Chapter 31 Pacing Technology and Its Indications
Advances in Threshold Management, Automatic Mode Switching, and Sensors
Since the first endocardial pacing lead implantation in 1958, pacemaker therapy has undergone remarkable technologic advances. For example, the number of circuitry components has increased from a mere two to three transistors in early pacemakers to nearly one million components with RAM (random access memory) sizes up to 124,000 bytes.1 This increased sophistication has led to pacemaker features that the average pacemaker implanter may not have the time to understand or to program appropriately. In addition, threshold and sensor assessment may take up to 40% of time in an average follow-up (Figure 31-1).2 Thus, automatic optimization of many pacing parameters has become a pressing need. This chapter reviews the current state of the art in three important modern pacemaker functions: (1) capture management, (2) automatic mode switching (AMS), and (3) implantable sensors. Particular attention is given to their indications and automaticity in programming.
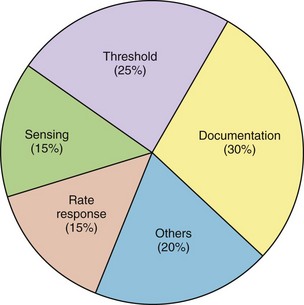
FIGURE 31-1 Time used for different activities during a routine pacemaker follow-up.
(From Marshall M, Butts L, Flaim G, et al: Predictors of time requirements for pacemaker clinic evaluation [abstract], Pacing Clin Electrophysiol 18:952, 1995.)
Capture Management
The primary function of a pacemaker is to pace effectively at an efficient energy output. This depends on the pacing threshold, which varies significantly from individual to individual and within an individual over time. The latter may occur because of the spontaneous threshold rise after implantation, the occurrence of gross dislodgment or microdislodgment, diurnal changes, and the changes introduced by drugs and myocardial ischemia.3,4 Thus, the ability to track threshold automatically will maximize patient safety, minimize battery drain for pacing, and, importantly, simplify programming. Box 31-1 presents a list of reasons for the need for automatic capture management.
Box 31-1 Needs and Potential Benefits of Capture Management
St. Jude/Pacesetter Autocapture
The Autocapture pacing system was first introduced in the single-chamber Microny pacemaker from St. Jude Medical in 1995. (See Box 31-2 for information on the manufacturers referred to in this chapter.) It is designed to verify a response to each pacemaker stimulation that represents capture or myocardial depolarization and to automatically adjust the pacing output accordingly on a beat-to-beat basis. After a VP stimulus, the Autocapture algorithm opens an evoked response (ER) detection window for 46 ms after a 15-ms blanking period. Detection of an ER is used to diagnose capture. If an ER is not detected (loss of capture), a high-energy backup pulse of 4.5 V is discharged at 100 ms after the VP stimulus. If two consecutive backup pulses are delivered, the algorithm starts a stimulation threshold search by increasing the output to effect two consecutive captures (Figure 31-2). In single-chamber devices (Microny and Regency SR), a margin of 0.3 V is added. In addition, to avoid pacing at high output caused by diurnal fluctuation in threshold, the device automatically performs a threshold search once every 8 hours. Again a safety margin of 0.3 V is added to the detected threshold. In dual-chamber devices, the A-V interval is shortened to 50 ms (Ap) or 25 ms (atrial sensing [As]) to ensure overdrive of intrinsic ventricular rhythm. In the Affinity DR, automatic decrements and increments of output during threshold search are 0.25 V and 0.125 V, respectively (see Figure 31-2). In addition, beat-to-beat capture verification has only recently been extended to atrial stimulation in the Zephyr pacemaker by St. Jude Medical (ACP confirm), as the small atrial electrical signal represents a major challenge in discrimination between the ER signal and the pace-induced after-polarization (see below).
Box 31-2 Pacemaker Manufacturers and Models
Efficacy
Factors that affect the Autocapture’s detection of an ER are listed in Box 31-3. One major challenge to this approach is the difficulty in distinguishing the ER signal and the pace-induced after-potential. A large electrode polarization artifact relative to the size of the ER can affect ER detection. This can be reduced with the use of low-polarization electrodes (made possible by increasing the microscopic electrode-tissue interface area).5 An alternative is to use a biphasic waveform that comprises a fast precharge followed by a negative postcharge to minimize the polarization effect.6 In one study, the effect of a modified fast prepulse on the Autocapture was tested in 45 patients with leads from two manufacturers (Medtronic 4024 Cap Sure, and Pacesetter 1450 K/T and 1470 T leads).7 Whereas the ER was independent of the type of pacing pulse, the polarization artifact was significantly less during the modified pulse compared with the conventional pacing pulse, resulting in an improved efficacy of the Autocapture algorithm (94% vs. 71% success rate in ER detection). An adequate ER amplitude of greater than 2.5 mV is recommended before activation of the Autocapture algorithm; this was present in 93% of 60 patients in one study.8 Neither the clinical data nor the conventional electrical parameters were effective in predicting the size of the ER signal. Body posture and exercise had relatively little effect on the ER.9 Recently, a new ER algorithm measuring the depolarization integral (area) instead of ER signal amplitudes (voltage) to determine ER has enhanced the accuracy of capture verification; in fact, the algorithm allows ER determination even with old high-polarization bipolar leads. Because of enhanced sensitivity, it has become possible to distinguish a small atrial ER from a pace-induced after-potential.
In a multicenter study, 113 patients received the Pacesetter Microny SR+ and were followed up for 1 year. ER was satisfactory for Autocapture in 102 of 113 patients.10 Even though ER was stable over time it correlated poorly with the R wave at the time of implantation. Acute and chronic pacing thresholds measured at the clinic using a standard surface ECG VARIO threshold test significantly correlated with that derived from the Autocapture, although the Autocapture threshold was higher (0.11 ± 0.22 V) because of the way in which threshold was derived. During Holter recordings, no failure of ventricular capture occurred, and backup pulses were used in 1.1% of all paced beats. Most of these were caused by fusion or pseudo-fusion beats (87%), undersensing of either the R wave or the ER (4.6%), and was the true cause of loss of capture in only 7%. Although these did not affect pacing performance, the need for backup pulses may negate the energy saving by the Autocapture itself. Similar positive results from the Autocapture algorithm in the medium term for safety and efficacy have been published.11,12
Projected increases in battery longevity afforded by the Autocapture have been reported.13,14 Compared with the factory-set pacemaker setting of 5 V, the Autocapture reduced the energy drain in the Microny SR+ (with 0.35 Ah) and increased device longevity by 53%. For the Regency SR+ with a larger battery (0.79 Ah), the increase in device longevity was even more significant (245%). However, when the conventional output was reduced to 2.5 V, the benefit of the Autocapture on battery life was much less impressive.13,14
Clinical Implications
The main benefits of any automatic capture management algorithm are patient safety and effective capture during threshold changes. Programming of threshold can be simplified as the Autocapture threshold has been significantly correlated with bedside threshold assessment. The energy saving would be more important in patients with chronic high thresholds. Conversely, fusion and pseudo-fusion beats appear to be the main limitation, not only because they reduce battery energy but also because they may lead to erroneous threshold determination.15
Biotronik Capture Control
The Logos pacemakers measure the ER signals from several successful capture beats to generate a reference curve, against which failure of capture is compared.16,17 No backup pacing pulses are present, but persistent loss of capture results in the increase of pulse output in 2-V steps. After a programmable period, the output is reduced to the programmed value. This algorithm ensures patient safety through beat-by-beat capture verification.
Medtronic Ventricular Capture Management
The Kappa 700 pacemakers incorporate a threshold assessment based on ER: The Pacing Threshold Search (ambulatory) and Capture Management Threshold Test (bedside). During the procedure, the threshold at the Rheobase is determined at 1 ms by amplitude decrement until loss of capture and then by amplitude increment until capture is confirmed. The chronaxie is then determined by doubling the programmed amplitude and decreasing the pulse width (and subsequently increasing the amplitude to capture). A recommended pacing setting is then determined (Figure 31-3). The physician can use the ambulatory threshold data to automatically adjust threshold (adaptive), use the data only for monitoring, or turn off the algorithm. A minimal adapted output needs to be programmed. The ventricular capture management can be activated once every 15 minutes for 42 days and is not a beat-by-beat threshold tracking algorithm.
Automatic Mode Switching
Because the ventricular response of a DDD pacemaker is dependent on the atrial rate, rapid VP can occur in a DDD pacemaker during episodes of atrial tachycardias (AT), especially during AF (Figure 31-4). This is managed in contemporary pacemakers by using an algorithm known as automatic mode switching. Patients with dual chamber pacemakers will develop AT for several reasons: (1) Nearly one half of patients receiving pacemakers have sinus node disease, and a substantial proportion of these patients have bradycardia-tachycardia syndrome. (2) As many as 30% of patients with complete AV block either have coexistent bradycardia-tachycardia syndrome or will develop this problem with time.18 (3) A dual chamber pacemaker is often used in patients after AV nodal ablation for refractory AT. (4) The incidence of AF increases markedly with age.19
Several registries and controlled trials have generated data on the incidence of AF. From 1988 to 1990, 12.9% of Medicare pacemaker recipients who received dual-chamber pacemakers had underlying paroxysmal AF.20 After implantation of a dual-chamber pacemaker, patients have an overall 2% to 3% per year risk of developing AF. In patients with sinus node disease, the risk of developing paroxysmal or persistent AF is increased to 8% per year.21–24
Conventional or specially designed pacemakers can convert automatically to another pacing mode under a variety of circumstances.25–32 The term automatic mode switching is now used to define an automatic function whereby a device is designed to switch temporarily to a nonatrial tracking destination mode during an AT and to switch spontaneously back to the original mode on resumption of sinus rhythm.
Components of an Automatic Mode Switching Algorithm
Atrial Tachycardia Detection
A device can detect AT in four main ways (Table 31-1): (1) Most devices use a rate cutoff criterion, in which a sensed atrial rate exceeding a programmable value will result in AMS. Some systems are designed to avoid mode switch during atrial ectopic beats or short runs of AT. For example, four short cycles of seven consecutive beats are required before AMS occurs in the Medtronic Kappa 700. Interval number summation is used in the incremental and decremental counter of the Meta DDDR for short cycles and long cycles, respectively. (2) Some devices use a mean atrial rate, or matched atrial rate, based on a moving value related to the duration of the prevailing sensed atrial cycle as a criterion to move toward AMS. AMS will occur when the matched atrial interval has shortened to a predetermined duration. This algorithm is used in the Medtronic Thera DR, the Kappa 400 (GEM DR ICD), and the St. Jude Trilogy DR+/Affinity family. Because the process is gradual, the rapidity of AMS will depend not only on the AT detection rate or interval but also on the pre-existing sinus rate. It is easier for the matched atrial interval to reach the tachycardia detection interval when AT occurs in the setting of a higher resting sinus rate than from a sinus bradycardia. This is because the matching atrial interval starts from a shorter baseline duration on its gradual way to reach the tachycardia detection interval. (3) Sensors can be used to determine the physiological rate (e.g., Diamond and SmarTracking in Marathon). To take into account the fluctuation in sinus rate, a physiological heart rate range based on the sensor-indicated rate is used to define sinus rhythm, and rates beyond the upper end of the physiological range will activate AMS. (4) Complex algorithms are a combination of algorithms, or they use additional criteria (often from implantable cardioverter-defibrillators [ICDs]) to distinguish AF and other rhythms. For example, a PR logic and a rate criterion are instrumented in the AT500 (Medtronic, Inc.) to detect AF and AT.33
Table 31-1 Classification of Different Methods of AT Detection in Current AMS Algorithms
CRITERION | EXAMPLES | INDICATIONS FOR MODE SWITCHING |
---|---|---|
Rate cutoff | Pulsar/Vigor/Meridian/Discovery | Incremental/decremental counter |
Inos/Logos | Ratio of short/total cycles (e.g., 4 of 7 consecutive cycles) | |
Kappa 400/700 | Ratio of short/total cycles (e.g., 4 of 7 consecutive cycles) | |
Marathon DR | Consecutive short cycles | |
Meta DDDR (model 1254/1256) | Incremental/decremental counter | |
Running average rate | Thera DR | Matched atrial interval computed from prevailing atrial rate |
Trilogy DR Affinity | Filtered atrial interval | |
Sensor-based physiological rate | Clarity/Diamond | Single beat outside a physiological rate band (15 or 30 beats/min) |
Marathon DR | SmarTracking rate range (accelerometer sensor) | |
Meta DR (model 1250) | Sensor-controlled PVARP | |
Living 1/Living 1 Plus | Sensor-indicated rate to define tachycardia detection | |
Complex | Marathon DR | SmarTracking and rate cutoff |
AT 500 | Rate cutoff and PR relationship |
AT, Atrial tachycardia; AMS, automatic mode switching; PVARP, postventricular atrial refractory period.
The Ideal Automatic Mode Switching Algorithm
An ideal AMS algorithm (Table 31-2) should have an appropriate onset speed after AT begins. Prolonged rapid VP caused by a slow algorithm or oscillation between mode switching and tracking during short-lasting AT in a fast algorithm will result in undesirable ventricular rate fluctuation, AV dissociation, or both. It is clear that speed of response and rate stability are two competing parameters. Atrial and ventricular responses during AMS should result in a pacing rate appropriate to the pathophysiological state of the patient. In general, this rate is sensor determined. At the termination of AT, and to avoid AV dissociation during the process, the algorithm should resynchronize to sinus rhythm at the earliest opportunity. Many algorithms incorporate a rate fallback mechanism to ensure smooth rate control during mode transitions. With ideal sensing and programming, these characteristics are dependent entirely on the AMS algorithm.
CHARACTERISTICS | REMARKS |
---|---|
Onset | Is rapid and avoids high-rate ventricular pacing without causing frequent mode oscillations during unsustained AT |
Response | Avoids excessive rate fluctuation |
Avoids inappropriate atrial pacing | |
Resynchronization | Restores AV synchrony to sinus rhythm at the earliest opportunity |
Sensitivity | Has the ability to sense AT of varying rates and signal sizes |
Has the ability to sense atrial flutter | |
Specificity | Avoids switching during VA cross-talk, sinus tachycardia, and extraneous electrical noises |
AMS, Automatic mode switching; AV, atrioventricular; AT, atrial tachycardia; VA, ventriculoatrial.
In clinical practice, however, arrhythmia-related and sensing-related issues affect the sensitivity and specificity of the AMS response significantly. Sensitivity of an AMS algorithm refers to its ability to detect AT (i.e., avoid false-negative response), whereas specificity refers to the absence of AMS during sinus rhythm (i.e., avoid false-positive response) (Table 31-3). With AMS algorithms, the greater the sensitivity, the lower is the specificity, and vice versa.
Table 31-3 Factors Affecting AT Detection in Dual-Chamber Pacemakers with AMS Algorithms
UNDERDETECTION (SENSITIVITY) | OVERDETECTION (SPECIFICITY) | |
---|---|---|
Arrhythmia related | Atrial flutter | Sinus tachycardia |
AF with small or widely varying signal amplitudes | — | |
Slow atrial tachycardia (actual or drug-induced) | — | |
Pacemaker related | ||
Lead configurations | — | Unipolar sensing (far-field sensing and myopotentials) |
Low atrial lead positions | ||
Lead in the coronary sinus | ||
Dual-site atrial or bi-atrial sensing | ||
Atrial sensitivity | Insufficient atrial sensitivity to sense AF | |
Atrial blanking | Reduced sensed AT rate | Inadequate blanking in A–V interval and after ventricular pacing |
A–V interval | Reduced sensed AT rate (some devices) | — |
PVARP | Reduced sensed AT rate (some devices) | PVARP-mediated AMS: AMS can occur during sinus tachycardia or ectopy |
VA cross-talk | — | Increased sensed atrial rate |
AF, Atrial fibrillation; AMS, automatic mode switching; AT, atrial tachycardia; AV, atrioventricular; PVARP, postventricular atrial refractory period.
Automatic Mode Switching Sensitivity
As most AMS algorithms detect AT by a rate cutoff criterion, a slow atrial rate (e.g., atrial rate slowing after antiarrhythmic medications) may fall below the tachycardia detection rate, and AMS will not occur. Conversion of AF to atrial flutter is a special situation in which alternate flutter waves coincide with the postvertricular atrial blanking period (PVAB), and the effectively detected atrial rate falls below the tachycardia detection rate and prevents AMS (Figure 31-5).
In the currently available devices, the postventricular atrial refractory period (PVARP) is opened (completely or on a conditional high rate) to enhance AMS. In other words, sensing occurs in the second part of the PVARP beyond the PVAB. The latter is designed to prevent far-field R-wave sensing. Atrial undersensing may occur because of an inappropriately long PVAB. Undersensing can often be avoided by appropriate adjustment of the PVAB, provided the atrial channel exhibits no far-field sensing. The widely varying amplitude of atrial ECG in AF, both temporally in a patient and between patients, can result in AMS failure when the atrial sensitivity is programmed incorrectly. During electrophysiological study, acutely recorded atrial ECGs (ECGs) during AF and sinus rhythm show similar mean amplitude, but the variability in amplitude is substantially wider and the minimum amplitude considerably smaller in AF compared with sinus rhythm (minimum atrial ECG: 1.4 ± 1.1 and 2.0 ± 0.8 mV, respectively).34 A high-programmed atrial sensitivity may cause As of far-field signals or noise, whereas a low atrial sensitivity can lead to undersensing during AF (Figure 31-6).35 Optimal programming of atrial sensitivity for AMS requires three times the safety margin compared with two times for sinus P-wave sensing (Figure 31-7).35