CHAPTER 56 Pancreatic Secretion
As has been reviewed in Chapter 55, the pancreas is an exocrine organ as well as an endocrine organ. This chapter is devoted to the exocrine pancreas. The exocrine pancreas has been of considerable interest to physiologists and other scientists for quite some time; in fact, the first demonstration of a hormone action was in the pancreas around the turn of the 20th century.1 The pancreas has been the major organ used to demonstrate the mechanisms of synthesis and transport for exportable proteins2 as well as the signaling pathways involved in regulated protein secretion.3 This chapter presents a concise description of the current understanding of pancreatic physiology.
FUNCTIONAL ANATOMY
The functional unit of the exocrine pancreas is composed of an acinus and its draining ductule (Fig. 56-1).4 The ductal epithelium extends to the lumen of the acinus with the centroacinar cell situated between the ductal epithelium and the acinus. The function of centroacinar is not established but may play a role in providing progenitor cells for pancreatic cell lineages. The ductule drains into interlobular (intercalated) ducts, which in turn drain into the main pancreatic ductal system.
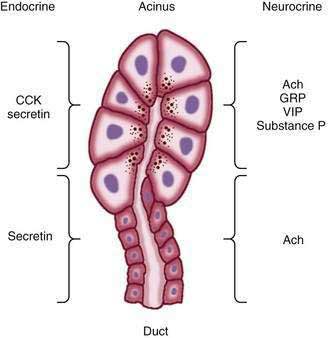
Figure 56-1. Regulation of exocrine pancreatic secretion during the intestinal phase of digestion. Exocrine secretions are regulated by endocrine and neurocrine pathways. In addition, the endocrine and neurocrine mediators regulate secretion from the acinus and the duct differ. For the acinus, cholecystokinin (CCK), secretin, acetylcholine (Ach), gastrin-releasing peptide (GRP), vasoactive intestinal polypeptide (VIP), and substance P regulate secretion. (Signaling mechanisms [second messengers] are shown in Fig. 56-6.) Secretin and Ach are the major regulators of pancreatic bicarbonate secretion from the duct. (Transporters involved in ductal secretion are illustrated in Fig. 56-4.)
(Adapted from Gorelick F, Pandol SJ, Topazian M. Pancreatic physiology, pathophysiology, acute and chronic pancreatitis. Gastrointestinal Teaching Project, American Gastroenterological Association, 2003.)
The acinus (from the Latin term meaning “berry in a cluster”) can be spherical or tubular, as shown in Figure 56-1, or can have some other irregular form.4 The acinar cells are specialized to synthesize, store, and secrete digestive enzymes. On the basolateral membrane are receptors for hormones and neurotransmitters that stimulate secretion of the enzymes.3 The basal aspect of the cell contains the nucleus as well as abundant rough endoplasmic reticulum for protein synthesis (Fig. 56-2, left). The apical region of the cell contains zymogen granules, the store of digestive enzymes. The apical surface of the acinar cell also possesses microvilli. Within the microvilli and in the cytoplasm underlying the apical plasma membrane is a filamentous actin meshwork that is involved in exocytosis of the contents of the zymogen granules.5,6 Secretion is into the lumen of the acinus. Tight junctions between acinar cells form a band around the apical aspects of the cells and act as a barrier to prevent passage of large molecules such as the digestive enzymes.7 The junctional complexes also provide for the paracellular passage of water and ions.
Another intercellular connection between acinar cells is the gap junction. This specialized area of the plasma membrane between adjacent cells acts as a pore to allow small molecules (molecular weight 500 to 1000 Da) to pass between cells. The gap junction allows chemical and electrical communication between cells.3 For example, calcium signaling is coordinated between the cells of an acinus with effects on digestive enzyme secretion.8,9
The duct epithelium consists of cells that are cuboidal to pyramidal and contain the abundant mitochondria necessary for energy products needed for ion transport (see Fig. 56-2, right). The duct cells as well as the centroacinar cells contain carbonic anhydrase, which is important for their ability to secrete bicarbonate.10
COMPOSITION OF EXOCRINE SECRETIONS
INORGANIC CONSTITUENTS
The principal inorganic components of exocrine pancreatic secretions are water, sodium, potassium, chloride, and bicarbonate (Fig. 56-3). The purposes of the water and ion secretions are to deliver digestive enzymes to the intestinal lumen and to help neutralize gastric acid emptied into the duodenum. Pancreatic juice secreted during stimulation with secretin is clear, colorless, alkaline, and isotonic with plasma. The flow rate increases from an average rate of 0.2 or 0.3 mL/minute in the resting (interdigestive) state to 4.0 mL/minute during postprandial stimulation. The total daily volume of secretion is 2.5 L. The osmolality of pancreatic juice is independent of flow rate. However, when the pancreas is stimulated by secretin (the major mediator of the greater volume output), bicarbonate and chloride concentrations change reciprocally (see Fig. 56-3).
Secretin stimulates secretion by binding to its receptor on the acinar cell and activating adenylate cyclase and increasing cyclic adenosine monophosphate (cAMP) in the ductal cell; acetylcholine does so by binding to its receptor and raising intracellular calcium concentrations. The initial events involve cAMP-dependent and Ca2+-dependent chloride (Cl−) channel activation on the luminal membrane as well as K+ channel activation on the basolateral membrane (Fig. 56-4).10–12 The cAMP-dependent Cl− channel is the cystic fibrosis transmembrane conductance regulator (CFTR).10–13 The activation of both channels by cAMP leads to Cl− secretion into the lumen. The higher chloride concentration in the lumen is coupled to a Cl−-HCO3− antiport, resulting in an exchange of Cl− for HCO3− in the lumen. Newer evidence also suggests a HCO3− channel on the apical surface involved in HCO3− secretion and that this HCO3− conductance is likely CFTR.11,12 On the basolateral surface of the duct cell are a Na+,H+ antiport and a Na+,HCO3− cotransport, as well as ATPases (Na+,K+-ATPase, H+-ATPase) and K+ channels. In combination, these transporters facilitate HCO3− secretion at the apical surface as well as maintain intracellular pH.14 Na+ and water are secreted into the ductal system to counter the electrical and osmotic forces resulting from the HCO3− secretion.
ORGANIC CONSTITUENTS15–17
The human pancreas has a large capacity for synthesizing protein (mostly digestive enzymes). Table 56-1 lists the major proteolytic, amylolytic, lipolytic, and nuclease digestive enzymes. Some of the enzymes are present in more than one form (e.g., cationic trypsinogen, anionic trypsinogen, and mesotrypsinogen). Enzymes that could digest the pancreas are stored in the pancreas and secreted into the pancreatic duct as inactive precursor forms. As illustrated in Figure 56-5, activation of these enzymes takes place in the intestinal lumen, where a brush-border glycoprotein peptidase, enterokinase, activates trypsinogen by removing (by hydrolysis) an N-terminal hexapeptide fragment of the molecule (Val-Asp-Asp-Asp-Asp-Lys).17 The active form, trypsin, then catalyzes the activation of the other inactive proenzymes.
Proenzymes* | Cationic trypsinogen |
Anionic trypsinogen | |
Mesotrypsinogen | |
Chymotrypsinogen (A, B) | |
Kallireinogen | |
Procarboxypeptidase A (1, 2) | |
Procarboxypeptidase B (1, 2) | |
Prophospholipase | |
Proelastase | |
Enzymes | Amylase |
Carboxylesterase | |
Sterol esterase | |
Lipase | |
DNase | |
RNase |
* Proenzymes listed are stored in the pancreas and secreted into the duodenal lumen as inactive proenzyme forms. If these enzymes were active in the pancreas, they would digest the pancreatic gland. Other enzymes, such as amylase and lipase, are stored and secreted in their active forms.
Adapted from Gorelick F, Pandol SJ, Topazian M. Pancreatic physiology, pathophysiology, acute and chronic pancreatitis. Gastrointestinal Teaching Project, American Gastroenterological Association, 2003.
In addition to the digestive enzymes, the acinar cell secretes a trypsin inhibitor, pancreatic secretory trypsin inhibitor (PSTI). This 56–amino acid peptide inactivates trypsin by forming a relatively stable complex with the enzyme near its catalytic site.18 The function of the inhibitor is to inactivate trypsins that are formed autocatalytically in the pancreas or pancreatic juice, thus preventing disorders such as pancreatitis.19
FUNCTIONS OF THE MAJOR DIGESTIVE ENZYMES
AMYLASE
Human amylase is secreted by both the pancreas and salivary glands. These enzymes digest starch and glycogen in the diet. Human salivary and pancreatic amylases have identical enzyme activities. However, they differ in molecular weight, carbohydrate content, and electrophoretic mobility.20 Salivary amylase initiates digestion in the mouth and may account for a significant portion of starch and glycogen digestion because it is transported with the meal into the stomach and small intestine, where it continues to have activity. In the stomach, the amylase activity is protected from secreted gastric acid by buffering from the meal and by the protected alkaline environment of salivary and gastric mucus. The action of both salivary and pancreatic amylase is to hydrolyze 1,4-glycoside linkages at every other junction between carbon 1 and oxygen. The products of amylase digestion are maltose and maltotriose (two- and three-α-1,4-linked molecules, respectively) and α-dextrins containing 1,6-glycosidic linkages, because 1,6-glycosidic linkages in starch cannot be hydrolyzed by amylase. The brush-border enzymes complete hydrolysis of the products of amylase digestion to glucose. The final product, glucose, is transported across the intestinal absorptive epithelial cell by a Na+-coupled transport (see Chapter 100).21,22
LIPASES
The pancreas secretes three lipases: lipase (or triglyceride lipase), phospholipase A2, and carboxylesterase (see Table 56-1). The most important source of these lipases, in contrast to amylase, is the pancreas. Salivary (lingual) and gastric lipases also contribute to fat digestion but in a minor fashion (see Chapter 49).
Pancreatic lipase hydrolyzes a triglyceride molecule to two fatty acid molecules released from carbons 1 and 3 and a monoglyceride with a fatty acid esterified to glycerol at carbon 2.16,23 Lipase binds to the oil-water interface of the triglyceride oil droplet, where it acts to hydrolyze the triglyceride. Bile acids as well as colipase are important for the full activity of lipase. Bile acids aid in the emulsification of triglyceride to enlarge the surface area for lipase to act on, and they form micelles with fatty acids and monoglyceride, which in turn remove these products from the oil-water interface. Colipase is believed to form a complex with lipase and bile salts. This ternary complex anchors lipase and allows it to act in a more hydrophilic environment on the hydrophobic surface of the oil droplet.
Phospholipase A2 catalyzes the hydrolysis of the fatty acid ester linkage at carbon 2 of phosphatidylcholine.16 This cleavage leads to the formation of free fatty acid and lysophosphatidylcholine.
Carboxylesterase has a broad specificity and will cleave cholesterol esters, lipid-soluble vitamin esters, triglycerides, diglycerides, and monoglycerides. Bile salts are also important for the full activity of this enzyme.24
PROTEASES
The combined actions of the pancreatic proteases and pepsin from the stomach result in the formation of oligopeptides and free amino acids. The oligopeptides are further digested by brush-border enzymes (see Chapter 100). Free amino acids and oligopeptides are transported across the intestinal mucosa by a group of Na+– and H+-coupled transporters.25 It is interesting that only certain amino acids (mostly essential amino acids) can be measured in the lumen during digestion, indicating that the combined action of the proteases is not random and that the products result from the combined specificities of the individual proteases. These amino acids have greater effects on stimulating pancreatic secretion, inhibiting gastric emptying, regulating small bowel motility, and causing satiety. Thus, the specific pattern of protease actions leads to physiologic regulation of several organs in the gastrointestinal tract.
DIGESTIVE ENZYME SYNTHESIS AND TRANSPORT
Synthesis of digestive enzymes takes place in the internal space of the rough endoplasmic reticulum (RER) (see Fig. 56-2, left).2,26 The mechanism for translation of the cell’s messenger RNA (mRNA) into exportable protein is explained by the signal hypothesis. The main feature of the hypothesis is that ribosomal subunits attach to mRNA and initiate synthesis of a hydrophobic “signal” sequence on the NH2-terminal of nascent proteins. This complex then attaches to the outer surface of the endoplasmic reticulum, and the signal sequence targets the protein being synthesized into the lumen of the RER.
Newly synthesized proteins can undergo modifications in the endoplasmic reticulum, including disulfide bridge formation, phosphorylation, sulfation, and glycosylation. Conformational changes resulting in tertiary and quaternary structures of the protein also take place in the endoplasmic reticulum. Processed proteins in the RER are transported to the Golgi complex where further post-translational modification (glycosylation) and concentration occur.27 Important conformational specificities are required for appropriate function and transport of proteins between compartments. These conformational specificities are facilitated by proteins called chaperones and foldases among others. When there is an increased demand on the RER for protein synthesis or when there is a cell stress that causes unfolding of proteins, the RER responds by increasing synthesis of chaperones and foldases, ceases synthesis of the proteins, and even degrades unfolded proteins using the mechanisms of the unfolded protein response (UPR).28 By keeping proteins in their appropriate conformation, the UPR maintains normal transport of proteins between compartments of the cell.
The Golgi complex also serves the important function of sorting and targeting newly synthesized proteins into various cell compartments. Digestive enzymes are transported to the zymogen granules.27 Lysosomal hydrolases are sorted to the lysosome.29 For this lysosomal pathway, mannose-6-phosphate groups are added to oligosaccharide chains on the protein during its presence in the cis-Golgi complex. The mannose-6-phosphate groups serve as a recognition site for a specific receptor. The interaction of the lysosomal enzyme mannose 6-phosphate with its receptor leads to formation of vesicles that transport this complex to the lysosome, delivering the enzyme. In the lysosome the enzyme dissociates from the receptor, which in turn cycles back to the Golgi complex.
Secretion of the digestive enzymes occurs by exocytosis. Exocytosis consists of movement of the secretory granule to the apical surface, the recognition of a plasma membrane site for fusion, and the fission of the granule membrane-plasma membrane site after fusion.2,30 Recent studies demonstrate roles for actin-myosin, SNARE (soluble N-ethylmaleimide–sensitive factor attachment protein [SNAP] receptor) proteins, and guanosine triphosphate (GTP)-binding proteins in these processes.30–38 Intracellular signals generated by agonist receptors as discussed following interact with these entities to mediate digestive enzyme secretion via exocytosis of zymogen granules.