is potentially immunogenic, although adverse reactions occur in fewer than 4% of patients and are usually mild.
Clinical staging, although inexpensive and simple, is not accurate enough for making treatment decisions. Therefore, imaging plays an important role in the staging of prostate cancer. Among the currently available imaging techniques, MRI is the most accurate method for local staging.100 MRI may be cost-effective, especially for patients who have an intermediate risk of having extracapsular extension and recurrent cancer defined by clinical prognostic factors such as digital rectal examination (stages T1 and T2), preoperative PSA (10 to 20 ng/mL), and biopsy Gleason score of 7.100
Testes
The testes are located within the scrotum. The scrotal septum divides the scrotal sac into two compartments. The normal adult testis measures 4 to 5 cm long, 2 to 3 cm wide, and 2 to 3 cm in anteroposterior diameter. The weight of the testis is 15 to 25 grams. The appendix of the testis, which is a müllerian duct remnant, is located at the upper pole of the testis.128
An outpouching of peritoneum called the tunica vaginalis envelops each testis anterolaterally. At the posterolateral bare area of the testis is the epididymis. The tunica albuginea is a dense fibrous connective tissue capsule covering the testis itself. Along the posterior aspect of the testis, tunica albuginea invaginates into the testis and forms the mediastinum testis. Septae from the mediastinum testis divide the testis into pyramidal lobules. Convoluted seminiferous tubules within the lobules of the testis straighten to form the rete testis in the region of the mediastinum testis. The efferent ductules run from the rete testis to the duct of epididymis. The ductus deferens (vas deferens) is a continuation of the duct of epididymis. The ductus deferens is approximately 30 cm long and extends to the ejaculatory duct. The ductus deferens, testicular vessels, and lymphatics are surrounded by a connective tissue fascia called spermatic cord. Each layer of the anterior abdominal wall musculature and parts of the cremasteric muscle contribute to form the spermatic cord.128
The testicular arteries arise from the aorta or renal artery. The veins of the testis converge at the mediastinum testis and form the pampiniform plexus.128
Testicular Tumors
Testicular tumors are rare, constituting less than 1% of all malignant neoplasms in men, but are the most common malignancy occurring in young men and adolescents.6,128 They can be classified into germ cell tumors, arising from spermatogenic cells, and non–germ cell tumors, deriving from the sex cords (Sertoli cells) and stroma (Leydig cells). Germ cell tumors constitute 95% of testicular neoplasms and are almost uniformly malignant. However, non–germ cell primary tumors of the testis are malignant in only 10% of cases. The secondary tumors of the testis include tumors such as lymphoma, leukemia, and metastases.128
Testicular tumors most commonly present with painless scrotal enlargement or as an incidental testicular nodule. Pain is an uncommon presenting symptom, reported by approximately 10% of patients. In some cases, testicular neoplasia may initially be misdiagnosed as orchitis. Testicular tumors sometimes may undergo regression, necrosis, and scarring (so-called “burned-out” germ cell tumors); therefore, some patients may have normal or small testes at presentation. Some patients, especially those who have an aggressive histologic tumor type, may present with metastases. A small group of patients with hormonally active tumors present with endocrine abnormalities, most commonly gynecomastia.128,129
Ultrasonography performed with high-frequency transducers is the most commonly used primary imaging modality for the initial workup and evaluation of a scrotal mass, as well as in the staging of a known testicular tumor (Fig. 20-15). It has been shown to be almost 100% sensitive in the detection of scrotal masses. Intratesticular versus extratesticular pathologic conditions can be differentiated with 98% to 100% sensitivity.128–130 The normal testis demonstrates a homogeneous echotexture. The covering tunica albuginea is generally not seen as a separate structure, except in mediastinum testis, where it can be seen as an echogenic line invaginating into the testis. The epididymis is isoechoic or slightly hyperechoic compared with the testis.
Ultrasound is very helpful in the localization of the scrotal mass, whether intratesticular or extratesticular. Further morphologic characterization of the lesion (e.g., as cystic or solid) is also possible. Solid intratesticular masses are frequently malignant. Testicular tumors are usually hypoechoic compared with the surrounding testicular parenchyma. Some tumors can be heterogeneous, containing calcifications or cystic areas. Color Doppler ultrasound may be helpful to identify an isoechoic mass or differentiate a complex cystic lesion from a solid mass.128
MRI can be a useful problem-solving tool in the rare cases in which ultrasound is inconclusive.131 The normal testis demonstrates a homogeneous, intermediate signal intensity on T1-weighted images and high signal intensity on T2-weighted images. The tunica albuginea appears as a thin, low-signal-intensity line surrounding the testis on both T1-weighted and T2-weighted images. The epididymis is isointense or slightly hypointense relative to the testis on T1-weighted images and hypointense on T2-weighted images.128,132 Testicular tumors often demonstrate homogeneous medium signal intensity similar to normal testis on T1-weighted images and decreased signal intensity within the high signal intensity of normal testis on T2-weighted images. Homogeneous or heterogeneous high-signal-intensity tumors or tumors with signal intensity similar to that of the testis can also be seen.132 MRI is very sensitive in depicting the tumors; however, its specificity as to tumor type is low.
Current NCCN guidelines recommend FDG-PET for patients with stage IIB, IIC, and III seminoma who have a residual mass and normal serum markers after primary chemotherapy; in addition, the guidelines state, “for persistent elevated beta-HCG [human chorionic gonadotropin], which is not rising, repeat serial markers, testosterone suppression test, and consider a PET scan.”133 Gonadal germ cell tumors comprise several histologic subtypes,134 possibly with differing FDG-avidities.135–138 Overall, FDG-PET appears sensitive in detecting seminoma139 and nonseminomatous germ cell carcinoma (NSGCT).140 A recent statement by the European Germ Cell Cancer Consensus (EGCCC) group141 states that FDG-PET does not improve staging of germ cell cancers compared with CT alone; to support this, the authors cite articles that show FDG-PET does improve staging compared with CT alone136,142,143 and is more sensitive than CT.136,143,144 Similarly, the EGCCC group makes the potentially misleading statement that PET scans are not recommended as part of routine initial staging procedures; the citations following this statement (see original EGCCC article141) include no articles that recommend against the use of PET in initial staging. Some articles cited by the EGCCC used PET methodology no longer accepted as state-of-the art (e.g., filtered backprojection data reconstruction145,146). A rationale for early detection of tumor relapse in NSGCT is to “catch” recurrent disease in a stage with a more favorable prognosis.147 In stage I NSGCT patients with negative CT and negative tumor markers who were postorchiectomy, FDG-PET/CT identified metastatic disease in approximately 15% to 21% of cases.143,144 Yet in patients with stage I NSGCT who have a high risk for recurrence, a negative FDG-PET scan does not modify or substratify the risk of relapse.144 As suggested by the NCCN guidelines, in the postchemotherapy setting FDG-PET appears able to distinguish viable germ cell tumor from inflammatory and necrotic debris (which are often somewhat FDG-avid).137,139,140,148,149
FDG-PET accuracy appears lower in patients with metastatic NSGCT and postchemotherapy masses.140 FDG-PET is incapable of distinguishing mature teratoma from inflammatory residuum, using standard semiquantitative (standard uptake value) PET measurements150,151; postchemotherapy masses containing mature teratoma are frequently false-negative.135,140,150 In general, with the conventional approach to FDG-PET, it appears that a positive FDG-PET scan is highly reliable in diagnosing residual tumor, whereas a negative FDG-PET is less reliable.152
Sugawara and colleagues found that more sophisticated quantitative analysis of FDG tumor pharmacokinetics distinguishes mature teratoma from inflammatory residuum151; yet the findings remain to be confirmed. Tissue inflammation related to therapy and tumor death is an FDG-avid process. In a small group of patients, Haba and colleagues153 attempted to circumvent the problem of FDG-avid inflammation obscuring tumor evaluation: in patients with stage 2 NSGCT, the investigators acquired FDG-PET scans 72 to 96 hours after initiation of chemotherapy, presumably aiming for a time-point after cancer cytotoxicity and cell death but before significant tissue infiltration by the FDG-avid inflammatory cells that clear tumor debris. Four of the six patients’ scans demonstrated complete resolution of tumor FDG-uptake, and one patient’s scan demonstrated a 50% reduction; these five patients went on to demonstrate a complete response by conventional criteria. Validation in a larger patient population is needed.
Penis
The penis is composed of two lateral corpora cavernosa and the ventral and medial corpus spongiosum. The posterior portions of the corpora cavernosa called the crura and the expanded posterior end of corpus spongiosum called the bulb of the penis form the radix penis located in the perineum. The major portions of the corpora cavernosa and the corpus spongiosum are found within the shaft of the penis. At its distal end, the corpus spongiosum expands to form the glans penis. The tunica albuginea, which is composed of fibrous tissue, covers all three corpora. The Buck fascia is a fibrous envelope that separates the corpora cavernosa from the corpus spongiosum. The suspensory ligaments of the penis blend with the Buck fascia and extend to the symphysis pubis.154
Penile Carcinoma
Carcinoma of the penis is a rare disease. Most penile cancers are squamous cell carcinomas. Penile carcinoma’s causal factors are unclear, but it is seldom seen in circumcised men and it is related to the presence of foreskin, irritant effects of smegma, and poor hygiene. Treatment depends on the degree of involvement and ranges from partial penile amputation to total penectomy.154 MRI is a useful tool to evaluate penile tumors that appear iso- to hypointense on T1-weighted images and hypointense on T2-weighted images compared with the surrounding corporeal body. MRI is especially useful when the tumor extends into the radix penis, which is difficult to evaluate clinically. Depiction of tumor extension into the bulb of the penis or across the urogenital diaphragm is important in determining treatment options, which include radiation or surgery.154
Not much clinical data has been reported regarding FDG-PET for penile cancer, yet it appears that untreated, primary penile cancers are frequently FDG-avid and that FDG-PET/CT is highly accurate for lymph node staging.155
Urinary Bladder and Urethra
The urinary bladder is extraperitoneal and located behind the symphysis pubis. In the male, the bladder base is related to the base of the prostate gland, seminal vesicles, and ductus deferens. In the female, the bladder base is related to the cervix, vagina, periurethral muscles, and urethra. In infants, the internal sphincter of the urinary bladder neck lies above the symphysis pubis and the upper border of the full urinary bladder rises to the level of the first sacral vertebra. In adults, the urinary bladder neck is located at the level of the symphysis pubis, and the full urinary bladder reaches the level of the second or third sacral vertebra.156
The male urethra is divided into three segments: prostatic, membranous, and penile urethra. The penile urethra is also divided into bulbar and anterior portions. The prostatic urethra is the 3- to 4-cm portion surrounded by the prostate gland. The membranous urethra is 1 cm long, extending from the apex of the prostate to the bulb of the penis, passing through the urogenital diaphragm. The wall of the membranous urethra consists of an inner layer of smooth muscle bundles and an outer, thicker layer of striated muscle fibers called the external sphincter. The penile urethra is 15 cm long (in the flacid state) and is located within the corpus spongiosum.99,154
The female urethra is 4 cm long and extends from the internal urethral orifice of the bladder to the external urethral orifice just anterior to the vaginal opening. The female urethra is divided into three portions: the upper, middle, and lower thirds. The upper third is composed mainly of an external muscular coat, which is continuous with the bladder neck. The middle third of the urethra is surrounded by an outer layer of circular striated muscle and an inner layer of smooth muscle in submucosa. The lower third of the female urethra is almost entirely fibrous tissue.156
Bladder Cancer
Bladder carcinoma is the most common malignancy involving the urethlial tract. It occurs more commonly in males than in females.6 Most bladder cancers are of epithelial origin and up to 90% are transitional cell carcinomas. Squamous cell carcinomas (5% to 10%) and adenocarcinomas (2%) are the other types of epithelial bladder cancers. Squamous cell carcinomas are associated with chronic inflammatory diseases of the urinary bladder.156 The prognosis of urinary bladder cancer depends on histologic type, pattern of tumor growth, and anatomic extent of disease at the time of diagnosis. Most patients present with superficial disease, for which transurethral resection and multiple cystoscopic biopsies are sufficient for diagnosis, staging, and definitive therapy. Staging with cross-sectional imaging is necessary when deeply invasive tumors are detected (Fig. 20-16 and Fig. 20-17). Evaluation of bladder cancer consists of determining the site, number and growth pattern of the tumors, depth of muscular and perivesical invasion, and lymph node status.
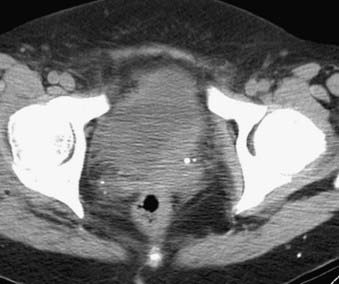
FIGURE 20-16 • Contrast-enhanced axial computed tomography image reveals bladder cancer causing thickening of the left posterolateral wall.
Different numbers of staging accuracies for bladder carcinoma were reported with CT (40% to 92%) and with MRI (50% to 96%). Dynamic contrast-enhanced MRI provides increased tumor detection and staging accuracy for superficial lesions. Limitations of both MRI and CT include their inabilities to depict small tumor growth into the muscle layer of the bladder wall or differentiate between tumor and inflammatory changes.157–163
Current NCCN guidelines do not mention a role for FDG-PET in the management of bladder cancer.164 Currently, no original research articles on bladder cancer and state-of-the-art FDG-PET/CT are available; a trial of modern FDG-PET/CT in bladder cancer ought to be done. Evaluation of primary tumors in bladder cancer by FDG-PET is generally precluded by the presence of excreted FDG in the urine; the intensity of radioactivity in the urine can obscure evaluation of adjacent nodal regions.165 This problem can be addressed by the use of indwelling bladder catheters and irrigation,165 diuresis,166,167 delayed imaging,166 and clever use of intravenous radiographic contrast and patient positioning.168 For lymph node staging, FDG-PET has a sensitivity of 67% to 76% and specificity of 86% to 97%, although some of the published studies have used outdated PET methodology.126,169–171
Rectum and Sigmoid Colon
The sigmoid colon is usually located in the true pelvis. It is an intraperitoneal organ. The rectosigmoid junction is located approximately at the level of the third segment of the sacrum. The rectosigmoid junction forms an acute angle and is fixed by the mesentery. The rectum is the terminal portion of the colon and ends at the anus. Anatomically, the rectum comprises a 10- to 12-cm long rectum proper and a 2.5- to 3-cm long anal canal. The upper portion of the rectum is totally covered by peritoneum. The dorsal part of the rectum is not covered by the peritoneum. The anterior and lateral aspects of the rectum are also covered by peritoneum to the level of the fifth sacral or first coccygeal vertebra. The peritoneal reflection anteriorly creates the rectovesical fossa in the male and the rectouterine fossa (pouch of Douglas) in the female. The lateral peritoneal recesses form the pararectal spaces. The ischiorectal fossae are located laterally to the rectum and bounded laterally by the obturator internus muscle and the levator ani muscle.172 The anal canal is the external aperture of the colon and terminates at the anus. The levator ani muscle attaches laterally to the anal canal and acts as an accessory sphincter. It consists of three parts: the iliococcygeus muscle, the pubococcygeus muscle, and the puborectalis muscle. The puborectalis muscle is also known as the sphincter muscle of the rectum. Two sphincters surround the anal canal. The internal anal sphincter is a 2- to 3-mm-thick ring, formed by the thickening of circular fibers of the muscularis propria. The external anal sphincter is elliptical in shape with three concentric layers of striated muscle fibers: deep, superficial, and subcutaneous parts.172
Rectal Cancer
Colorectal carcinoma is the most common cancer of the gastrointestinal tract, and the second most common cause of cancer-related death in the United States.6 The most common histologic type of sigmoid and rectal cancer is adenocarcinoma. The standard surgical management for rectal carcinoma was once abdominoperineal resection and formation of permanent colostomy. However, new surgical techniques, such as low-anterior resection with anal sphincter sparing and local excision, have become more popular for well-differentiated, early-stage tumors. Preoperative staging of rectal cancer determines the choice of surgical approach and the need for adjuvant radio- or chemotherapy. In addition, the degree of tumor invasion through the layers of the rectal wall and the patient’s nodal status are very important features that determine the risk of local tumor recurrence.173,174 For these reasons, correct preoperative local staging of rectal cancer is essential.
Transrectal ultrasound and MRI are commonly used for assessing tumor penetration through the rectal wall layers and nodal status. Unlike CT, both transrectal ultrasound and MRI can distinguish the layers of the rectal wall and allow depiction of tumor growth through these layers. According to the TNM staging system, a stage T1 tumor is limited to the mucosa and submucosa and does not extend to the muscularis propria. Stage T2 refers to tumors that partially involve the muscularis propria. A stage T3 tumor totally disrupts the muscular layer and extends into the perirectal fat. When invasion is present in adjacent organs or structures, the tumor is identified as stage T4 (Fig. 20-18). Rectal cancer is an FDG-avid disease,175 although the degree of FDG-avidity can be variable, and not clearly associated with the size of the tumor176 or the proliferative rate of its cancer cells.177 Two major factors, in determining the FDG-avidity of a rectal tumor are its density of cancer cells and the hexokinase activity of those cells.178 Although nonmucinous adenocarcinoma is FDG-avid on PET, mucinous cancer can lack distinct FDG uptake, likely because of the relative hypocellularity of such tumors.179 Premalignant polyps can be FDG-avid as well, and other FDG-avid diseases of the rectum can mimic a tumor on FDG-PET, including abscesses and focal rectal inflammation caused by prior polypectomy.176
For the local staging of rectal cancer, the reported accuracy of transrectal ultrasound ranges between 70% and 81%, and that for MRI between 71% and 91%. Most under-staging errors are caused by the inability of both methods to detect microscopic or minimal invasion. Inflammatory changes in perirectal fat mimicking tumor invasion are reported to be the most frequent cause of over-staging.180–187
The 2008 NCCN guidelines state that FDG-PET is not routinely recommended in the workup of rectal cancer appropriate for resection.188 Yet the addition of FDG-PET to conventional staging changes clinical management in approximately 17% to 27% of cases101,175,189–193 (Fig. 20-19). FDG-PET findings may help clinicians avoid futile surgery or adjust radiotherapy fields.190 Compared with conventional CT-guided planning of IMRT, FDG-PET changes the radiation treatment plan in approximately 26% of cases.189,190 A small study suggested that PET-CT reduces the interobserver variability of target-volume delineation in rectal cancer, compared with CT-guided planning.194 One controversial issue in the use of FDG-PET/CT for radiotherapy planning is the proper method of adjusting the PET image intensity windows so that target volume is accurately delineated.195–197 Target volumes are an anatomic issue and FDG-PET is not an anatomic imaging modality in its essence. FDG-PET may provide a coarse scintigraphic estimate of tumor volume; PET image intensity windows can be adjusted so that the region (i.e., voxels) of FDG uptake closely matches the true anatomic volume of tumor. The question remains how to do this accurately without foreknowledge of true tumor volume. The group at St-Luc University Hospital, in Brussels, Belgium, provided one attractive, semiautomated computer algorithm intended for this purpose,198 which they then validated against surgical specimens in pharyngolaryngeal squamous cell carcinoma.199 Of note, in this study, FDG-PET was found to be the superior modality for delineating target volume, compared with CT and MRI.
FDG-PET/CT is most accurate in the pretherapy setting,178,200 being extremely sensitive in the detection of liver and lung metastases,200 the two viscera most commonly involved by metastatic disease at initial presentation.201 In general, FDG-PET/CT has long been recognized as superior to conventional CT for detecting hepatic metastases,176,202,203 although its sensitivity decreases in characterizing subcentimeter hepatic lesions.204,205 FDG-PET is recommended by the NCCN for the workup of patients with metachronous resectable metastases.188 FDG-PET will detect extrahepatic disease in 18% to 32% of patients planning to undergo hepatic resection based on conventional imaging; in 20% to 40% of these patients with extrahepatic disease, it will lead to a change in management.204–207 A recent study from Washington University208 found that patients with hepatic metastases who underwent FDG-PET for preoperative staging had a much higher 5-year survival rate than did historic controls. Yet structural imaging will continue to have an important role, as it provides indispensable anatomic information for surgical planning.
Dedicated chest CT is likely more sensitive than FDG-PET/CT for the detection of small lung metastases.209 The companion CT of a PET/CT study is optimized for PET imaging—not for imaging pulmonary anatomy—making it more difficult to detect subcentimeter pulmonary nodules. Such tiny lesions are often nonspecific and require surveillance with optimized chest CT.
For patients with stage II or III rectal cancer, treatment with preoperative combined-modality therapy (CMT) followed by total mesorectal excision is a common approach210; hypothetically, it would be advantageous to know how well tumors respond to preoperative CMT early in the course of treatment, so that ineffective treatments could be changed.211 Yet ultrasound, MRI, CT, and digital rectal examination all do a suboptimal job of identifying tumor response to preoperative CMT.212–215 A number of studies have suggested that FDG-PET is the best noninvasive modality for identifying the presence and extent of rectal tumor response, correlating with histopathology177,211,213,216–219 and prognosis.192,220 Most studies on these methods examined rectal tumor FDG uptake before and after the completion of preoperative therapy, although one exception studied therapy-induced changes during treatment (day 12) and found one of the highest accuracy levels reported.218 A recent Danish study found that FDG was not accurate in identifying the presence and extent of rectal tumor response to therapy221; however, in the Danish study, FDG-PET was performed only at a single time-point (post-treatment) and therapy-induced changes in FDG uptake were therefore not examined. Therapy-induced reductions in rectal tumor FDG uptake appear to mainly reflect reductions in the tumor cancer cell population177 and cellular hexokinase activity,178 rather than changes in cellular proliferation.177 Post-therapy tumor inflammation is a recognized phenomenon222,223 and it is FDG-avid, potentially obscuring the signal loss associated with the death of FDG-avid cancer cells, by FDG-PET. Tumor inflammation is likely a good sign in terms of patient prognosis: the Memorial Sloan-Kettering Cancer Center group reported that a paucity of inflammatory or ulcerative changes in tumors after preoperative chemoradiotherapy is an independent predictor of a worse outcome.224
The American Society of Clinical Oncology,225 the European Society for Medical Oncology,226 and the NCCN188 do not currently recommend PET or MRI for routine tumor surveillance in the management of rectal cancer. Yet the NCCN guidelines do recommend that physicians consider obtaining an FDG-PET scan in the setting of a rising serum carcinoembryonic antigen (CEA) level. Serum CEA levels play a key role in surveillance, as rises in CEA levels precede detectable abnormalities on conventional imaging, typically by 5 to 8 months.227,228 Yet several studies have found that FDG-PET/CT is highly accurate for detection of recurrent disease and alters patient management in a significant percentage of cases (26%-61%).179,229–232 In studies of colorectal cancer patients, FDG-PET has repeatedly proven its usefulness in the setting of rising CEA when conventional imaging studies were normal233–236 or equivocal.229 Moreover, prospective comparisons236,237 of FDG-PET and spiral CT in colorectal cancer patients with suspected recurrence found FDG-PET superior for both staging local recurrence and detecting hepatic metastases; in this setting, the use of FDG-PET changed clinical management in 38% of cases,237 often preventing futile surgery, to the benefit of the patient as well as the healthcare system.236 Retrospective studies yielded similar findings.238–241
If conventional imaging documents metachronous metastases that appear resectable, NCCN guidelines recommend evaluation by FDG-PET.188 A well-designed study by the National Institutes of Health (NIH),234 albeit with a small study population, addressed the following issue: 60% to 90% of patients with CEA elevations and normal conventional structural imaging findings have recurrent disease,242,243 yet at laparotomy, only half of these recurrences will prove resectable (resectability being associated with a more favorable prognosis)244; hence, the main role of imaging is not for detecting recurrence (CEA levels being more sensitive than imaging in this regard), but for defining recurrences as resectable or nonresectable. The NIH group studied preoperative FDG-PET in two groups of patients staged by conventional imaging: those with no imaging evidence of disease and those with recurrence that appeared resectable on conventional imaging. Extra-abdominal disease, visualized by PET, was confirmed by biopsy; patients without extra-abdominal disease on PET went to laparotomy. Two surgeons, one blinded and one unblinded to PET information, inspected the abdominal cavity sequentially. Of the 15 patients thought to have resectable disease based on conventional imaging, 6 were found to be unresectable at laparotomy; 11 patients with occult disease on imaging were found to have intraabdominal recurrences at laparotomy, of which 4 were unresectable. Of these 10 cases of unresectable disease in patients who went to laparotomy, preoperative FDG-PET had correctly identified 90% as being unresectable. Another study reported similar accuracy for FDG-PET in predicting resectability.236 Of the 16 cases of resectable disease in the NIH study, preoperative FDG-PET incorrectly identified 19% as unresectable. FDG-PET, when read by imaging specialists blinded to conventional imaging findings, located tumor in all patients with known recurrence; in patients with intra-abdominal recurrences that were invisible to conventional imaging, FDG-PET detected 89% of tumors found at laparotomy by the two surgeons. Moreover, in the 11 patients with intra-abdominal recurrences that were invisible on conventional imaging, the blinded surgeon missed two recurrences that were detected with FDG-PET and were found by the surgeon who operated with the benefit of knowing the PET results. Of the two patients without recurrent colorectal cancer, at laparotomy and confirmed by 2 to 4 years of follow-up, FDG-PET correctly excluded disease in one, while incorrectly identifying recurrent disease in the other. Thus, preoperative FDG-PET provides important guidance to surgeons in locating recurrences at laparotomy; identification of extra-abdominal disease may allow avoidance of futile surgery, but the fact that FDG-PET incorrectly identified 19% of resectable abdominal recurrences as unresectable raises doubt as to its reliability for deciding to forgo surgery, as removal of resectable disease improves survival. Moore and colleagues reported similar sensitivity (84%) for FDG-PET using modern techniques in the detection of pelvic recurrence of rectal cancer, with specificity of 88%.245 It must be noted that the NIH study and the study by Moore and colleagues used FDG-PET, not FDG-PET/CT. Cohade and colleagues246 found that PET/CT improved diagnostic accuracy, compared with FDG-PET alone, in a population of colorectal cancer patients. In rectal cancer patients, Even-Sapir and colleagues247 found PET/CT superior to PET alone, as physiologic uptake in displaced viscera sometimes fooled readers of PET images without CT; the authors reported that FDG-PET/CT had 98% sensitivity and 96% sensitivity in the detection of pelvic recurrence on a per-lesion basis.
Even-Sapir and colleagues found FDG-PET/CT extremely accurate in distinguishing viable tumor from postoperative scarring in the presacral region, with 100% sensitivity and 96% specificity. Fukunaga and colleagues248 fused independent PET and CT images using software, and found that software-fused FDG-PET/CT had diagnostic accuracy of 93%, which was superior to that of CT alone; in particular, FDG-PET distinguished viable tumor from postoperative scarring. The complementary information altered management in 26% of cases. Yet software-fused PET/CT is not the same as true PET/CT acquired with hybrid PET/CT scanners249,250; the University of California, Los Angeles, group demonstrated that recurrence of colorectal cancer is detected by “in-line” hardware-fusion PET/CT with significantly greater accuracy than it is with software-fused PET/CT.249
Kinkel and colleagues203 reported a meta-analysis comparing CT, MRI, ultrasound, and FDG-PET for the detection of hepatic metastases as sites of relapsed gastrointestinal cancers. FDG-PET had a sensitivity of 90% (95% confidence interval [CI] 80%-97%) that was significantly superior to the sensitivities of all other modalities. Whiteford and colleagues reported PET-CT to be superior to conventional CT in detecting hepatic and extrahepatic metastases in recurrent rectal cancer.179
1 Carrington B, Hricak H. Anatomy of the pelvis. In: Hricak H, Carrington BM, editors. MRI of the pelvis. A text atlas. London: Martin Dunitz Ltd; 1991:43.
2 Carrington B, Hricak H. The uterus and vagina. In: Hricak H, Carrington BM, editors. MRI of the pelvis. A text atlas. London: Martin Dunitz Ltd; 1991:93.
3 Ascher SM, Reinhold C. Imaging of cancer of the endometrium. Radiol Clin North Am. 2002;40(3):563-576.
4 Nicolet V, Carignan L, Bourdon F, et al. MR imaging of cervical carcinoma: a practical staging approach. Radiographics. 2000;20(6):1539-1549.
5 Scheidler J, Heuck AF. Imaging of cancer of the cervix. Radiol Clin North Am. 2002;40(3):577-590. vii
6 Jemal A, Siegel R, Ward E, et al. Cancer statistics, 2008. CA Cancer J Clin. 2008;58(2):71-96.
7 Barakat R, Grigsby PW, Zaino SP. Corpus epithelial tumors. In: Hoskins W, Perez CA, Young RC, editors. Principles and practice of gynecologic oncology. Philadelphia: Lippincott Williams & Wilkins; 2000:919.
8 Boronow RC, Morrow CP, Creasman WT, et al. Surgical staging in endometrial cancer: clinical-pathologic findings of a prospective study. Obstet Gynecol. 1984;63(6):825-832.
9 Gordon AN, Fleischer AC, Dudley BS, et al. Preoperative assessment of myometrial invasion of endometrial adenocarcinoma by sonography (US) and magnetic resonance imaging (MRI). Gynecol Oncol. 1989;34(2):175-179.
10 Balfe DM, Van Dyke J, Lee JK, et al. Computed tomography in malignant endometrial neoplasms. J Comput Assist Tomogr. 1983;7(4):677-681.
11 Walsh JW, Goplerud DR. Computed tomography of primary, persistent, and recurrent endometrial malignancy. AJR Am J Roentgenol. 1982;139(6):1149-1154.
12 Hardesty LA, Sumkin JH, Hakim C, et al. The ability of helical CT to preoperatively stage endometrial carcinoma. AJR Am J Roentgenol. 2001;176(3):603-606.
13 Hirano Y, Kubo K, Hirai Y, et al. Preliminary experience with gadolinium-enhanced dynamic MR imaging for uterine neoplasms. Radiographics. 1992;12(2):243-256.
14 Hricak H, Rubinstein LV, Gherman GM, et al. MR imaging evaluation of endometrial carcinoma: results of an NCI cooperative study. Radiology. 1991;179(3):829-832.
15 Lien HH, Blomlie V, Trope C, et al. Cancer of the endometrium: value of MR imaging in determining depth of invasion into the myometrium. AJR Am J Roentgenol. 1991;157(6):1221-1223.
16 National Comprehensive Cancer Network, NCCN clinical practice guidelines in oncology, Uterine neoplasms. V.I.2008; 2008.
17 Belhocine T, De Barsy C, Hustinx R, et al. Usefulness of (18)F-FDG PET in the post-therapy surveillance of endometrial carcinoma. Eur J Nucl Med Mol Imaging. 2002;29(9):1132-1139.
18 Park JY, Kim EN, Kim DY, et al. Comparison of the validity of magnetic resonance imaging and positron emission tomography/computed tomography in the preoperative evaluation of patients with uterine corpus cancer. Gynecol Oncol. 2008;108(3):486-492.
19 Chao A, Chang TC, Ng KK, et al. 18F-FDG PET in the management of endometrial cancer. Eur J Nucl Med Mol Imaging. 2006;33(1):36-44.
20 Suzuki R, Miyagi E, Takahashi N, et al. Validity of positron emission tomography using fluoro-2-deoxyglucose for the preoperative evaluation of endometrial cancer. Int J Gynecol Cancer. 2007;17(4):890-896.
21 Saga T, Higashi T, Ishimori T, et al. Clinical value of FDG-PET in the follow up of post-operative patients with endometrial cancer. Ann Nucl Med. 2003;17(3):197-203.
22 Horowitz NS, Dehdashti F, Herzog TJ, et al. Prospective evaluation of FDG-PET for detecting pelvic and para-aortic lymph node metastasis in uterine corpus cancer. Gynecol Oncol. 2004;95(3):546-551.
23 Wahl RL. Why nearly all PET of abdominal and pelvic cancers will be performed as PET/CT. J Nucl Med. 2004;45(Suppl 1):82S-95S.
24 Chung CK, Nahhas WA, Zaino R, et al. Histologic grade and lymph node metastasis in squamous cell carcinoma of the cervix. Gynecol Oncol. 1981;12(3):348-354.
25 Cobby M, Browning J, Jones A, et al. Magnetic resonance imaging, computed tomography and endosonography in the local staging of carcinoma of the cervix. Br J Radiol. 1990;63(753):673-679.
26 Kim SH, Choi BI, Lee HP, et al. Uterine cervical carcinoma: comparison of CT and MR findings. Radiology. 1990;175(1):45-51.
27 Subak LL, Hricak H, Powell CB, et al. Cervical carcinoma: computed tomography and magnetic resonance imaging for preoperative staging. Obstet Gynecol. 1995;86(1):43-50.
28 Yildirim Y, Sehirali S, Avci ME, et al. Integrated PET/CT for the evaluation of para-aortic nodal metastasis in locally advanced cervical cancer patients with negative conventional CT findings. Gynecol Oncol. 2008;108(1):154-159.
29 Narayan K, Hicks RJ, Jobling T, et al. A comparison of MRI and PET scanning in surgically staged loco-regionally advanced cervical cancer: potential impact on treatment. Int J Gynecol Cancer. 2001;11(4):263-271.
30 Reinhardt MJ, Ehritt-Braun C, Vogelgesang D, et al. Metastatic lymph nodes in patients with cervical cancer: detection with MR imaging and FDG PET. Radiology. 2001;218(3):776-782.
31 Rose PG, Adler LP, Rodriguez M, et al. Positron emission tomography for evaluating para-aortic nodal metastasis in locally advanced cervical cancer before surgical staging: A surgicopathologic study. J Clin Oncol. 1999;17(1):41-45.
32 Wright JD, Dehdashti F, Herzog TJ, et al. Preoperative lymph node staging of early-stage cervical carcinoma by [18F]-fluoro-2-deoxy-D-glucose-positron emission tomography. Cancer. 2005;104(11):2484-2491.
33 Goff BA, Muntz HG, Paley PJ, et al. Impact of surgical staging in women with locally advanced cervical cancer. Gynecol Oncol. 1999;74(3):436-442.
34 Vergote I, Amant F, Berteloot P, et al. Laparoscopic lower para-aortic staging lymphadenectomy in stage IB2, II, and III cervical cancer. Int J Gynecol Cancer. 2002;12(1):22-26.
35 Esthappan J, Mutic S, Malyapa RS, et al. Treatment planning guidelines regarding the use of CT/PET-guided IMRT for cervical carcinoma with positive paraaortic lymph nodes. Int J Radiat Oncol Biol Phys. 2004;58(4):1289-1297.
36 Tsai CS, Chang TC, Lai CH, et al. Preliminary report of using FDG-PET to detect extrapelvic lesions in cervical cancer patients with enlarged pelvic lymph nodes on MRI/CT. Int J Radiat Oncol Biol Phys. 2004;58(5):1506-1512.
37 Malyapa RS, Mutic S, Low DA, et al. Physiologic FDG-PET three-dimensional brachytherapy treatment planning for cervical cancer. Int J Radiat Oncol Biol Phys. 2002;54(4):1140-1146.
38 Lin LL, Mutic S, Low DA, et al. Adaptive brachytherapy treatment planning for cervical cancer using FDG-PET. Int J Radiat Oncol Biol Phys. 2007;67(1):91-96.
39 Grigsby PW, Mutch DG, Rader J, et al. Lack of benefit of concurrent chemotherapy in patients with cervical cancer and negative lymph nodes by FDG-PET. Int J Radiat Oncol Biol Phys. 2005;61(2):444-449.
40 Husain A, Akhurst T, Larson S, et al. A prospective study of the accuracy of 18Fluorodeoxyglucose positron emission tomography (18FDG PET) in identifying sites of metastasis prior to pelvic exenteration. Gynecol Oncol. 2007;106(1):177-180.
41 National Comprehensive Cancer Network, NCCN clinical practice guidelines in oncology, Cervical Cancer. V.I.2008; 2008.
42 Chang T-C, Law K-S, Hong J-H, et al. Positron emission tomography for unexplained elevation of serum squamous cell carcinoma antigen levels during follow-up for patients with cervical malignancies. Cancer. 2004;101(1):164-171.
43 Chung HH, Jo H, Kang WJ, et al. Clinical impact of integrated PET/CT on the management of suspected cervical cancer recurrence. Gynecol Oncol. 2007;104(3):529-534.
44 Lai C-H, Huang K-G, See L-C, et al. Restaging of recurrent cervical carcinoma with dual-phase [18F]fluoro-2-deoxy-D-glucose positron emission tomography. Cancer. 2004;100(3):544-552.
45 Hricak H, Hamm B, Semelka RC, et al. Carcinoma of the uterus: use of gadopentetate dimeglumine in MR imaging. Radiology. 1991;181(1):95-106.
46 Hricak H, Lacey CG, Sandles LG, et al. Invasive cervical carcinoma: comparison of MR imaging and surgical findings. Radiology. 1988;166(3):623-631.
47 Lien HH, Blomlie V, Kjorstad K, et al. Clinical stage I carcinoma of the cervix: Value of MR imaging in determining degree of invasiveness. AJR Am J Roentgenol. 1991;156(6):1191-1194.
48 Sironi S, Belloni C, Taccagni GL, et al. Carcinoma of the cervix: value of MR imaging in detecting parametrial involvement. AJR Am J Roentgenol. 1991;156(4):753-756.
49 Togashi K, Nishimura K, Sagoh T, et al. Carcinoma of the cervix: staging with MR imaging. Radiology. 1989;171(1):245-251.
50 Kim SH, Kim SC, Choi BI, et al. Uterine cervical carcinoma: evaluation of pelvic lymph node metastasis with MR imaging. Radiology. 1994;190(3):807-811.
51 Scheidler J, Hricak H, Yu KK, et al. Radiological evaluation of lymph node metastases in patients with cervical cancer. A meta-analysis. JAMA. 1997;278(13):1096-1101.
52 Schwarz JK, Siegel BA, Dehdashti F, et al. Association of posttherapy positron emission tomography with tumor response and survival in cervical carcinoma. JAMA. 2007;298(19):2289-2295.
53 Downey RJ, Akhurst T, Ilson D, et al. Whole body 18FDG-PET and the response of esophageal cancer to induction therapy: results of a prospective trial. J Clin Oncol. 2003;21(3):428-432.
54 Morris MJ, Akhurst T, Larson SM, et al. Fluorodeoxyglucose positron emission tomography as an outcome measure for castrate metastatic prostate cancer treated with antimicrotubule chemotherapy. Clin Cancer Res. 2005;11(9):3210-3216.
55 Ong SC, Schoder H, Lee NY, et al. Clinical utility of 18F-FDG PET/CT in assessing the neck after concurrent chemoradiotherapy for locoregional advanced head and neck cancer. J Nucl Med. 2008;49(4):532-540.
56 Schelling M, Avril N, Nahrig J, et al. Positron emission tomography using [18F]fluorodeoxyglucose for monitoring primary chemotherapy in breast cancer. J Clin Oncol. 2000;18(8):1689-1695.
57 Smith IC, Welch AE, Hutcheon AW, et al. Positron emission tomography using [18F]-fluorodeoxy-D-glucose to predict the pathologic response of breast cancer to primary chemotherapy. J Clin Oncol. 2000;18(8):1676-1688.
58 Weber WA, Ott K, Becker K, et al. Prediction of response to preoperative chemotherapy in adenocarcinomas of the esophagogastric junction by metabolic imaging. J Clin Oncol. 2001;19(12):3058-3065.
59 Unger JB, Ivy JJ, Connor P, et al. Detection of recurrent cervical cancer by whole-body FDG PET scan in asymptomatic and symptomatic women. Gynecol Oncol. 2004;94(1):212-216.
60 Havrilesky LJ, Wong TZ, Secord AA, et al. The role of PET scanning in the detection of recurrent cervical cancer. Gynecol Oncol. 2003;90(1):186-190.
61 Ryu SY, Kim MH, Choi SC, et al. Detection of early recurrence with 18F-FDG PET in patients with cervical cancer. J Nucl Med. 2003;44(3):347-352.
62 Chang SD. Imaging of the vagina and vulva. Radiol Clin North Am. 2002;40(3):637-658.
63 Lamoreaux WT, Grigsby PW, Dehdashti F, et al. FDG-PET evaluation of vaginal carcinoma. Int J Radiat Oncol Biol Phys. 2005;62(3):733-737.
64 Carrington B. The adnexae. In: Hricak H, Carrington BM, editors. MRI of the Pelvis. A Text Atlas. London: Martin Dunitz Ltd; 1991:185.
65 Coakley FV. Staging ovarian cancer: role of imaging. Radiol Clin North Am. 2002;40(3):609-636.
66 Koonings PP, Campbell K, Mishell DRJr, et al. Relative frequency of primary ovarian neoplasms: a 10-year review. Obstet Gynecol. 1989;74(6):921-926.
67 Boente MP, Chi DS, Hoskins WJ. The role of surgery in the management of ovarian cancer: primary and interval cytoreductive surgery. Semin Oncol. 1998;25(3):326-334.
68 National Comprehensive Cancer Network, NCCN clinical practice guidelines in oncology, Ovarian Cancer. V.I.2008; 2008.
69 Castellucci P, Perrone AM, Picchio M, et al. Diagnostic accuracy of 18F-FDG PET/CT in characterizing ovarian lesions and staging ovarian cancer: Correlation with transvaginal ultrasonography, computed tomography, and histology. Nucl Med Commun. 2007;28(8):589-595.
70 Hubner KF, McDonald TW, Niethammer JG, et al. Assessment of primary and metastatic ovarian cancer by positron emission tomography (PET) using 2-[18F]deoxyglucose (2-[18F]FDG). Gynecol Oncol. 1993;51(2):197-204.
71 Kurokawa T, Yoshida Y, Kawahara K, et al. Expression of GLUT-1 glucose transfer, cellular proliferation activity and grade of tumor correlate with [F-18]-fluorodeoxyglucose uptake by positron emission tomography in epithelial tumors of the ovary. Int J Cancer. 2004;109(6):926-932.
72 Yoshida Y, Kurokawa T, Kawahara K, et al. Incremental benefits of FDG positron emission tomography over CT alone for the preoperative staging of ovarian cancer. Am J Roentgenol. 2004;182(1):227-233.
73 Kim CK, Park BK, Choi JY, et al. Detection of recurrent ovarian cancer at MRI: comparison with integrated PET/CT. J Comput Assist Tomogr. 2007;31(6):868-875.
74 Nanni C, Rubello D, Farsad M, et al. (18)F-FDG PET/CT in the evaluation of recurrent ovarian cancer: a prospective study on forty-one patients. Eur J Surg Oncol. 2005;31(7):792-797.
75 Mangili G, Picchio M, Sironi S, et al. Integrated PET/CT as a first-line re-staging modality in patients with suspected recurrence of ovarian cancer. Eur J Nucl Med Mol Imaging. 2007;34(5):658-666.
76 Sebastian S, Lee SI, Horowitz NS, et al. PET-CT vs. CT alone in ovarian cancer recurrence. Abdom Imaging. 2008;33(1):112-118.
77 Garcia-Velloso MJ, Jurado M, Ceamanos C, et al. Diagnostic accuracy of FDG PET in the follow-up of platinum-sensitive epithelial ovarian carcinoma. Eur J Nucl Med Mol Imaging. 2007;34(9):1396-1405.
78 Thrall MM, DeLoia JA, Gallion H, et al. Clinical use of combined positron emission tomography and computed tomography (FDG-PET/CT) in recurrent ovarian cancer. Gynecol Oncol. 2007;105(1):17-22.
79 Torizuka T, Nobezawa S, Kanno T, et al. Ovarian cancer recurrence: role of whole-body positron emission tomography using 2-[fluorine-18]-fluoro-2-deoxy-D-glucose. Eur J Nucl Med Mol. 2002;29(6):797-803.
80 Zimny M, Siggelkow W, Schroder W, et al. 2-[Fluorine-18]-fluoro-2-deoxy-d-glucose positron emission tomography in the diagnosis of recurrent ovarian cancer. Gynecol Oncol. 2001;83(2):310-315.
81 Bristow RE, del Carmen MG, Pannu HK, et al. Clinically occult recurrent ovarian cancer: patient selection for secondary cytoreductive surgery using combined PET/CT. Gynecol Oncol. 2003;90(3):519-528.
82 Sironi S, Messa C, Mangili G, et al. Integrated FDG PET/CT in patients with persistent ovarian cancer: correlation with histologic findings. Radiology. 2004;233(2):433-440.
83 Hauth EA, Antoch G, Stattaus J, et al. Evaluation of integrated whole-body PET/CT in the detection of recurrent ovarian cancer. Eur J Radiol. 2005;56(2):263-268.
84 Murakami M, Miyamoto T, Iida T, et al. Whole-body positron emission tomography and tumor marker CA125 for detection of recurrence in epithelial ovarian cancer. Int J Gynecol Cancer. 2006;16(Suppl 1):99-107.
85 Kubik-Huch RA, Dorffler W, von Schulthess GK, et al. Value of (18F)-FDG positron emission tomography, computed tomography, and magnetic resonance imaging in diagnosing primary and recurrent ovarian carcinoma. Eur Radiol. 2000;10(5):761-767.
86 Simcock B, Neesham D, Quinn M, et al. The impact of PET/CT in the management of recurrent ovarian cancer. Gynecol Oncol. 2006;103(1):271-276.
87 Cohen LS, Escobar PF, Scharm C, et al. Three-dimensional power Doppler ultrasound improves the diagnostic accuracy for ovarian cancer prediction. Gynecol Oncol. 2001;82(1):40-48.
88 Kinkel K, Hricak H, Lu Y, et al. US characterization of ovarian masses: a meta-analysis. Radiology. 2000;217(3):803-811.
89 Rieber A, Nussle K, Stohr I, et al. Preoperative diagnosis of ovarian tumors with MR imaging: comparison with transvaginal sonography, positron emission tomography, and histologic findings. AJR Am J Roentgenol. 2001;177(1):123-129.
90 Hricak H, Chen M, Coakley FV, et al. Complex adnexal masses: detection and characterization with MR imaging—Multivariate analysis. Radiology. 2000;214(1):39-46.
91 Stevens SK, Hricak H, Stern JL. Ovarian lesions: detection and characterization with gadolinium-enhanced MR imaging at 1.5 T. Radiology. 1991;181(2):481-488.
92 Fenchel S, Grab D, Nuessle K, et al. Asymptomatic adnexal masses: correlation of FDG PET and histopathologic findings. Radiology. 2002;223(3):780-788.
93 Grab D, Flock F, Stohr I, et al. Classification of asymptomatic adnexal masses by ultrasound, magnetic resonance imaging, and positron emission tomography. Gynecol Oncol. 2000;77(3):454-459.
94 Nishizawa S, Inubushi M, Okada H. Physiological 18F-FDG uptake in the ovaries and uterus of healthy female volunteers. Eur J Nucl Med Mol Imaging. 2005;32(5):549-556.
95 Spellman CM, Fottrell PF, Baynes S, et al. A study of some enzymes and isoenzymes of carbohydrate metabolism in human endometrium during the menstrual cycle. Clin Chim Acta. 1973;48(3):259-268.
96 Risum S, Hogdall C, Loft A, et al. Prediction of suboptimal primary cytoreduction in primary ovarian cancer with combined positron emission tomography/computed tomography—a prospective study. Gynecol Oncol. 2008;108(2):265-270.
97 Avril N, Sassen S, Schmalfeldt B, et al. Prediction of response to neoadjuvant chemotherapy by sequential F-18-fluorodeoxyglucose positron emission tomography in patients with advanced-stage ovarian cancer. J Clin Oncol. 2005;23(30):7445-7453.
98 Coakley FV, Hricak H. Radiologic anatomy of the prostate gland: A clinical approach. Radiol Clin North Am. 2000;38(1):15-30.
99 Hricak H, Carrington BM. The prostate gland. In: Hricak H, Carrington BM, editors. MRI of the Pelvis. London: Martin Dunitz Ltd; 1991:249.
100 Yu KK, Hricak H. Imaging prostate cancer. Radiol Clin North Am. 2000;38(1):59-85. viii
101 Calvo FA, Domper M, Matute R, et al. 18F-FDG positron emission tomography staging and restaging in rectal cancer treated with preoperative chemoradiation. Int J Radiat Oncol Biol Phys. 2004;58(2):528-535.
102 Hricak H. The seminal vesicles. In: Hricak H, Carrington BM, editors. MRI of the pelvis. London: Martin Dunitz Ltd; 1991:313.
103 Kabalin JN, McNeal JE, Price HM, et al. Unsuspected adenocarcinoma of the prostate in patients undergoing cystoprostatectomy for other causes: incidence, histology and morphometric observations. J Urol. 1989;141(5):1091-1094.
104 Quinlan DM, Partin AW, Walsh PC. Can aggressive prostatic carcinomas be identified and can their natural history be altered by treatment? Urology. 1995;46(3 Suppl A):77-82.
105 Shinohara K, Scardino PT, Carter SS, et al. Pathologic basis of the sonographic appearance of the normal and malignant prostate. Urol Clin North Am. 1989;16(4):675-691.
106 Shinohara K, Wheeler TM, Scardino PT. The appearance of prostate cancer on transrectal ultrasonography: correlation of imaging and pathological examinations. J Urol. 1989;142(1):76-82.
107 Schnall MD, Imai Y, Tomaszewski J, et al. Prostate cancer: local staging with endorectal surface coil MR imaging. Radiology. 1991;178(3):797-802.
108 Yu KK, Hricak H, Alagappan R, et al. Detection of extracapsular extension of prostate carcinoma with endorectal and phased-array coil MR imaging: Multivariate feature analysis. Radiology. 1997;202(3):697-702.
109 Scheidler J, Hricak H, Vigneron DB, et al. Prostate cancer: Localization with three-dimensional proton MR spectroscopic imaging—clinicopathologic study. Radiology. 1999;213(2):473-480.
110 Wefer AE, Hricak H, Vigneron DB, et al. Sextant localization of prostate cancer: comparison of sextant biopsy, magnetic resonance imaging and magnetic resonance spectroscopic imaging with step section histology. J Urol. 2000;164(2):400-404.
111 Yu KK, Scheidler J, Hricak H, et al. Prostate cancer: prediction of extracapsular extension with endorectal MR imaging and three-dimensional proton MR spectroscopic imaging. Radiology. 1999;213(2):481-488.
112 National Comprehensive Cancer Network, NCCN clinical practice guidelines in oncology, Prostate Cancer. V.I.2008; 2008.
113 Effert PJ, Bares R, Handt S, et al. Metabolic imaging of untreated prostate cancer by positron emission tomography with sup 18 fluorine-labeled deoxyglucose. J Urol. 1996;155(3):994-998.
114 Shreve PD, Grossman HB, Gross MD, et al. Metastatic prostate cancer: initial findings of PET with 2-deoxy-2-[F-18]fluoro-D-glucose. Radiology. 1996;199(3):751-756.
115 Schöder H, Herrmann K, Gonen M, et al. 2-[18F]fluoro-2-deoxyglucose positron emission tomography for the detection of disease in patients with prostate-specific antigen relapse after radical prostatectomy. Clin Cancer Res. 2005;11(13):4761-4769.
116 Seltzer MA, Barbaric Z, Belldegrun A, et al. Comparison of helical computerized tomography, positron emission tomography and monoclonal antibody scans for evaluation of lymph node metastases in patients with prostate specific antigen relapse after treatment for localized prostate cancer. J Urol. 1999;162(4):1322-1328.
117 Oyama N, Akino H, Suzuki Y, et al. Prognostic value of 2-deoxy-2-[F-18]fluoro-D-glucose positron emission tomography imaging for patients with prostate cancer. Mol Imaging Biol. 2002;4(1):99-104.
118 Robbins RJ, Wan Q, Grewal RK, et al. Real-time prognosis for metastatic thyroid carcinoma based on 2-[18F]fluoro-2-deoxy-D-glucose-positron emission tomography scanning. J Clin Endocrinol Metab. 2006;91(2):498-505.
119 Schoder H, Noy A, Gonen M, et al. Intensity of 18fluorodeoxyglucose uptake in positron emission tomography distinguishes between indolent and aggressive non-Hodgkin’s lymphoma. J Clin Oncol. 2005;23(21):4643-4651.
120 Agus DB, Golde DW, Sgouros G, et al. Positron emission tomography of a human prostate cancer xenograft: association of changes in deoxyglucose accumulation with other measures of outcome following androgen withdrawal. Cancer Res. 1998;58(14):3009-3014.
121 Oyama N, Kim J, Jones LA, et al. MicroPET assessment of androgenic control of glucose and acetate uptake in the rat prostate and a prostate cancer tumor model. Nucl Med Biol. 2002;29(8):783-790.
122 Hofer C, Laubenbacher C, Block T, et al. Fluorine-18-fluorodeoxyglucose positron emission tomography is useless for the detection of local recurrence after radical prostatectomy. Eur Urol. 1999;36(1):31-35.
123 Liu IJ, Zafar MB, Lai YH, et al. Fluorodeoxyglucose positron emission tomography studies in diagnosis and staging of clinically organ-confined prostate cancer. Urology. 2001;57(1):108-111.
124 Sanz G, Robles JE, Giménez M, et al. Positron emission tomography with 18fluorine-labelled deoxyglucose: utility in localized and advanced prostate cancer. BJU Int. 1999;84(9):1028-1031.
125 Bucerius J, Ahmadzadehfar H, Hortling N, et al. Incidental diagnosis of a PSA-negative prostate cancer by 18FDG PET/CT in a patient with hypopharyngeal cancer. Prostate Cancer Prostatic Dis. 2007;10(3):307-310.
126 Heicappell R, Muller-Mattheis V, Reinhardt M, et al. Staging of pelvic lymph nodes in neoplasms of the bladder and prostate by positron emission tomography with 2-[(18)F]-2-deoxy-D-glucose. Eur Urol. 1999;36(6):582-587.
127 Morris MJ, Akhurst T, Osman I, et al. Fluorinated deoxyglucose positron emission tomography imaging in progressive metastatic prostate cancer. Urology. 2002;59(6):913-918.
128 Ulbright T, Amin MB, Young RH. Tumors of the testis, adenxa, spermatic cord and scrotum. Atlas of Tumor Pathology, Fasc 25, Ser 3. Washington DC: Armed Forces Institute of Pathology; 1999. p 1
129 Schwerk WB, Schwerk WN, Rodeck G. Testicular tumors: prospective analysis of real-time US patterns and abdominal staging. Radiology. 1987;164(2):369-374.
130 Rifkin MD, Kurtz AB, Pasto ME, et al. Diagnostic capabilities of high-resolution scrotal ultrasonography: prospective evaluation. J Ultrasound Med. 1985;4(1):13-19.
131 Serra AD, Hricak H, Coakley FV, et al. Inconclusive clinical and ultrasound evaluation of the scrotum: Impact of magnetic resonance imaging on patient management and cost. Urology. 1998;51(6):1018-1021.
132 Hricak H. The testis. In: Hricak H, Carrington BM, editors. MRI of the pelvis: a text atlas. London: Martin Dunitz Ltd; 1991:343.
133 National Comprehensive Cancer Network, NCCN clinical practice guidelines in oncology, Testicular Cancer. V.I.2008; 2008.
134 Ulbright TM. Germ cell tumors of the gonads: a selective review emphasizing problems in differential diagnosis, newly appreciated, and controversial issues. Mod Pathol. 2005;18(Suppl 2):S61-79.
135 Cremerius U, Effert PJ, Adam G, et al. FDG PET for detection and therapy control of metastatic germ cell tumor. J Nucl Med. 1998;39(5):815-822.
136 Cremerius U, Wildberger JE, Borchers H, et al. Does positron emission tomography using 18-fluoro-2-deoxyglucose improve clinical staging of testicular cancer? Results of a study in 50 patients. Urology. 1999;54(5):900-904.
137 Hain SF, O’Doherty MJ, Timothy AR, et al. Fluorodeoxyglucose PET in the initial staging of germ cell tumours. Eur J Nucl Med. 2000;27(5):590-594.
138 Nuutinen JM, Leskinen S, Elomaa I, et al. Detection of residual tumours in postchemotherapy testicular cancer by FDG-PET. Eur J Cancer. 1997;33(8):1234-1241.
139 De Santis M, Bokemeyer C, Becherer A, et al. Predictive impact of 2-18fluoro-2-deoxy-D-glucose positron emission tomography for residual postchemotherapy masses in patients with bulky seminoma. J Clin Oncol. 2001;19(17):3740-3744.
140 Kollmannsberger C, Oechsle K, Dohmen BM, et al. Prospective comparison of [18F]fluorodeoxyglucose positron emission tomography with conventional assessment by computed tomography scans and serum tumor markers for the evaluation of residual masses in patients with nonseminomatous germ cell carcinoma. Cancer. 2002;94(9):2353-2362.
141 Krege S, Beyer J, Souchon R, et al. European consensus conference on diagnosis and treatment of germ cell cancer: a report of the second meeting of the European Germ Cell Cancer Consensus group (EGCCCG): part I. Eur Urol. 2008;53(3):478-496.
142 de Wit M, Hartmann M, Kotzerke J, et al. German Multicenter PETSG. [18F]-FDG-PET in clinical stage I and II non-seminomatous germ cell tumors: first results of the German Multicenter Trial. J Clin Oncol (Meeting Abstracts). 2005;23(Suppl 16):4504.
143 Lassen U, Daugaard G, Eigtved A, et al. Whole-body FDG-PET in patients with stage I non-seminomatous germ cell tumours. Eur J Nucl Med Mol Imaging. 2003;30(3):396-402.
144 Huddart RA, O’Doherty MJ, Padhani A, et al. 18-Fluorodeoxyglucose positron emission tomography in the prediction of relapse in patients with high-risk, clinical stage I nonseminomatous germ cell tumors: preliminary report of MRC Trial TE22—the NCRI Testis Tumour Clinical Study Group. J Clin Oncol. 2007;25(21):3090-3095.
145 Albers P, Bender H, Yilmaz H, et al. Positron emission tomography in the clinical staging of patients with Stage I and II testicular germ cell tumors. Urology. 1999;53(4):808-811.
146 Tsatalpas P, Beuthien-Baumann B, Kropp J, et al. Diagnostic value of 18F-FDG positron emission tomography for detection and treatment control of malignant germ cell tumors. Urol Int. 2002;68(3):157-163.
147 Rustin GJ, Mead GM, Stenning SP, et al. Randomized trial of two or five computed tomography scans in the surveillance of patients with stage I nonseminomatous germ cell tumors of the testis: Medical Research Council Trial TE08, ISRCTN56475197—the National Cancer Research Institute Testis Cancer Clinical Studies Group. J Clin Oncol. 2007;25(11):1310-1315.
148 Becherer A, Santis MD, Karanikas G, et al. FDG PET is superior to CT in the prediction of viable tumour in post-chemotherapy seminoma residuals. Eur J Radiol. 2005;54(2):284-288.
149 Lewis DA, Tann M, Kesler K, et al. positron emission tomography scans in postchemotherapy seminoma patients with residual masses: a retrospective review from Indiana University Hospital. J Clin Oncol. 2006;24(34):e54-55.
150 Stephens AW, Gonin R, Hutchins GD, et al. Positron emission tomography evaluation of residual radiographic abnormalities in postchemotherapy germ cell tumor patients. J Clin Oncol. 1996;14(5):1637-1641.
151 Sugawara Y, Zasadny KR, Grossman HB, et al. Germ cell tumor: differentiation of viable tumor, mature teratoma, and necrotic tissue with FDG PET and kinetic modeling. Radiology. 1999;211(1):249-256.
152 Pfannenberg AC, Oechsle K, Bokemeyer C, et al. The role of [(18)F] FDG-PET, CT/MRI and tumor marker kinetics in the evaluation of post chemotherapy residual masses in metastatic germ cell tumors—prospects for management. World J Urol. 2004;22(2):132-139.
153 Haba Y, Williams MV, Neal DE, et al. Stage migration and pilot studies of reduced chemotherapy supported by positron-emission tomography findings suggest new combined strategies for stage 2 nonseminoma germ cell tumour. BJU Int. 2008;101(5):570-574.
154 Hricak H. The penis and male urethra. In: Hricak H, Carrington BM, editors. MRI of the pelvis. London: Martin Dunitz Ltd; 1991:383.
155 Scher B, Seitz M, Reiser M, et al. 18F-FDG PET/CT for staging of penile cancer. J Nucl Med. 2005;46(9):1460-1465.
156 Hricak H. The bladder and female urethra. In: Hricak H, Carrington BM, editors. MRI of the pelvis. London: Martin Dunitz Ltd; 1991:417.
157 Barentsz JO, Jager GJ, Witjes JA, et al. Primary staging of urinary bladder carcinoma: The role of MRI and a comparison with CT. Eur Radiol. 1996;6(2):129-133.
158 Barentsz JO, Ruijs SH, Strijk SP. The role of MR imaging in carcinoma of the urinary bladder. AJR Am J Roentgenol. 1993;160(5):937-947.
159 Husband JE, Olliff JF, Williams MP, et al. Bladder cancer: staging with CT and MR imaging. Radiology. 1989;173(2):435-440.
160 Kim B, Semelka RC, Ascher SM, et al. Bladder tumor staging: comparison of contrast-enhanced CT, T1- and T2-weighted MR imaging, dynamic gadolinium-enhanced imaging, and late gadolinium-enhanced imaging. Radiology. 1994;193(1):239-245.
161 Narumi Y, Kumatani T, Sawai Y, et al. The bladder and bladder tumors: imaging with three-dimensional display of helical CT data. AJR Am J Roentgenol. 1996;167(5):1134-1135.
162 Persad R, Kabala J, Gillatt D, et al. Magnetic resonance imaging in the staging of bladder cancer. Br J Urol. 1993;71(5):566-573.
163 Tanimoto A, Yuasa Y, Imai Y, et al. Bladder tumor staging: comparison of conventional and gadolinium-enhanced dynamic MR imaging and CT. Radiology. 1992;185(3):741-747.
164 National Comprehensive Cancer Network, NCCN clinical practice guidelines in oncology, Bladder cancer. V.I.2008; 2008.
165 Koyama K, Okamura T, Kawabe J, et al. Evaluation of 18F-FDG PET with bladder irrigation in patients with uterine and ovarian tumors. J Nucl Med. 2003;44(3):353-358.
166 Anjos DA, Etchebehere EC, Ramos CD, et al. 18F-FDG PET/CT delayed images after diuretic for restaging invasive bladder cancer. J Nucl Med. 2007;48(5):764-770.
167 Kamel EM, Jichlinski P, Prior JO, et al. Forced diuresis improves the diagnostic accuracy of 18F-FDG PET in abdominopelvic malignancies. J Nucl Med. 2006;47(11):1803-1807.
168 Purcell DD, Coakley FV, Franc BL, et al. Anterior layering of excreted 18F-FDG in the bladder on PET/CT: frequency and cause. Am J Roentgenol. 2007;189(2):W96-99.
169 Bachor R, Kotzerke J, Reske SN, et al. [Lymph node staging of bladder neck carcinoma with positron emission tomography]. Urologe A. 1999;38(1):46-50.
170 Kosuda S, Kison PV, Greenough R, et al. Preliminary assessment of fluorine-18 fluorodeoxyglucose positron emission tomography in patients with bladder cancer. Eur J Nucl Med. 1997;24(6):615-620.
171 Liu IJ, Lai YH, Espiritu JI, et al. Evaluation of fluorodeoxyglucose positron emission tomography imaging in metastatic transitional cell carcinoma with and without prior chemotherapy. Urol Int. 2006;77(1):69-75.
172 Hricak H, Carrington BM. The rectum and sigmoid colon. In: Hricak H, Carrington BM, editors. MRI of the Pelvis. London: Martin Dunitz Ltd; 1991:463.
173 Fazio VW, Tjandra JJ. Primary therapy of carcinoma of the large bowel. World J Surg. 1991;15(5):568-575.
174 Izbicki JR, Blochle C. Colorectal carcinoma: impact of staging on surgical treatment. Endoscopy. 1993;25(1):117-124.
175 Kantorova I, Lipska L, Belohlavek O, et al. Routine (18)F-FDG PET preoperative staging of colorectal cancer: comparison with conventional staging and its impact on treatment decision making. J Nucl Med. 2003;44(11):1784-1788.
176 Abdel-Nabi H, Doerr RJ, Lamonica DM, et al. Staging of primary colorectal carcinomas with fluorine-18 fluorodeoxyglucose whole-body PET: correlation with histopathologic and CT findings. Radiology. 1998;206(3):755-760.
177 Schiepers C, Haustermans K, Geboes K, et al. The effect of preoperative radiation therapy on glucose utilization and cell kinetics in patients with primary rectal carcinoma. Cancer. 1999;85(4):803-811.
178 Akhurst T, Kates TJ, Mazumdar M, et al. Recent chemotherapy reduces the sensitivity of [18F]fluorodeoxyglucose positron emission tomography in the detection of colorectal metastases. J Clin Oncol. 2005;23(34):8713-8716.
179 Whiteford MH, Whiteford HM, Yee LF, et al. Usefulness of FDG-PET scan in the assessment of suspected metastatic or recurrent adenocarcinoma of the colon and rectum. Dis Colon Rectum. 2000;43(6):759-767.
180 Blomqvist L, Holm T, Rubio C, et al. Rectal tumours—MR imaging with endorectal and/or phased-array coils, and histopathological staging on giant sections. A comparative study. Acta Radiol. 1997;38(3):437-444.
181 Chan TW, Kressel HY, Milestone B, et al. Rectal carcinoma: staging at MR imaging with endorectal surface coil. Work in progress. Radiology. 1991;181(2):461-467.
182 Gualdi GF, Casciani E, Guadalaxara A, et al. Local staging of rectal cancer with transrectal ultrasound and endorectal magnetic resonance imaging: comparison with histologic findings. Dis Colon Rectum. 2000;43(3):338-345.
183 Indinnimeo M, Grasso RF, Cicchini C, et al. Endorectal magnetic resonance imaging in the preoperative staging of rectal tumors. Int Surg. 1996;81(4):419-422.
184 Kim NK, Kim MJ, Yun SH, et al. Comparative study of transrectal ultrasonography, pelvic computerized tomography, and magnetic resonance imaging in preoperative staging of rectal cancer. Dis Colon Rectum. 1999;42(6):770-775.
185 Maldjian C, Smith R, Kilger A, et al. Endorectal surface coil MR imaging as a staging technique for rectal carcinoma: a comparison study to rectal endosonography. Abdom Imaging. 2000;25(1):75-80.
186 Schnall MD, Furth EE, Rosato EF, et al. Rectal tumor stage: correlation of endorectal MR imaging and pathologic findings. Radiology. 1994;190(3):709-714.
187 Zagoria RJ, Schlarb CA, Ott DJ, et al. Assessment of rectal tumor infiltration utilizing endorectal MR imaging and comparison with endoscopic rectal sonography. J Surg Oncol. 1997;64(4):312-317.
188 National Comprehensive Cancer Network, NCCN clinical practice guidelines in oncology, Rectal Cancer. V.I.2008; 2008.
189 Anderson C, Koshy M, Staley C, et al. PET-CT fusion in radiation management of patients with anorectal tumors. Int J Radiat Oncol Biol Phys. 2007;69(1):155-162.
190 Heriot AG, Hicks RJ, Drummond EG, et al. Does positron emission tomography change management in primary rectal cancer? A prospective assessment. Dis Colon Rectum. 2004;47(4):451-458.
191 Gearhart S, Frassica D, Rosen R, et al. Improved staging with pretreatment positron emission tomography/computed tomography in low rectal cancer. Ann Surg Oncol. 2006;13(3):397-404.
192 Kalff V, Duong C, Drummond EG, et al. Findings on 18F-FDG PET scans after neoadjuvant chemoradiation provides prognostic stratification in patients with locally advanced rectal carcinoma subsequently treated by radical surgery. J Nucl Med. 2006;47(1):14-22.
193 Rappeport ED, Loft A, Berthelsen AK, et al. Contrast-enhanced FDG-PET/CT vs. SPIO-enhanced MRI vs. FDG-PET vs. CT in patients with liver metastases from colorectal cancer: a prospective study with intraoperative confirmation. Acta Radiol. 2007;48(4):369-378.
194 Patel DA, Chang ST, Goodman KA, et al. Impact of integrated PET/CT on variability of target volume delineation in rectal cancer. Technol Cancer Res Treat. 2007;6(1):31-36.
195 Black QC, Grills IS, Kestin LL, et al. Defining a radiotherapy target with positron emission tomography. Int J Radiat Oncol Biol Phys. 2004;60(4):1272-1282.
196 Nestle U, Kremp S, Schaefer-Schuler A, et al. Comparison of different methods for delineation of 18F-FDG PET-positive tissue for target volume definition in radiotherapy of patients with non-small cell lung cancer. J Nucl Med. 2005;46(8):1342-1348.
197 Roels S, Haustermans K, Gregoire V, et al, Automated functional image-guided radiation treatment planning for rectal cancer, Int J Radiat Oncol Biol Phys 62:893–900, 2005;, In regard to Ciernik et al.; Int J Radiat Oncol Biol Phys; 64. 5; 2006:1611-1612.
198 Daisne JF, Sibomana M, Bol A, et al. Tri-dimensional automatic segmentation of PET volumes based on measured source-to-background ratios: influence of reconstruction algorithms. Radiother Oncol. 2003;69(3):247-250.
199 Daisne JF, Duprez T, Weynand B, et al. Tumor volume in pharyngolaryngeal squamous cell carcinoma: comparison at CT, MR imaging, and FDG PET and validation with surgical specimen. Radiology. 2004;233(1):93-100.
200 Nahas CS, Akhurst T, Yeung H, et al. Positron emission tomography detection of distant metastatic or synchronous disease in patients with locally advanced rectal cancer receiving preoperative chemoradiation. Ann Surg Oncol. 2008;15(3):704-711.
201 Jemal A, Siegel R, Ward E, et al. Cancer statistics, 2006. CA Cancer J Clin. 2006;56(2):106-130.
202 Even-Sapir E, Lerman H, Figer A, et al. Role of (18)F-FDG dual-head gamma-camera coincidence imaging in recurrent or metastatic colorectal carcinoma. J Nucl Med. 2002;43(5):603-609.
203 Kinkel K, Lu Y, Both M, et al. Detection of hepatic metastases from cancers of the gastrointestinal tract by using noninvasive imaging methods (US, CT, MR imaging, PET): A meta-analysis. Radiology. 2002;224(3):748-756.
204 Fong Y, Saldinger PF, Akhurst T, et al. Utility of 18F-FDG positron emission tomography scanning on selection of patients for resection of hepatic colorectal metastases. Am J Surg. 1999;178(4):282-287.
205 Ruers TJ, Langenhoff BS, Neeleman N, et al. Value of positron emission tomography with [F-18]fluorodeoxyglucose in patients with colorectal liver metastases: a prospective study. J Clin Oncol. 2002;20(2):388-395.
206 Boykin KN, Zibari GB, Lilien DL, et al. The use of FDG-positron emission tomography for the evaluation of colorectal metastases of the liver. Am Surg. 1999;65(12):1183-1185.
207 Lai DT, Fulham M, Stephen MS, et al. The role of whole-body positron emission tomography with [18F]fluorodeoxyglucose in identifying operable colorectal cancer metastases to the liver. Arch Surg. 1996;131(7):703-707.
208 Fernandez FG, Drebin JA, Linehan DC, et al. Five-year survival after resection of hepatic metastases from colorectal cancer in patients screened by positron emission tomography with F-18 fluorodeoxyglucose (FDG-PET). Ann Surg. 2004;240(3):438-447.
209 Bellomi M, Rizzo S, Travaini LL, et al. Role of multidetector CT and FDG-PET/CT in the diagnosis of local and distant recurrence of resected rectal cancer. Radiol Med (Torino). 2007;112(5):681-690.
210 Guillem JG, Chessin DB, Cohen AM, et al. Long-term oncologic outcome following preoperative combined modality therapy and total mesorectal excision of locally advanced rectal cancer. Ann Surg. 2005;241(5):829-836.
211 Chessin DB, Kiran RP, Akhurst T, et al. The emerging role of 18F-fluorodeoxyglucose positron emission tomography in the management of primary and recurrent rectal cancer. J Am Coll Surg. 2005;201(6):948-956.
212 Guillem JG, Chessin DB, Shia J, et al. Clinical examination following preoperative chemoradiation for rectal cancer is not a reliable surrogate end point. J Clin Oncol. 2005;23(15):3475-3479.
213 Guillem JG, Puig-La Calle JJr, Akhurst T, et al. Prospective assessment of primary rectal cancer response to preoperative radiation and chemotherapy using 18-fluorodeoxyglucose positron emission tomography. Dis Colon Rectum. 2000;43(1):18-24.
214 Hoffmann KT, Rau B, Wust P, et al. Restaging of locally advanced carcinoma of the rectum with MR imaging after preoperative radio-chemotherapy plus regional hyperthermia. Strahlenther Onkol. 2002;178(7):386-392.
215 Kim HJ, Wong WD. Role of endorectal ultrasound in the conservative management of rectal cancers. Semin Surg Oncol. 2000;19(4):358-366.
216 Amthauer H, Denecke T, Rau B, et al. Response prediction by FDG-PET after neoadjuvant radiochemotherapy and combined regional hyperthermia of rectal cancer: correlation with endorectal ultrasound and histopathology. Eur J Nucl Med Mol Imaging. 2004;31(6):811-819.
217 Capirci C, Rampin L, Erba PA, et al. Sequential FDG-PET/CT reliably predicts response of locally advanced rectal cancer to neo-adjuvant chemo-radiation therapy. Eur J Nucl Med Mol Imaging. 2007;34(10):1583-1593.
218 Cascini GL, Avallone A, Delrio P, et al. 18F-FDG PET is an early predictor of pathologic tumor response to preoperative radiochemotherapy in locally advanced rectal cancer. J Nucl Med. 2006;47(8):1241-1248.
219 Melton GB, Lavely WC, Jacene HA, et al. Efficacy of preoperative combined 18-fluorodeoxyglucose positron emission tomography and computed tomography for assessing primary rectal cancer response to neoadjuvant therapy. J Gastrointest Surg. 2007;11(8):961-969.
220 Guillem JG, Moore HG, Akhurst T, et al. Sequential preoperative fluorodeoxyglucose-positron emission tomography assessment of response to preoperative chemoradiation: a means for determining longterm outcomes of rectal cancer. J Am Coll Surg. 2004;199(1):1-7.
221 Kristiansen C, Loft A, Berthelsen A, et al. PET/CT and histopathologic response to preoperative chemoradiation therapy in locally advanced rectal cancer. Dis Colon Rectum. 2008;51(1):21-25.
222 Bouzourene H, Bosman FT, Seelentag W, et al. Importance of tumor regression assessment in predicting the outcome in patients with locally advanced rectal carcinoma who are treated with preoperative radiotherapy. Cancer. 2002;94(4):1121-1130.
223 Engenhart R, Kimmig BN, Strauss LG, et al. Therapy monitoring of presacral recurrences after high-dose irradiation: value of PET, CT, CEA and pain score. Strahlenther Onkol. 1992;168(4):203-212.
224 Shia J, Guillem JG, Moore HG, et al. Patterns of morphologic alteration in residual rectal carcinoma following preoperative chemoradiation and their association with long-term outcome. Am J Surg Pathol. 2004;28(2):215-223.
225 Desch CE, Benson AB3rd, Somerfield MR, et al. Colorectal cancer surveillance: 2005 update of an American Society of Clinical Oncology practice guideline. J Clin Oncol. 2005;23(33):8512-8519.
226 Tveit KM, Kataja VV. ESMO minimum clinical recommendations for diagnosis, treatment and follow-up of rectal cancer. Ann Oncol. 2005;16(Suppl 1):i20-21.
227 Carriquiry LA, Pineyro A. Should carcinoembryonic antigen be used in the management of patients with colorectal cancer? Dis Colon Rectum. 1999;42(7):921-929.
228 Mitchell EP. Role of carcinoembryonic antigen in the management of advanced colorectal cancer. Semin Oncol. 1998;25(5 Suppl 11):12-20.
229 Flamen P, Hoekstra OS, Homans F, et al. Unexplained rising carcinoembryonic antigen (CEA) in the postoperative surveillance of colorectal cancer: The utility of positron emission tomography (PET). Eur J Cancer. 2001;37(7):862-869.
230 Selzner M, Hany TF, Wildbrett P, et al. Does the novel PET/CT imaging modality impact on the treatment of patients with metastatic colorectal cancer of the liver? Ann Surg. 2004;240(6):1027-1034.
231 Simo M, Lomena F, Setoain J, et al. FDG-PET improves the management of patients with suspected recurrence of colorectal cancer. Nucl Med Commun. 2002;23(10):975-982.
232 Staib L, Schirrmeister H, Reske SN, et al. Is (18)F-fluorodeoxyglucose positron emission tomography in recurrent colorectal cancer a contribution to surgical decision making? Am J Surg. 2000;180(1):1-5.
233 Flanagan FL, Dehdashti F, Ogunbiyi OA, et al. Utility of FDG-PET for investigating unexplained plasma CEA elevation in patients with colorectal cancer. Ann Surg. 1998;227(3):319-323.
234 Libutti SK, Alexander HRJr, Choyke P, et al. A prospective study of 2-[18F] fluoro-2-deoxy-D-glucose/positron emission tomography scan, 99mTc-labeled arcitumomab (CEA-scan), and blind second-look laparotomy for detecting colon cancer recurrence in patients with increasing carcinoembryonic antigen levels. Ann Surg Oncol. 2001;8(10):779-786.
235 Maldonado A, Sancho F, Cerdan J, et al. 16. FDG-PET in the detection of recurrence in colorectal cancer based on rising CEA level. Experience in 72 patients. Clin Positron Imaging. 2000;3(4):170.
236 Valk PE, Abella-Columna E, Haseman MK, et al. Whole-body PET imaging with [18F]fluorodeoxyglucose in management of recurrent colorectal cancer. Arch Surg. 1999;134(5):503-511.
237 Arulampalam T, Costa D, Visvikis D, et al. The impact of FDG-PET on the management algorithm for recurrent colorectal cancer. Eur J Nucl Med. 2001;28(12):1758-1765.
238 Delbeke D, Vitola JV, Sandler MP, et al. Staging recurrent metastatic colorectal carcinoma with PET. J Nucl Med. 1997;38(8):1196-1201.
239 Lonneux M, Reffad AM, Detry R, et al. FDG-PET improves the staging and selection of patients with recurrent colorectal cancer. Eur J Nucl Med Mol Imaging. 2002;29(7):915-921.
240 Ogunbiyi OA, Flanagan FL, Dehdashti F, et al. Detection of recurrent and metastatic colorectal cancer: Comparison of positron emission tomography and computed tomography. Ann Surg Oncol. 1997;4(8):613-620.
241 Vitola JV, Delbeke D, Sandler MP, et al. Positron emission tomography to stage suspected metastatic colorectal carcinoma to the liver. Am J Surg. 1996;171(1):21-26.
242 Minton JP, Hoehn JL, Gerber DM, et al. Results of a 400-patient carcinoembryonic antigen second-look colorectal cancer study. Cancer. 1985;55(6):1284-1290.
243 Sardi A, Nieroda CA, Siddiqi MA, et al. Carcinoembryonic antigen directed multiple surgical procedures for recurrent colon cancer confined to the liver. Am Surg. 1990;56(4):255-259.
244 Martin EWJr, Minton JP, Carey LC. CEA-directed second-look surgery in the asymptomatic patient after primary resection of colorectal carcinoma. Ann Surg. 1985;202(3):310-317.
245 Moore HG, Akhurst T, Larson SM, et al. A case-controlled study of 18-fluorodeoxyglucose positron emission tomography in the detection of pelvic recurrence in previously irradiated rectal cancer patients. J Am Coll Surg. 2003;197(1):22-28.
246 Cohade C, Osman M, Leal J, et al. Direct comparison of (18)F-FDG PET and PET/CT in patients with colorectal carcinoma. J Nucl Med. 2003;44(11):1797-1803.
247 Even-Sapir E, Parag Y, Lerman H, et al. Detection of recurrence in patients with rectal cancer: PET/CT after abdominoperineal or anterior resection. Radiology. 2004;232(3):815-822.
248 Fukunaga H, Sekimoto M, Ikeda M, et al. Fusion image of positron emission tomography and computed tomography for the diagnosis of local recurrence of rectal cancer. Ann Surg Oncol. 2005;12(7):561-569.
249 Kim J-H, Czernin J, Allen-Auerbach MS, et al. Comparison between 18F-FDG PET, in-line PET/CT, and software fusion for restaging of recurrent colorectal cancer. J Nucl Med. 2005;46(4):587-595.
250 von Schulthess GK, Steinert HC, Hany TF. Integrated PET/CT: Current applications and future directions. Radiology. 2006;238(2):405-422.