Chapter 75 Patterns of Genetic Transmission
Family History and Pedigree Notation
A pedigree provides a graphic depiction of a family’s structure and medical history. It is important when taking a pedigree to be systematic and use standard symbols and configurations (Figs. 75-1, 75-2, 75-3, 75-4) so that anyone can read and understand the information. In the pediatric setting, the proband is typically the child or adolescent who is being evaluated. The proband is designated in the pedigree by an arrow.
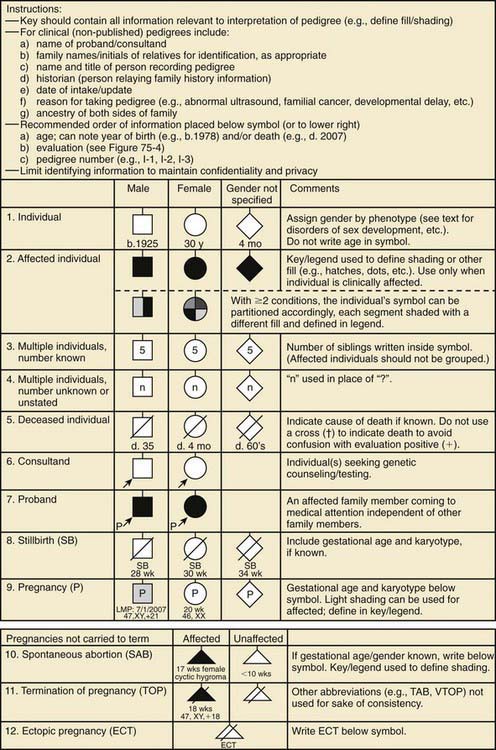
Figure 75-1 Common pedigree symbols, definitions, and abbreviations.
(From Bennett RL, French KS, Resta RG, et al: Standardized human pedigree nomenclature: update and assessment of the recommendations of the National Society of Genetic Counselors, J Genet Counsel 17:424–433, 2008.)
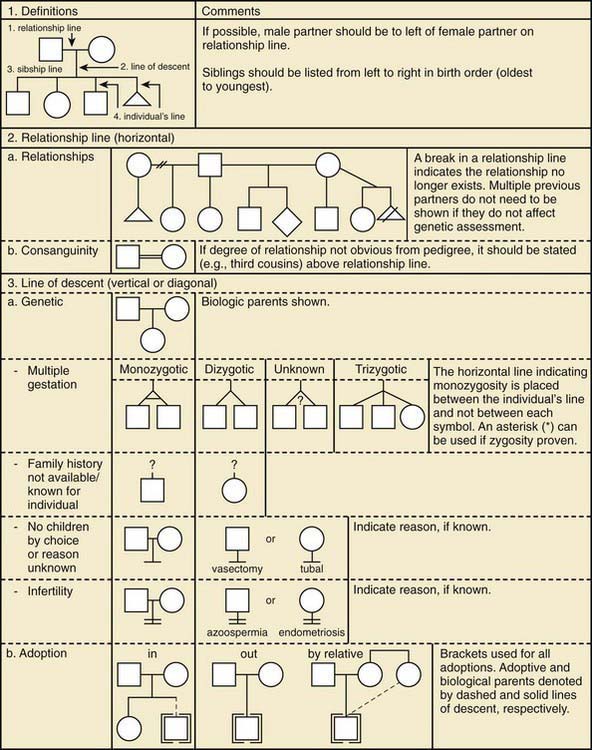
Figure 75-2 Pedigree line definitions.
(From Bennett RL, French KS, Resta RG, et al: Standardized human pedigree nomenclature: update and assessment of the recommendations of the National Society of Genetic Counselors, J Genet Counsel 17:424–433, 2008.)
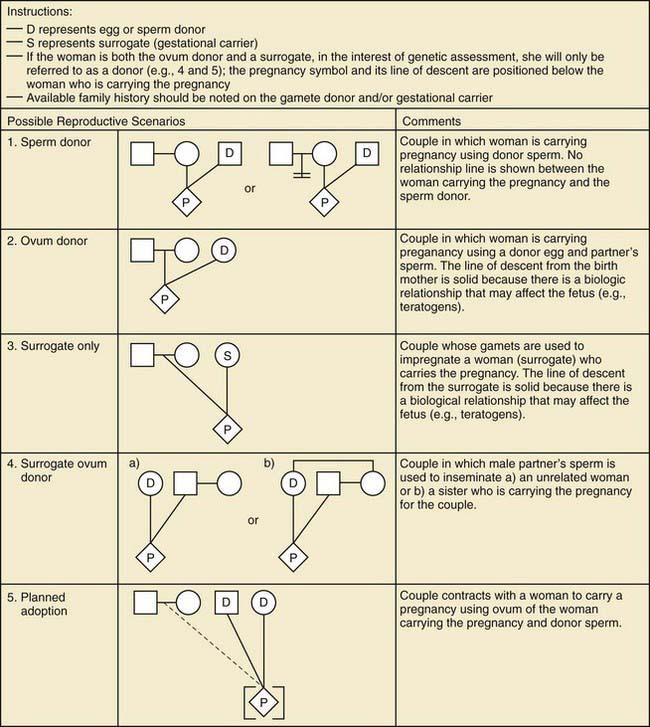
Figure 75-3 Assisted reproductive technology symbols and definitions.
(From Bennett RL, French KS, Resta RG, et al: Standardized human pedigree nomenclature: update and assessment of the recommendations of the National Society of Genetic Counselors, J Genet Counsel 17:424–433, 2008.)
Mendelian Inheritance
Autosomal Dominant Inheritance
The pedigree for an autosomal dominant disorder (Fig. 75-5) demonstrates certain characteristics. The disorder is transmitted in a vertical (parent to child) pattern and can appear in multiple generations. This is illustrated by individual I.1 (see Fig 75-5) passing on the changed gene to II.2 and II.5. An affected individual has a 50% (1 in 2) chance of passing on the deleterious gene in each pregnancy and, therefore, of having a child affected by the disorder. This is referred to as the recurrence risk for the disorder. Unaffected individuals (family members who do not manifest the trait) do not pass the disorder to their children. Males and females are equally affected. Although not a characteristic per se, the finding of male-to-male transmission essentially confirms autosomal dominant inheritance. Vertical transmission can also be seen with X-linked traits. However, because a father passes on his Y chromosome to a son, male-to-male transmission cannot be seen with an X-linked trait. Therefore, male-to-male transmission eliminates X-linked inheritance as a possible explanation. Although male-to-male transmission can occur with Y-linked genes as well, there are very few Y-linked disorders compared with thousands having the autosomal dominant inheritance pattern.
Although parent to child transmission is a characteristic of autosomal dominant inheritance, for many patients with an autosomal dominant disorder there is no history of an affected family member. There are several possible reasons: First, the patient may represent a new mutation that occurred in the DNA of the egg or sperm that came together to form that individual. Second, many autosomal dominant conditions demonstrate incomplete penetrance, meaning that not all individuals who carry the mutation have phenotypic manifestations. In a pedigree this can appear as a skipped generation, in which an unaffected individual links two affected persons (Fig. 75-6). There are many potential reasons that a disorder exhibits incomplete penetrance, including the effect of modifier genes, environmental factors, gender, and age. Third, individuals with the same autosomal dominant mutation can manifest the disorder to different degrees. This is termed variable expression and is a characteristic of many autosomal dominant disorders. Fourth, some spontaneous genetic mutations occur not in the egg or sperm that forms a child but rather in a cell in the developing embryo. Such events are referred to as somatic mutations, and because not all cells are affected, the change is said to be mosaic. The resulting phenotype caused by a somatic mutation can be varied, but it is usually milder than if all cells contain the mutation. In germline mosaicism, the mutation occurs in cells that populate the germline that produce eggs or sperm. A germline mosaic might not have any manifestations of the disorder but might produce multiple eggs or sperm that carry the mutation.
Autosomal Recessive Inheritance
Autosomal recessive inheritance involves mutations in both copies of a gene. Examples of autosomal recessive diseases are cystic fibrosis and sickle cell disease. Characteristics of autosomal recessive traits (Fig. 75-7) include horizontal transmission, the observation of multiple affected members of a kindred in the same generation, but no affected family members in other generations; recurrence risk of 25% for parents with a previous affected child; males and females being equally affected, although some traits exhibit different expression in males and females and increased incidence, particularly for rare traits, in the offspring of consanguineous parents. Consanguinity refers to the existence of a relationship by a common ancestor and increases the chance that both parents carry a gene affected by an identical mutation that they inherited. Consanguinity between parents of a child with a suspected genetic disorder implies (but does not prove) autosomal recessive inheritance. Although consanguineous unions are uncommon in Western society, in other parts of the world (southern India, Japan, and the Middle East) they are common. The risk of a genetic disorder for the offspring of a first-cousin marriage (6-8%) is about double the risk in the general population (3-4%).
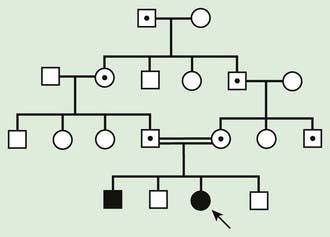
Figure 75-7 Autosomal recessive pedigree with parental consanguinity. Central dot, carriers; black, affected patients.
where p is the frequency of one of a pair of alleles and q is the frequency of the other. For example, if the frequency of cystic fibrosis among white Americans is 1 in 2,500 (p2), then the frequency of the heterozygote (2pq) can be calculated: If p2 = 1/2,500, then p = 1/50 and q = 49/50; 2pq = 2 × (1/50) × (49/50) = 98/2500 or 3.92%.
Pseudodominant Inheritance
Pseudodominant inheritance refers to the observation of apparent dominant (parent to child) transmission of a known autosomal recessive disorder (Fig. 75-8). This occurs when a homozygous affected individual has a partner who is a heterozygous carrier, and it is most likely to occur for relatively common traits, such as sickle cell anemia or nonsyndromic autosomal recessive hearing loss due to mutations in GJB2, the gene that encodes Connexin 26.