Overview of the nervous system
Parts of the nervous system
Central and peripheral nervous systems
The nervous system is divided into central and peripheral parts (Fig. 1.1). The central nervous system (CNS) is made up of the brain and spinal cord, encased within the bones of the skull and vertebral column. The CNS represents the main integrating and decision-making centre of the nervous system. The peripheral nervous system (PNS) includes 31 pairs of spinal nerves which emerge between the vertebrae (Fig. 1.2) and 12 pairs of cranial nerves which arise from the base of the brain (Fig. 1.3). At the roots of the upper and lower limbs, sensory and motor fibres are redistributed in the brachial and lumbosacral plexuses to enter a number of named peripheral nerves (Figs 1.4 and 1.5). The function of the PNS is to transmit nerve impulses to and from the brain and spinal cord.
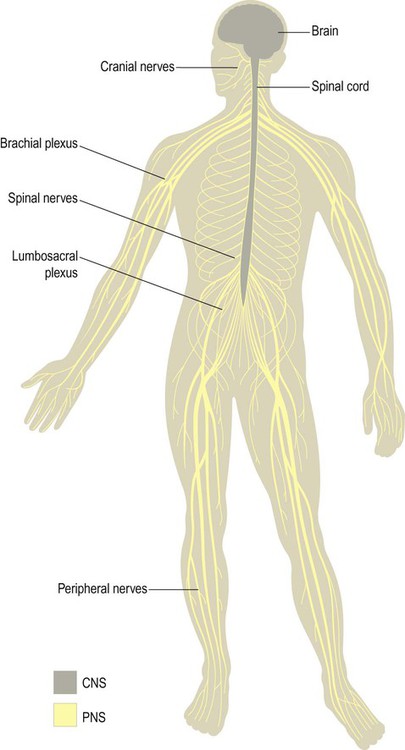
The central nervous system (CNS) includes the brain and spinal cord. The peripheral nervous system (PNS) is made up of sensory and motor fibres passing to and from the CNS.
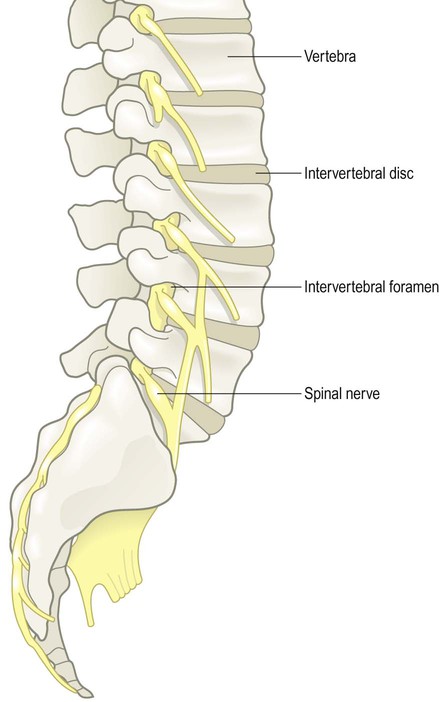
The mixed spinal nerves can be seen emerging from the intervertebral foramina.
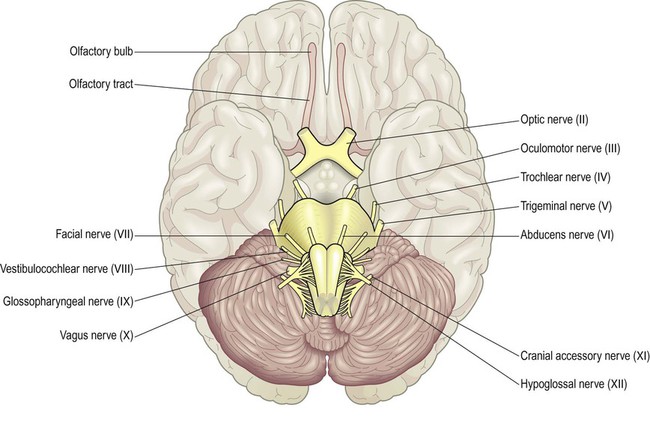
The olfactory nerve (cranial nerve I) is not seen here; it consists of millions of filaments that arise from the nasal cavity and synapse in the olfactory bulb.
Autonomic nervous system
The autonomic nervous system (ANS) or visceromotor system regulates the activities of the cardiovascular, respiratory, digestive and urogenital systems. It operates autonomously (Greek: autos, self; nomos, governed by law). The ANS depends upon a constant stream of sensory information from the internal organs and blood vessels which it uses to regulate the contraction of cardiac and smooth muscle and the secretory activity of glands. Ultimate control of the autonomic nervous system resides in the hypothalamus, a tiny region at the centre of the brain that is sometimes referred to as the ‘head ganglion’ of the ANS (described later; see also Ch. 3).
Divisions of the ANS
The autonomic nervous system has two divisions that widely innervate the body and tend to have opposing effects on their target structures (Fig. 1.6):
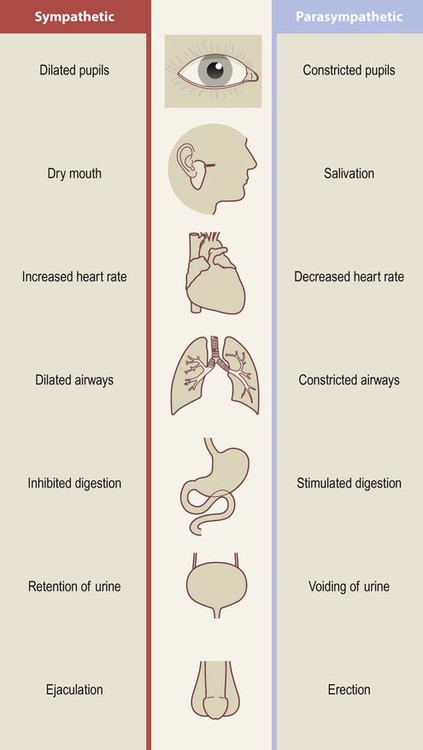
The two limbs of the ANS tend to have opposing or complimentary effects on their target structures.
The sympathetic nervous system is most active during highly stressful situations that provoke intense fear or rage, typified by the ‘fight or flight’ response: e.g. dilated pupils, dry mouth, racing pulse. It is said to act ‘in sympathy’ with the emotions.
The parasympathetic nervous system generally has an antagonistic or complimentary effect on a given organ or tissue and is more associated with vegetative activities (‘resting and digesting’).
It is important to note that most structures are innervated by both divisions of the ANS and can be controlled independently (e.g. it is possible to dilate the pupils without increasing the heart rate); but the ‘fight or flight’ response is a useful way to remember the effects of the sympathetic division.
The two divisions of the ANS have distinct anatomical origins. Sympathetic fibres originate in the thoracic and upper lumbar segments of the spinal cord (T1–L2/3) as the thoracolumbar outflow (Fig. 1.7). This feeds into the sympathetic chain, on either side of the vertebral column, which provides sympathetic innervation to the entire body.
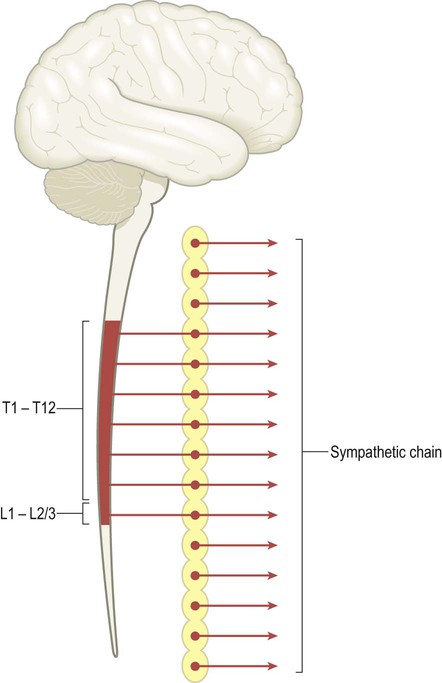
Sympathetic fibres from the thoracic and upper lumbar spinal cord (T1–L2/3) innervate the sympathetic chain, which is located on either side of the vertebral column. Superior and inferior extensions of the sympathetic chain provide sympathetic innervation to the entire body.
The parasympathetic fibres originate in cranial nerves III, VII, IX and X and the sacral spinal cord (S2–4). This constitutes the craniosacral outflow (Fig. 1.8). The vagus nerve (cranial nerve X) has a wide territory of distribution that includes most of the thoracic and abdominal viscera (Latin: vagus, wandering).
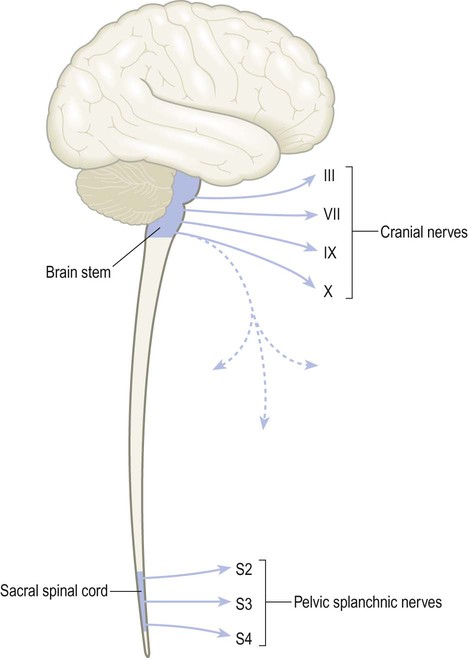
The craniosacral outflow includes contributions from cranial nerves III, VII, IX and X and the pelvic splanchnic nerves which arise from segments S2–S4 of the sacral spinal cord. Note that the vagus nerve arises in the brain stem but descends in the neck (dashed blue line) to supply most of the thoracic and abdominal organs.
Cells of the nervous system
Neural tissue contains two specialized cell types: neurons and glia. These will be introduced here and discussed further in Chapter 5.
Nerve cells (neurons)
The basic structural and functional unit of the nervous system is the neuron or nerve cell. Neurons are electrically excitable cells that are highly specialized for the receipt, integration and transmission of information via rapid electrochemical impulses. A typical neuron is illustrated in Fig. 1.9.
The point of contact between two neurons is called a synapse (Greek: sunapsis, point of contact). Neurons influence effector structures such as muscle fibres and glandular tissue at neuroeffector junctions. The specific junction between a somatic motor neuron and a skeletal muscle fibre is the neuromuscular junction (NMJ) (see Ch. 4). The electrical activity of the target cell is influenced by neurotransmitters, chemical mediators that are released at the axon terminal in response to the arrival of a nerve impulse. Neurotransmitters are stored in membrane-bound organelles within the axon terminal called synaptic vesicles (see Ch. 7).
Neurons can be classified into three functional types (Fig. 1.10):
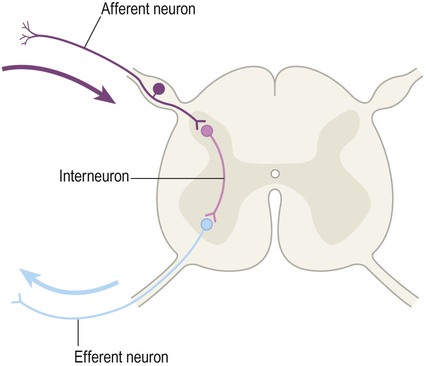
The vast majority of nerve cells in the central nervous system are interneurons (association neurons).
Afferent neurons carry nerve impulses towards the central nervous system. The term afferent fibre (or simply ‘afferent’) is also used to refer to any nerve process carrying impulses towards a particular structure. For example, ‘cortical afferents’ are fibres carrying impulses to the cerebral cortex. The term sensory neuron is usually reserved for afferent neurons conveying information that is to be consciously perceived.
Efferent neurons carry nerve impulses away from the central nervous system. Again, the term efferent fibre (or ‘efferent’) can be used to refer to any process carrying impulses away from a particular structure. The term motor neuron is best reserved for efferent neurons that are involved in pathways concerned with voluntary movement.
Association neurons (or interneurons) make up the vast network of interconnections within the brain and spinal cord. They have an integrative function, transforming sensory inputs into appropriate motor responses. The majority of neurons therefore fall into this category.
Neurons can also be classified by the number of processes that they have. The example shown in Fig. 1.9 is a multipolar neuron because it has several processes. Neurons with one or two processes are termed unipolar and bipolar respectively. The vast majority of neurons in the CNS are multipolar.
Grey and white matter
CNS tissue can be divided into grey and white matter (Fig. 1.11). Grey matter is composed mainly of neuronal cell bodies, dendrites and synapses. It is sharply demarcated from the adjacent white matter, which is made up of nerve fibres travelling to other parts of the nervous system. The pale colour of white matter is due to the lipid-rich myelin sheath that surrounds axons and enhances their conduction velocity (see Fig. 1.9; see also Chs 5 & 6). Discrete groups of neurons within the central nervous system are referred to as nuclei (singular: nucleus). In the peripheral nervous system, collections of neuronal cell bodies form aggregates that resemble knots on a piece of string. These are referred to as ganglia (singular: ganglion).
Neuroglial cells
In addition to neurons, the nervous system contains a variety of support cells that are known collectively as glia. These cells were originally thought to offer mainly physical support, literally holding nervous tissue together (Greek: glia, glue) but they are now known to carry out a wide range of important functions. The four main types of glial cell (discussed further in Chapter 5) are:
Astrocytes, which provide structural and metabolic support to neurons and help to regulate the exchange of molecules between the bloodstream and the brain.
Oligodendrocytes, which invest axons with a myelin sheath in the brain and spinal cord.
Schwann cells, the peripheral counterparts of oligodendrocytes.
Microglia, the resident phagocytic and immunocompetent cells of the central nervous system.
Although glial cells are relatively small, with an average diameter of around 4–8 µm, they outnumber neurons in a ratio of 10 : 1 and make up approximately 50% of the total volume of the CNS. Glial cells are able to undergo cell division (unlike neurons, which are post-mitotic cells).
Basic cerebral topography
The human brain is a pale pink organ with a mean mass of around 1.3 kg and a very soft, gelatinous consistency. It can be separated into three major parts – the cerebrum, cerebellum and brain stem (Fig. 1.12). The brain stem is further subdivided into the midbrain, pons and medulla oblongata.
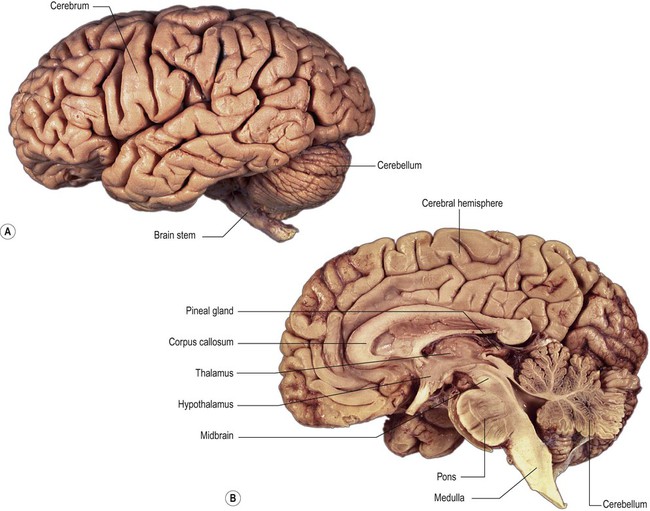
(A) Lateral surface; (B) Median sagittal section. From Crossman: Neuroanatomy ICT 4e (Churchill Livingstone 2010) with permission.
Cerebrum
The cerebrum is dominated by the paired cerebral hemispheres which are responsible for cognition, language, memory, emotion and behaviour. The surface of the cerebral hemispheres is composed of a thin shell of grey matter, the cerebral cortex (Latin: cortex, bark) which is 2–4 mm thick. The cortex is thrown into numerous convolutions or gyri separated by furrows called sulci (singular: gyrus and sulcus) (Fig. 1.13). Cortical folding allows the maximum surface area of grey matter to be compressed into the limited confines of the skull, with two-thirds of the cortex hidden within the depths of sulci.
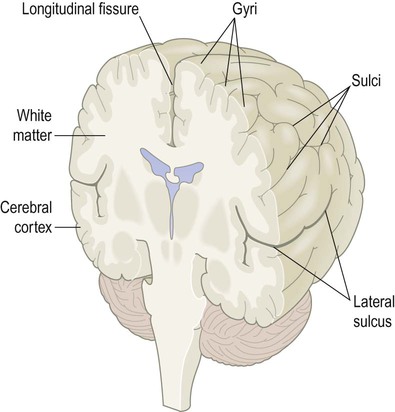
In the cerebral hemispheres, all furrows that are lined by cortical grey matter are referred to as sulci; compare the lateral sulcus (which is lined by a continuous ribbon of grey matter) to the longitudinal fissure (which is not).
The cerebral hemispheres are divided by a deep cleft termed the longitudinal fissure and separated from the underlying cerebellum by the transverse fissure. Two prominent sulci on the cerebral convexity, the lateral sulcus and the central sulcus, help to divide the hemispheres into frontal, parietal, occipital and temporal lobes (Fig. 1.14; see also Ch. 3).
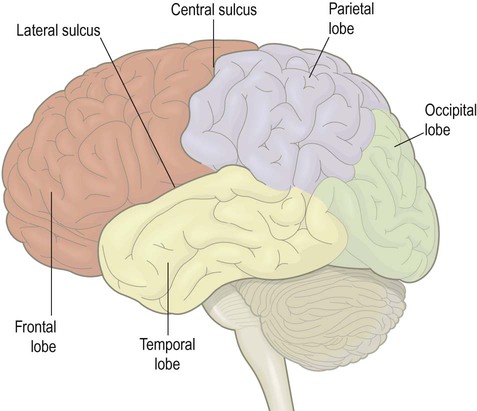
Note: there are no consistent sulci on the lateral surface of the hemisphere that clearly delineate the posterior lobar boundaries.
Internal anatomy
Slicing the cerebrum reveals a number of grey matter structures including the corpus striatum, amygdala and thalamus (discussed further below) (Fig. 1.15). These subcortical structures are closely related to a collection of fluid-filled cavities and channels inside the brain, called the ventricular system.
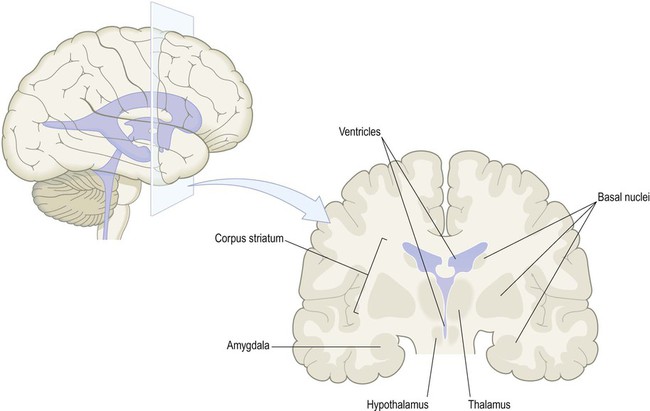
The corpus striatum and amygdala are referred to as basal nuclei (grey matter in the base of the cerebral hemisphere). The thalamus and hypothalamus are not basal nuclei, but are instead part of the diencephalon, a central part of the cerebrum surrounding the cavity of the third ventricle.
The corpus striatum is the largest component of the basal ganglia. These are a collection of nuclei (the term ‘ganglia’ is a misnomer) that are involved in movement control and are affected in Parkinson’s disease (Ch. 13). They also contribute to cognition, emotion and behaviour. The amygdala is concerned with emotional responses (especially anxiety and fear). It has close links to the limbic lobe, part of the brain that is particularly concerned with emotion and memory.
The thalamus and hypothalamus belong to the diencephalon. This region lies at the centre of the brain, surrounding the cavity of the third ventricle and is normally hidden from view between the cerebral hemispheres (Greek: dia-, between; enkephalos, brain) (Fig. 1.16). The thalamus is known as the ‘gateway’ to the cerebral cortex, since most ascending sensory pathways relay in one of its nuclei in order to reach their cortical targets. The hypothalamus controls the ANS and endocrine system and is involved in the maintenance of homeostasis.
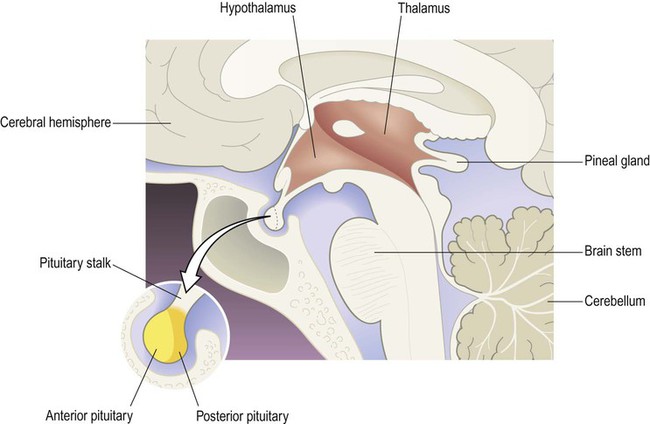
The main components of the diencephalon are the thalamus and hypothalamus which form the side wall and floor of the third ventricle (coloured red). Although not part of the diencephalon, the pituitary gland is attached to the floor of the third ventricle and has links with the hypothalamus.
Hemispheric white matter
The subcortical white matter is composed of numerous interlacing tracts, which are groups of axons with a common origin, destination and function. Two or more tracts running in company make up a bundle or fasciculus (plural: fasciculi). There are three main types of white matter bundle in the cerebrum, illustrated in Figs 1.17–1.19:
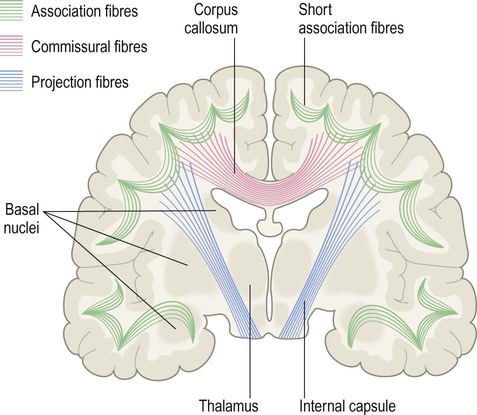
The corpus callosum is the main white matter connection between the cerebral hemispheres; the internal capsule is a massive white matter bundle consisting of fibres passing to and from the cerebral cortex.
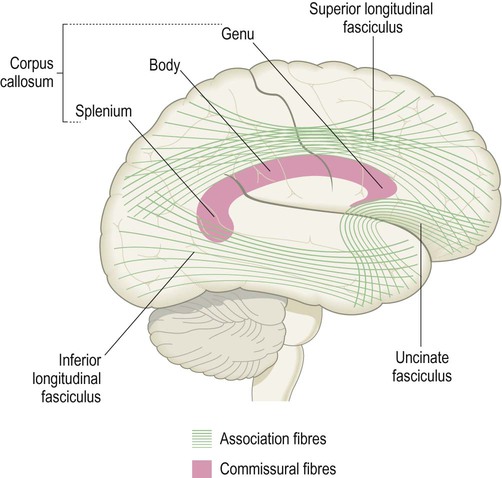
Some of the major long association fibres are shown projected on to the surface of the cerebral hemisphere. The approximate position of the corpus callosum appears in transverse section; notice the genu and splenium, which give rise to fibres interconnecting the frontal lobes and the posterior parts of the cerebral hemispheres respectively.
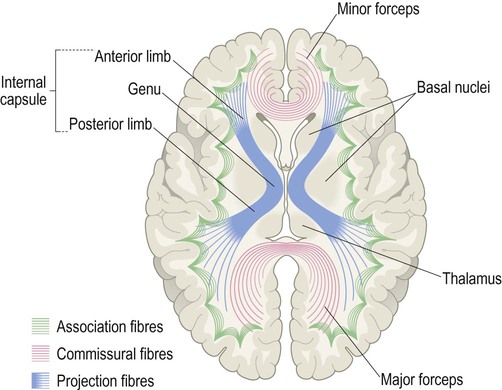
The internal capsule (blue) is seen in transverse section and consists of ascending and descending axons passing to and from the cerebral cortex [to understand the orientation of the fibres, compare with Fig. 1.17]. The minor and major forceps are also seen in this view, which represent commissural fibres crossing the midline in the genu and splenium of the corpus callosum [compare with Fig. 1.18].
Association fibres interconnect parts of the same hemisphere. Short association fibres (subcortical ‘U-fibres’) loop between neighbouring gyri. Long association fibres link more distant areas within a particular hemisphere.
Commissural fibres cross the midline to connect with matching areas of the opposite hemisphere (Latin: commissūra, a joining together). The largest commissure is the corpus callosum, composed of some 200–300 million myelinated axons. The anterior and medial temporal lobes are linked by the much smaller anterior commissure (see Ch. 3, Fig. 3.25).
Projection fibres pass to and from the cerebral cortex. The internal capsule is a massive white matter system composed of 20 million projection fibres that pass through the corpus striatum.
The internal capsule contains the pyramidal tract (primary voluntary motor pathway). This originates in the motor areas of the frontal lobe and projects to the brain stem and spinal cord.
Cerebellum
The cerebellum is composed of two large cerebellar hemispheres connected in the midline by the narrow vermis (which is said to resemble a segmented garden worm; Latin: vermis, worm) (Fig. 1.20A). The hemispheres and vermis are further subdivided into anterior and posterior lobes by the primary fissure. The cerebellum also contains a much smaller flocculonodular lobe that is composed of the paired flocculi (Latin: flocculus, tuft of wool) and the nodule of the vermis (see Chs 2 and 3).
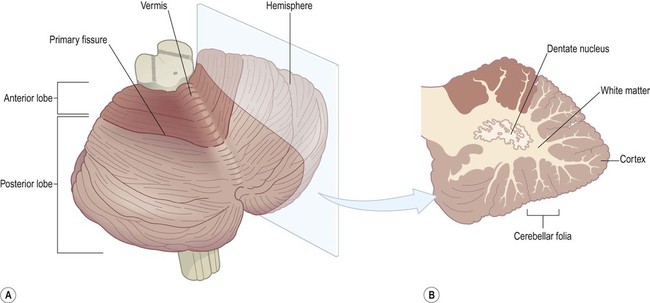
(A) Oblique view of the cerebellum from the posterolateral aspect, showing its intimate relationship to the brain stem. The division of the cerebellar hemispheres and vermis into anterior and posterior lobes is illustrated. The small flocculonodular lobe is not visible in this view (it can only be seen from the anterior aspect); (B) An oblique slice through the cerebellar hemisphere showing the cortex, white matter and dentate nucleus.
Like the cerebrum, the cerebellum also has a folded outer layer of grey matter overlying a central core of white matter. However, the cortex of the cerebellum is arranged in parallel ridges called folia (separated by creases called fissures) and has a comparatively simple three-layered structure. Slicing the cerebellar hemisphere reveals the dentate nucleus embedded in the subcortical white matter (Fig. 1.20B). This is the principal efferent (outflow) nucleus of the cerebellum.
Cerebellar peduncles
The cerebellum is attached to the brain stem (on each side) via three white matter bundles (Fig. 1.21):
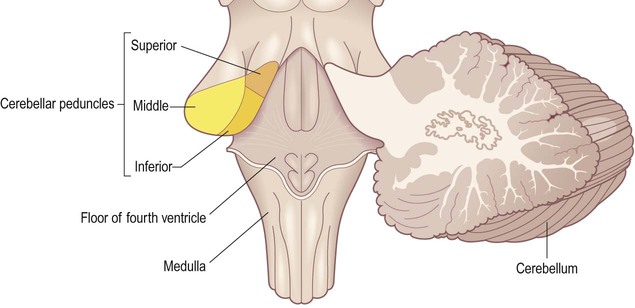
The three cerebellar peduncles have been cut (on the left-hand side) and are therefore seen in transverse section. [NB: The inferior cerebellar peduncle ascends vertically through the medulla and then passes directly backwards into the cerebellum, making a 90-degree bend.]
The superior cerebellar peduncles are attached to the midbrain and contain a major projection from the dentate nucleus to the opposite thalamus and frontal lobe.
The middle cerebellar peduncles arise from the pons and represent the principal afferent supply to the cerebellar hemispheres, which mainly originates from the opposite frontal lobe (see below).
The inferior cerebellar peduncles are connected to the medulla and transmit afferent sensory information from the spinal cord.
The motor and premotor areas of the frontal lobe project to the basal pons (on the same side) via the frontopontine pathway. The pontine nuclei then give rise to transverse pontine fibres which project to the contralateral cerebellar hemisphere (via the middle cerebellar peduncle). Each frontal lobe thus projects to the contralateral cerebellar hemisphere. The cerebellum is therefore ‘uncrossed’ (with respect to the cerebrum) so that unilateral cerebellar damage affects coordination on the same (ipsilateral) side of the body.
Brain stem
The cerebrum and cerebellum are both attached to the brain stem, which is composed of the midbrain, pons and medulla oblongata (discussed separately in Ch. 3).
Taken as a whole, the brain stem can be divided into two longitudinal regions called the base and tegmentum (Fig. 1.22). In other animals, such as rodents, the base of the brain stem lies inferiorly and the tegmentum is above it (Latin: tegmentum, a covering). Since humans are bipedal (and stand upright) the ‘base’ is anterior to the tegmentum.
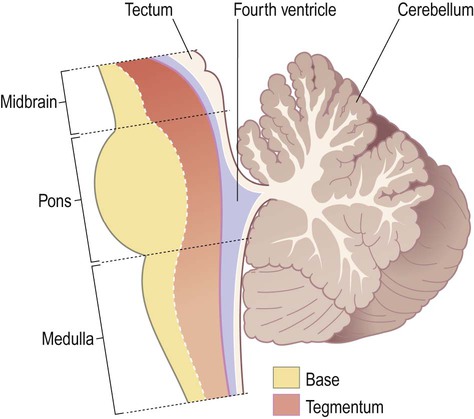
Note that the midbrain also has a ‘roof plate’ (the tectum) that lies dorsal to the cerebral aqueduct, but there is no corresponding region in the pons or medulla.
Reticular formation
The ascending reticular activating system (ARAS) is a diffuse projection that arises from the rostral brain stem. It receives afferents from each of the sensory systems and influences cortical excitability by release of excitatory neurotransmitters including acetylcholine and noradrenaline (Fig. 1.23). Activity in this system is influenced by general and special sensory afferents and is vital for maintaining wakefulness. For this reason, brain stem damage may result in coma (Clinical Box 1.1).
Diffuse neurochemical systems
A number of small brain stem nuclei give rise to extremely diffuse neurochemical projections that influence the entire CNS (Fig. 1.24). These diffuse modulatory systems release the neurotransmitters serotonin, noradrenaline, dopamine and acetylcholine via synaptic terminals distributed throughout the brain and spinal cord. The main pathways travel together between the brain stem and the cerebral hemisphere within the medial forebrain bundle.
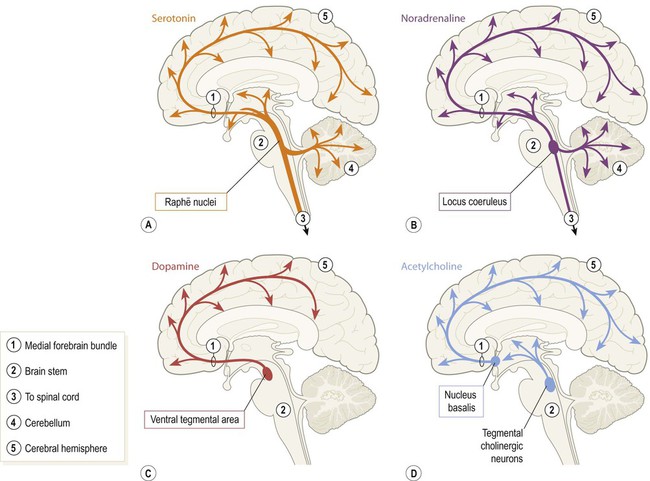
(A) Serotonergic fibres originate in the midline raphē nuclei (pronounced ‘RAFF-ay’); (B) The paired loci coerulei (singular: locus coeruleus) of the rostral pons give rise to noradrenergic fibres; (C) Dopamine is released throughout the cerebral cortex, limbic lobe and amygdala by fibres originating in the ventral tegmental area of the midbrain; the substantia nigra (another midbrain dopaminergic nucleus that is part of the basal ganglia) projects only to the corpus striatum and is not a diffuse projection system; (D) There are several cholinergic nuclei that give rise to diffuse projections, including those within the nucleus basalis (of the basal forebrain) and the tegmentum of the rostral brain stem.
The suffix ‘-ergic’ is used to refer to fibres releasing a common neurotransmitter such that those releasing serotonin are said to be ‘serotonergic’ and those releasing noradrenaline are referred to as ‘noradrenergic’. Coordinated activity in these diffuse projections influences neural functions on a global scale, including arousal, vigilance, sleep–wake cycles and mood (Clinical Box 1.2).
The spinal cord
The spinal cord is a slender continuation of the brain stem which is contained within the bony spinal canal. It is 40–50 cm in length, up to 1.5 cm in width and contains around a billion neurons. A lateral view of the spinal cord shows the cervical and lumbar enlargements which are required for the considerable sensory and motor supply to the upper and lower limbs (Fig. 1.25).
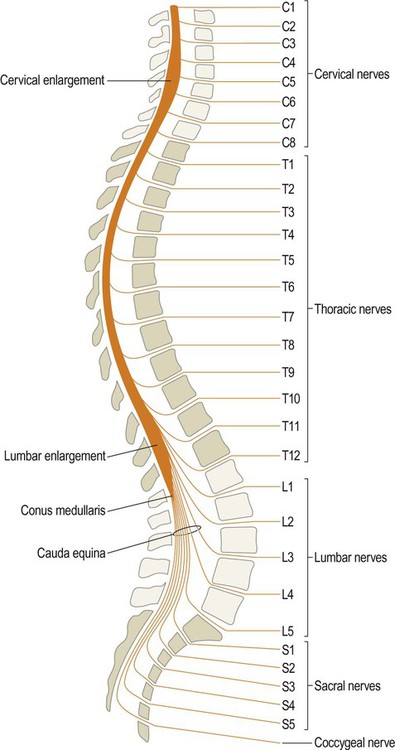
The cervical nerves emerge above their corresponding vertebrae, apart from the C8 nerve which emerges below the seventh cervical vertebra. This is because there are eight cervical nerves but only seven vertebrae. All other spinal nerves (thoracic, lumbar and sacral) exit below their corresponding vertebrae. The spinal cord ends at the conus medullaris (at the level of the L1/L2 disc).
Internal anatomy
The spinal cord contains a central, H-shaped core of grey matter (with dorsal and ventral horns) that is surrounded by a thick layer of white matter. The spinal cord white matter is arranged in three longitudinal columns that contain ascending and descending pathways (Fig. 1.26).
Protective coverings of the CNS
The skull and vertebral column
The skull is composed of the cranium (which encases the brain), together with the facial skeleton and mandible (lower jaw). The brain lies within the cranial cavity, resting on the skull base. It is covered by the dome-like cranial vault. The bones that make up the skull vault are the calvaria (singular: calvarium) (Fig. 1.27). They include the frontal, parietal, occipital and temporal bones, after which the underlying lobes of the cerebral hemispheres are named. The calvarial bones unite at the cranial sutures, but this process is not complete until about 18 months of age.
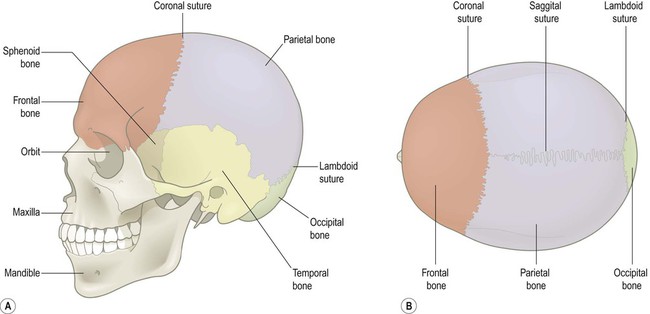
(A) Lateral view; (B) Superior view. The separate bones are united at the cranial sutures.
The spinal cord is protected by the vertebral column, which is composed of multiple separate vertebrae that align to form the hollow vertebral canal. The joints, ligaments and muscles attached to the vertebral column provide the necessary stability and protection for the spinal cord, whilst at the same time permitting a good deal of mobility (Fig. 1.28).
Cranial and spinal meninges
In addition to its bony coverings, the central nervous system is invested by three layers of protective membranes, the meninges (singular: meninx) (Fig. 1.29).
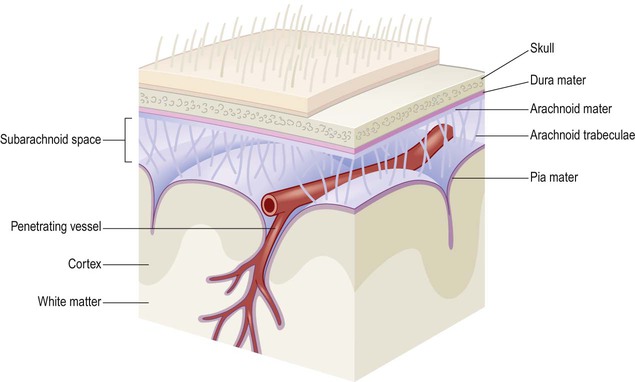
Note that the cerebral arteries occupy the fluid-filled subarachnoid space and that penetrating blood vessels are invested by a sheath of pia mater as they plunge into the CNS tissue.
Subarachnoid space
The arrangement of the three meningeal layers creates the subarachnoid space between the arachnoid and pia. The subarachnoid space is filled with cerebrospinal fluid (CSF) and is in continuity with the cerebral ventricles via three openings at the base of the brain (see Ch. 2). Thus the surface of the CNS is bathed in cerebrospinal fluid, which contains dissolved oxygen and glucose that helps to nourish neural tissues.
The subarachnoid space also has a protective role since it offers buoyancy and cushions against sudden head movements. Further support is provided by fine fibrous connections between the arachnoid and pia (the arachnoid trabeculae) which effectively suspend the brain like puppet strings. Since the CSF is intimately related to the brain and spinal cord it is sometimes sampled clinically to look for evidence of infection, metabolic derangements or tumours (Clinical Box 1.3).
Dural compartments
Double-layered folds of dura form two incomplete partitions inside the skull, dividing it up like the inside of an egg box (Fig. 1.31).
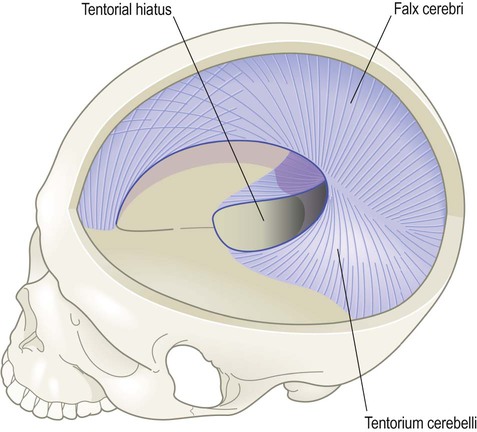
The cranial dura contains a number of blood-filled channels that run along the free and attached margins of the falx cerebri and tentorium cerebelli (Fig. 1.32). These are the dural venous sinuses, into which the cerebral veins empty. The cerebral arteries are discussed in Chapter 10, in the context of stroke.
Subarachnoid cisterns
The depth of the subarachnoid space varies from place to place within the CNS and in some areas is significantly expanded to create a CSF-filled cistern (Fig. 1.33). The largest example is the cisterna magna which is located between the cerebellum and medulla. It is occasionally tapped for a CSF sample when a lumbar puncture proves technically difficult.
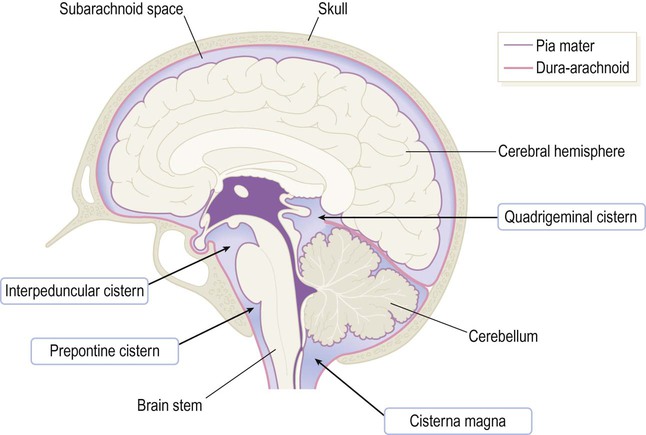
CSF-filled cisterns occur wherever the surface of the brain (covered by pia) is some distance from the overlying skull (lined by dura-arachnoid). The cisterna magna is the largest of the basal cisterns and is also known as the cerebellomedullary cistern.