Chapter 187 Orthoses
Complication Prevention and Management
Spinal bracing encompasses a variety of time-honored techniques that provide external support to injured or diseased segments of the vertebral column. The practice of bracing is as old as medicine itself, having appeared throughout history in the medical and surgical writings of Hippocrates, Galen, Pare, Levacher, and Andry.1–4 In more modern times, Frankel et al.5 showed that bedrest and immobilization can be effective means of achieving bony fusion. The pressures of modern medicine, however, require early mobilization both to decrease hospital length of stay and to minimize medical complications.
Principles of Spinal Bracing
By definition, all orthoses are externally applied devices that apply indirect forces to the spine for correcting or preventing deformity, stabilization, unloading, and/or supportive effects (e.g., massage, warmth, psychological comfort).6 The most common element among these goals is motion restriction.
The Spine as a Column
The manner by which orthoses exert restrictive effects is perhaps best understood in terms of column mechanics. Several authors have described the spine as a complex variant of an ideal column with a fixed base and free upper end.2,7 As a theoretical structure, this “ideal” column is considered a homogeneous rod of constant composition, length, and cross-sectional area. Its behavior when loaded by a balanced axial force has been described mathematically by Euler’s relationship for long-segment column dynamics:
where P is the magnitude of the applied axial load, E is related to the modulus of elasticity of the column material, A is the cross-sectional area of the column, and L is the length8 (Fig. 187-1A). As the structure is progressively loaded, it initially shortens along its longitudinal axis. Once the axial load P exceeds a critical load specific for the column, failure occurs by elastic buckling.7 Methods for stabilizing or increasing the column axial load-bearing capacity involve altering one or more of three variables: column elasticity (E), cross-sectional area (A), or length (L).
Prototypical spinal orthoses consist of two end-fixation elements and a connecting longitudinal member (Fig. 187-1B). An example is the chairback, thoracolumbosacral orthosis (TLSO), which purchases the rib cage with a thoracic band and the hips with a pelvic band. Uprights interconnect the thoracic and pelvic bands.3 Sleeve-type orthoses, such as the clamshell TLSO (see later discussion), incorporate these elements into their circumferential design.1 In terms of column mechanics, braces add to the relative cross-sectional area and the total modulus of elasticity of the spine, thus creating a heterogeneous composite structure that shares the axial load.
Internal segmental fixation applies direct immobilization to spinal segments, while most spinal orthoses apply their forces at some distance from the spine. There is an inverse relationship between the thickness of the soft tissue separating the spine from the inner surface of the orthosis and the resulting effectiveness of immobilization. Conformation of the brace to the body helps to maintain the cylindrical body shell, thereby increasing the stability of the spine.9–12 Also, longer braces provide more stability than shorter ones do; therefore, the length-to-width ratio of the orthotic significantly affects efficacy (see Fig. 187-1A).
Orthoses may also apply balanced transverse forces in a three-point bending arrangement that resists bending forces and thus contributes to axial load bearing.1,8,13 The Jewett brace utilizes the aforementioned biomechanical advantage of a three-point bending force application produced by applying dorsal forces at the sternum and pubis in combination with a ventral force at the affected thoracic or lumbar vertebra.4,11 The long lever arms minimize the force required to produce a sufficient bending moment. Three-point bending also effectively divides the column functionally into two portions of smaller length, increasing the critical failure load of the whole column to that of each segment (Fig. 187-1C).
An underlying principle in long bone splinting is the immobilization of the fractured bone from one joint above to one joint below the site of injury. Extrapolating this concept to the axial skeleton, one may consider it to be composed of five segments, each of which may be considered a long bone (cranial, cervical, thoracic, lumbar, and sacropelvic) (see Figs. 187-1A and B). Therefore, one segment above and one segment below the unstable motion segment would be included in the brace.
Dynamic and Passive Control
All orthoses control spinal motion by a combination of dynamic and passive mechanisms. Dynamic control describes the significant role of intrinsic musculature in actively stabilizing the spine and is the major component in the action of most orthoses. It has been demonstrated experimentally that opposing muscular forces significantly stiffen the spinal column, increasing its load-bearing capacity.9 If isolated from its muscular support, the osseous and ligamentous spinal column holds only 2 kg of axial load before failure by buckling.2 In terms of a column model, muscular action directly affects the modulus of elasticity and relative cross-sectional area of the composite spinal column. Orthoses promote this muscular stabilization by tactile feedback that guides the patient to maintain proper positioning of the body. Pressure at the orthosis-skin contact site creates a reminder to maintain a safe position and limit unwanted gross body motion.4,13–16 The patient therefore is able to prevent undesirable motion of the spine by using only intrinsic muscular support guided by the orthosis. A stiffer, more securely worn appliance is more effective at limiting motion than is a flexible brace because of the heightened sensation of resistance that the stiffer appliance produces. Sypert and others have noted that the effectiveness of an appliance is directly related to the level of its discomfort.3,4 However, this discomfort may also contribute to higher levels of noncompliance.
Passive mechanisms for motion control are important in three-point bending mechanisms and are derived from intrinsic properties of the orthosis itself such as design, size, and material composition. The two common design elements of all orthoses are similar in principle to internal fixation constructs and include end-stabilizing elements (e.g., thoracic bands, pelvic bands) and longitudinal members or uprights that interconnect the end elements.1 Passive mechanisms apply reactive forces to the body that oppose forces resulting from physiologic movements of the head or trunk; viscoelastic forces of ligaments, discs, and muscles; and gravitational force.17 As summarized by White and Panjabi,8 passive mechanical strategies form the basis for most orthotic techniques and include spinal distraction, fluid compression, balanced transverse force application, and skeletal fixation. Most appliances use a combination of techniques for spinal motion control.
Distraction
Distracting the ends of a column is a useful means of correcting or preventing deformity. Its effectiveness depends on the efficiency of transmitting the distracting force directly to the column. Spinal distraction is a major strategy of internal fixation devices used for spine correction and stabilization. This is best illustrated by the Harrington apparatus.7 Distraction is also the technique that is used to reduce acutely unstable spine fractures with tong or halo traction. A braced column in distraction can be considered a composite of an externally applied distracting force plus the axial supporting properties of the original structure.
Distraction orthoses typically act on the head and thorax and cannot directly affect individual vertebral segments. Purchase of the head is either indirect, with pads located at the mandible and the occiput (conventional orthoses), or direct by means of skull pins (halo-skeletal fixation). Thoracic purchase is obtained at the sternum and rib cage through a combination of pads, straps, or vest attachment. The effectiveness of a distraction orthosis depends on the efficiency of force transmission to the vertebral segment of interest and the mechanical rigidity of the orthosis material itself.13 Inefficiency in transmitting external force to the spine has been termed “the transmitter problem” by White and Panjabi8 and represents the loss of energy that occurs when force is applied to “low stiffness, viscoelastic” structures such as overlying soft tissues, intervening normal joints, and ligaments. In the cervical area, distraction applied to the mandible is compromised by cushioning effects of soft tissue under the chin, the temporomandibular joint, cervical muscle tone, the C0-1 articulation, and each successive segmental articulation above the level at which the force is to have its effect (level of pathology). A more rigid brace with a tighter fit improves the efficiency of transmitting force by compressing intervening soft tissue.17 These factors paradoxically increase the risk of pressure injury to overlying soft tissues.18 Skeletal fixation improves the effectiveness of force transmission by directly purchasing the skull, minimizing the risk of pressure injury in the head and neck region.
Point-of-contact problems also exist with thoracolumbar braces that involve the shoulder girdle, pectoral muscles, rib cage, and upper abdomen. The shoulders have a significant amount of overlying skin, fat, and muscle and are by definition highly mobile structures involved in arm movement. Because of this mobility, orthoses that rely on shoulder straps or pads to apply a counterforce cannot consistently distract the spine.13 Changes in body position from sitting to supine also produce shoulder movement contributing to the difficulty of spinal distraction. Koch and Nickel19 studied this effect in six patients wearing the halo apparatus by measuring the forces of distraction and compression exerted through the device with an attached strain gauge. Distraction force varied by more than 20 pounds in a halo vest and 30 pounds in a halo cast when patients changed from supine to sitting positions. Similar variations in distraction with the halo device were noted during shoulder shrugging, coughing, sneezing, and deep breathing. Shoulder purchase is thus a highly variable means of anchoring the caudal end of a distraction orthosis.
Appliances with pads overlying the pectoral areas are compromised by the energy-absorbing effects of fat, muscle, and breast tissue. Movement of the chest occurs with arm motion in a manner similar to that of the shoulders. Although the rib cage is generally a stable structure, deep breathing, coughing, and sneezing produce significant motion that is directly transmitted by all devices purchasing the thorax. Orthoses extending below the thorax to the upper and lower abdomen are at an even greater disadvantage because of the highly elastic nature of this fluid- and air-filled region.8
Fluid Compression
Fluid compression refers to the ability of a tight circumferential binder, such as a corset, to compress partially fluid-filled soft tissues surrounding the spine, thus creating a fluid cylinder.6,8 Because of its mechanical incompressibility, a fluid-filled cylinder has axial load-bearing capacity. For a column model, this technique increases the aggregate cross-sectional area by converting soft tissues into load-bearing structures. Several studies have directly measured the effect of abdominal and thoracic cavity compression, noting little effect of compression on intra-abdominal pressure.6,9,12 In Nachemson and Morris’s20 classic report, however, a 25% reduction in intradiscal pressure was observed in lumbar segments that were braced with an inflatable abdominal corset. The true unloading effect of fluid compression is thought to be a minor factor for orthotic thoracolumbar stabilization and is beneficial only for restricting sagittal plane motion.6 Fluid compression is a strategy that is not applicable for the cervical spine, in which airway, vascular, and muscular tissues make up a relatively large proportion of the cross-sectional area of the neck and do not tolerate significant compression.4
Transverse Loading
Balanced transverse loading describes a common and effective strategy for restricting spinal rotation and translation. Orthoses typically use a three-point bending force application arrangement with two horizontal reactive forces applied at the ends of the column in one direction and a third balancing force in the opposite direction at the fulcrum of the deformity8,13 (see Fig. 187-1C). Because the system is in equilibrium, the sum of all horizontal forces is zero. This prevents translation. Similarly, bending moments generated by the applied forces acting at the axis of rotation for the injured segment also equal zero if rotational motion is adequately controlled.13 Keys to an effective transverse loading strategy include (1) identifying the axis of rotation at the level of injury or point of instability by using an appliance that is centered at or near this axis of rotation and (2) using an adequately long appliance that maximizes the length of the applied moment arm to control the spinal segment of interest.13
Classification
Spinal orthoses are generally considered either conventional orthotics or skeletal fixators. Conventional devices are contact-type orthoses that control spinal motion through direct contact with the skin and soft tissues of the head, neck, thorax, abdomen, or pelvis. A contact orthosis may have only limited skin contact through discrete pads and straps or extensive surface area coverage (e.g., total contact orthosis). Examples of total contact devices are the Yale brace21 and the molded clamshell TLSO (see later discussion). Advantages of newer contact orthoses that use thermoplastic materials include ease of application, light weight, warmth, ventilation, ability to be removed for optimal hygiene, and patient acceptance.22,23 All conventional devices provide some control of flexion and extension but are more limited in reducing lateral bending and rotation.24 Additional drawbacks include poor patient compliance, excessive warmth causing sweating, variability of fit, and complications of skin or soft tissue contact. All devices have limitations that must be balanced with their advantages. Each segment of the spine must be considered individually because of the variability of anatomy, mobility, and applicability of an orthosis.
Harris25 reported the results of a consensus task force of orthotists, spine surgeons, and other health officials who set forth a common nomenclature for conventional spinal orthoses with the intent of standardizing communication among spine professionals and avoiding the plethora of eponyms describing individual appliances (Table 187-1). In this scheme, devices are classified as cervical orthoses (CO), cervicothoracic orthoses (CTO), TLSO, lumbosacral orthoses (LSO), or sacroiliac orthoses (SIO). This classification, which was intended to reflect the region of the spine that is immobilized by the device, has become the standard nomenclature of spinal orthotics since 1973. Krag16 has recently expanded the cervical classification into four subcategories based on the specific anatomy of the region: cervical (CO), occipital-mandibular-cervical (OMC), occipital-mandibular-high thoracic (OMHT), and occipital-mandibular-low thoracic (OMLT).
Appliance Category | Examples |
---|---|
Cervical orthoses | |
Cervical collars | Foam collar |
Occipital-mandibular-cervical | Thomas collar, Queen Anne collar |
Occipital-mandibular-high thoracic | Philadelphia collar, Miami J collar, Guilford two-poster brace, four-poster brace |
Cervicothoracic orthoses | Yale brace, Minerva brace, SOMI (sternal-occipital-mandibular immobilizer) brace |
Thoracolumbosacral orthoses | Clamshell thermoplastic body jacket, Jewett extension brace, Boston overlap brace |
Lumbosacral orthoses | Lumbosacral corset, chairback orthosis, Knight brace |
Sacroiliac orthoses | Sacroiliac corset with perineal straps |
Halo devices | Vest halo, four-pad halo, thermoplastic Minerva body jacket |
Cervical Orthoses
COs are basically soft foam or felt collars with minimal purchase of the mandible or occiput16 (Fig. 187-2A). These collars are light, inexpensive, easy to use, and relatively comfortable to wear. Regrettably, they offer negligible resistance to cervical motion in any plane of motion, functioning only to remind patients to limit voluntary extremes of neck movement.26–28 Because they are only supportive, cervical collars are inappropriate for patients with bony instability. They can provide tactile generated support of cervical musculature and psychological comfort in cases of myofascial strain or sprain or in straightforward postoperative patients without instability.23
Occipital-Mandibular-Cervical Orthoses
OMC orthoses are hard plastic collars that are more rigid than foam collars and offer slightly better purchase of the mandible or occiput16 (Fig. 187-2B). Most usage is as a prophylactic measure, in conjunction with a backboard, in acute trauma situations. They have no thoracic extension; therefore, while offering an improved cranial point of fixation, they lack a true caudal one. The addition of an adjustable chin or occipital piece increases resistance to flexion or extension to a mild degree.26 Lateral bending and rotation are poorly controlled with these braces.24 Like the soft collars, OMC orthoses do not provide significant immobilization to the cervical spine and are not recommended for patients with instability.
Occipital-Mandibular-High Thoracic Orthoses
Examples of OMHT orthoses are the widely used Philadelphia collar, the four-poster brace, the two-poster brace (e.g., Guilford, Duke), and several newly available hard plastic collars16 (Figs. 187-2C–F). All OMHT appliances control head movement with occipital and mandibular supports and have better upper thoracic purchase than collars because of longer length. Like the collars, they are relatively easy to apply, are lightweight, and are relatively inexpensive.
The popular Philadelphia collar is a Plastazote foam device reinforced with hard plastic. It is available in different sizes and consists of front and back halves connected by Velcro straps.3 Although generally well tolerated, it can be quite hot to wear, causing significant sweating and secondary skin maceration. The Plastazote material is less rigid than other OMHT appliances such as the poster orthoses.29 Despite its limitations, Johnson et al.24 found this device to be as effective in controlling upper (C0-3) flexion-extension as the rigid OMHT orthoses. It was less effective in restricting flexion-extension in the midcervical and lower cervical segments (Fig. 187-3) and poor in controlled rotation and lateral bending at all levels. The device is frequently used for patients with mild cervical injuries and in postoperative patients with minor instability. Polin et al.30 evaluated the use of the Philadelphia collar and the halo device in odontoid fractures. Their findings indicated no significant difference in the rate of fracture healing between the two orthoses for both type II and type III fractures and suggested that the less invasive collar may be adequate in this setting. Modifications of the Philadelphia collar have been developed that maintain cervical immobilization yet improve the comfort and convenience of a plastic removable collar. In 2009, Koller et al.31 did an in vivo analysis of atlantoaxial motion in 20 healthy volunteers, comparing the halo thoracic vest and the Philadelphia collar. They found that, as expected, the absolute range of motion of the subaxial spine was more restricted by the halo vest than that of the Philadelphia collar. However, they found that the atlantoaxial complex had a tendency to be more restricted in sagittal motion between extreme flexion and extension in the Philadelphia collar (mean, 1.3 degrees) than in the halo vest (mean, 3.3 degrees). The author subsequently suggested that a Philadelphia collar might be sufficient in the conservative treatment of stable odontoid fractures.
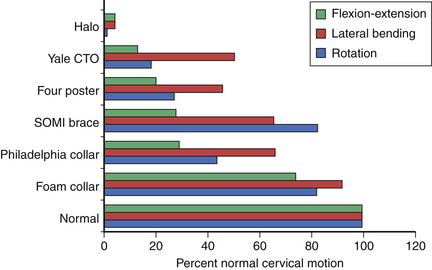
FIGURE 187-3 Overall motion allowed in cervical orthoses.
(From Johnson RM, Hart DL, Simmons EF, et al: Cervical orthoses: a study comparing their effectiveness in restricting cervical motion in normal subjects. J Bone Joint Surg [Am] 59:332–339, 1977.)
Schneider et al.32 did a biomechanical evaluation of common cervical orthoses. They looked at reduction in head and intervertebral motion provided by seven contemporary cervical orthoses in 45 individuals. They evaluated the overall range of motion of the head in three planes as well as intervertebral motion in the sagittal plane. The tested subjects also reported comfort for each brace being tested.
With respect to patient-reported comfort, there was a significant association between the type of brace and patient-reported brace comfort score. The most comfortable orthoses were the Miami J and the Aspen. In general, the cervical collars were more comfortable than the cervicothoracic orthoses32 (Fig. 187-4A).
With respect to the motion restriction of the head, all cervical braces significantly reduced overall sagittal plane flexion-extension motion of the head as well as axial rotation and coronal plane side-to-side bending. As was expected, however, some braces reduced motion significantly more than others did32 (Fig. 187-4B).
The intervertebral motion was measured from the fluoroscopic images of maximum flexion and extension; all braces significantly reduced intervertebral rotation at all levels. Some of the braces reduced rotation more than others, and the differences between braces were more pronounced at the midcervical than the upper or lower cervical levels32 (Fig. 187-4C).
Occipital-Mandibular-Low Thoracic Orthoses
The OMLT orthoses are represented by the sternal-occipital-mandibular immobilizer (SOMI), the extended Philadelphia collar (Yale brace), and the Minerva brace (Figs. 187-2G–I). These devices are essentially longer versions of the OMHT appliances with the addition of circumferential chest straps. In general, they are the most rigid of the conventional orthoses because of their increased length and better thoracic purchase24,29 (see Figs. 187-3 and 187-4). Advantages of the OMHT orthoses include best immobilization of flexion-extension and rotation of the conventional braces, noninvasiveness, and ease of application. However, they are more cumbersome and uncomfortable to wear than the smaller appliances. With the exception of the Yale brace, they are moderately expensive and still have limited control of lateral bending.21,24
Thoracolumbosacral Orthoses
Representative TLSOs include the clamshell thermoplastic body jacket and the thoracolumbar extension orthosis or Jewett brace (Figs. 187-5A–C). There are a number of modifications of these two basic designs. By definition, these appliances apply three-point bending forces at the upper thorax and pelvis and at the midportion of the brace across the thoracolumbar junction.1 This class of orthotic is best suited for restricting thoracolumbar and lumbar gross-body motion33 and poorly controls low lumbar and sacral segments.14 Molded appliances are particularly useful for thoracolumbar junction trauma in which the total contact feature helps to control lateral bending and rotation. Velcro straps and thermoplastic materials have greatly improved the ease of wear and comfort of TLSOs in recent years, improving both compliance and effectiveness.
Jewett braces primarily control flexion-extension and are therefore often used for minor flexion compression injuries such as stable compression fractures.3 More severe thoracolumbar injuries were recently studied by Patwardhan et al.34 with a finite element computer model. The Jewett brace was effective at preventing deformity under physiologic flexion loading with injuries that resulted in less than 50% normal spinal stiffness (single-column injuries). With injuries that reduced stiffness to between 50% and 85% (two-column injuries) and those greater than 85% (three-column injuries), the brace was ineffective for resisting spinal deformation.34
Lumbosacral Orthoses
Perhaps the most frequently prescribed appliances, LSOs are also the most controversial because of their questionable effectiveness.6 Lumbosacral corsets with or without stays, chairback braces, and the Knight brace are representative3 (Fig. 187-5D). LSOs stabilize the lumbar and sacral regions by encircling the upper abdomen and rib cage and the pelvis. Because of the difficulty in firmly purchasing these areas, three-point bending is probably not a major mechanism in the action of this class of orthosis. Fluid compression likely has a role, although measurements of intra-abdominal pressure while wearing the devices have been inconsistent.9
To quantify the effect of bracing on segmental motion, Norton and Brown11 measured lumbar motion in volunteers by following the motion of K-wires inserted into the spinous processes. Movement at the lumbosacral junction was paradoxically greater while sitting with an LSO than without the brace. The effect was thought to be secondary to stress concentration at the caudal end of the supported segment. Waters and Morris12 studied lumbosacral stabilization in orthoses by monitoring paraspinal muscle electromyography. Although back muscle activity during standing was reduced with an LSO, activity during fast walking was greater, possibly because of altered pelvis rotation. Lantz and Schultz33 measured gross trunk motion and myoelectric activity in five volunteers wearing the lumbosacral corset, a chairback brace, and a molded TLSO during standing and sitting. Gross upper body motion was reduced up to 20% in flexion and 45% in extension, lateral bending, and rotation. The TLSO was overall the most effective device tested. Myoelectrical activity varied widely, however, ranging from a 9% reduction to a 44% increase.35 Axelsson et al.14