Chapter 121 Omental Transposition and Spine Surgery
Emphasis on Revascularization and Scar Prevention
Editor’s note: In this chapter, the author has provided his unique perspective on an equally unique population of patients with impaired spinal cord function. Some, including Clifton et al. (Omental transposition in chronic spinal cord injury, Spinal Cord 34:193–203, 1996), have discounted the author’s work, while others find it intriguing. Regardless of the reader’s opinion, it is included in this book in an attempt to cover all aspects of care. The reader also is referred to Chapter 72 in this book for an in-depth discussion of pharmacologic and regenerative therapeutic acute spinal cord injury strategies.
Pathophysiology of Spinal Cord Injury
One of the most important factors associated with an SCI is the production of vasogenic edema and extravasated blood that occurs rapidly at the site of the injury. A traumatized SC decreases the amount of localized edema that accumulates at the site of the injured SC by the dynamic movement of the fluid up and down the SC.1 This compensatory action within the SC, however, usually is inadequate to decrease the high interstitial pressure that is caused by fluid accumulation at the site of the SCI. The swelling of the SC caused by this fluid accumulation continues within the confines of the nonyielding dura mater and the rigid spinal canal. When the interstitial pressure within the SC becomes excessive due to swelling, venous compression results. This action results in an elevated upstream venous pressure, which further enhances the capillary extrusion of blood from the porous blood vessels at the injury site. This situation causes an increase in focal osmotic pressure, which leads to a further increase in fluid accumulation. The high interstitial pressure within the SC also compresses small capillaries in the region of the SCI, which can lead to the progressive loss of capillary perfusion. This eventually can lead to complete blockage of vascular flow, causing the destruction of viable neural tissue in the area of the SC impaction.
The inability of edema fluid and blood to be absorbed at the site of an SCI is due to the absence of a lymphatic system in the SC. Without the absorbing mechanisms of a lymphatic system, edema fluid and blood remain at the SCI site, and the fluids eventually result in fibrosis scarring. This scarring is thought to be caused by the fibrinogen that is present in the blood that leaks from injured SC blood vessels. The fibrinogen apparently becomes activated at the SC injury site to make fibrin scar, which causes compression on blood vessels, a decrease in SC blood flow, and the blockage of axons from penetrating through the tissue (Fig. 121-1).
If healing of an acute SCI is to take place, it would appear that action should be undertaken as early as possible after injury to limit edema and blood accumulation resulting from the SCI. It has been shown that if an intact omentum pedicle is placed directly on an SC shortly after injury, absorption of edema and blood can be accomplished with little, if any, fluid accumulation or scar formation (Fig. 121-2), due to the enormous ability of the omentum to absorb fluids.2,3 Placing the omentum directly on an injured SC apparently establishes a dynamic equilibrium between the production of blood and edema by the traumatized SC and the absorption of these fluids by the overlying omentum. Such absorption by the omentum not only decreases interstitial pressure in the area of the SCI, but, most importantly, its absorptive capability decreases the amount of blood (fibrinogen) in the injured area, thereby lessening the potential for scar formation at the injury site.
To apply the omentum directly onto an SCI location, a laminectomy is required, followed by opening of the dura mater. These maneuvers alone have a beneficial effect on an SCI, because they allow the injured SC to expand, thereby lowering the high interstitial pressure in the SC, which promotes capillary perfusion to increase in the area of the SC injury. It cannot be overemphasized that in an acute SC it is not sufficient to simply surgically stabilize the vertebral column. It appears to be necessary to open the dura mater and treat the SC directly by applying the omentum onto the injured SC, thus allowing edema absorption. This procedure must be done shortly after injury (within a few days), because a fibrotic process begins within a week of injury.
Spinal Cord Revascularization
As with any type of wound, adequate blood supply to the involved area is essential to optimize healing. Research has shown that when the omentum is laid directly on an injured SC, vascular connections penetrate from the omentum into the SC within hours.4 This ability to revascularize the SC by the omentum was clearly proven by injecting India ink into an artery located within an intact omental pedicle that had been placed directly on a normal SC. The injected dye marker was subsequently observed on the surface of the SC, as well as in the deepest positioned capillaries that are located within the SC. It subsequently was observed in laboratory animals that omental blood vessels revascularize the SC rapidly and that a sizeable amount of blood was delivered to an uninjured SC through these omental vessels. The revascularization process was found to be even more rapid when the omentum was placed directly on an injured SC (Fig. 121-3). It is important, however, that the omentum be placed on the SCI as early following the injury as clinically possible.5
Spinal Cord Scarring
Clinical SCI research today is aimed primarily at determining how to improve treatment results. This is of critical importance because there has been no significant improvement in the surgical approach to such injuries over the years. It has been found, however, that absorption of blood and edema by the omentum at the site of an SCI in animals has led to functional improvement, suggesting that this approach might have a comparable beneficial effect in humans.
It is known that in animals with an acute SCI, laying an omental pedicle graft on an injured SC facilitates the absorption of blood and edema.6 The importance of this absorptive capability is that it impedes the development of scar tissue, a step that appears to be critical in preventing the evolution of scarring in the injured SC. The idea that scar tissue markedly hinders SCI improvement is not new. Ramon y Cajal claimed over 100 years ago that functional regeneration in the SC could be accomplished if physical obstacles to central neuritic outgrowth could be removed.7 The critical significance of scar being the physical obstacle preventing axons from penetrating through scar tissue was later brought into clearer focus by the work of Freeman at the University of Indiana in the 1950s. He clearly demonstrated that scar tissue at an SCI site prevented axons from penetrating through and making connections with neural structures in the caudal cord. Freeman wrote “that axons have a relentless compulsion to grow until they participate in return of function. If axonal regrowth is blocked by scar tissue, axons continue to grow in circles to form neuromata.”8 Cajal’s and Freeman’s observations strongly indicate that if scar can be eliminated from the healing process within an injured SC, functional and neuroelectrical improvement might well occur.
There appears to be no question that adequate blood supply and prevention of scar formation are crucial if SC healing is to take place. As far as revascularization is concerned, the ability of the omentum to carry out increased angiogenic activity in any area of the body is well known, especially since it has been shown that the highest concentration of vascular endothelial growth factor, the most angiogenic substance in the body, is in omental tissue.9 Although revascularization of an injured SC obviously is important, scarring within the SC may well turn out to be the critical issue if SC healing is to occur.
Blood flow measurements in the SC of animals with the omental-collagen bridge and in control animals were taken just before sacrifice, 90 days after surgery. The SC blood flow in omental-collagen animals demonstrated a 59% increase compared with the SC blood flow in normal (nontransected) control animals.10 Also noted was a 3-to-1 increase in the blood vessel density counts in the omental-collagen–treated cats as compared with normal controls.11
Of particular importance in the omental-collagen bridge animals was the presence of dopaminergic and noradrenergic fibers that penetrated through the biodegrading collagen bridge with continued growth of the fibers into the distal SC stump12 (Fig. 121-4). The axons were found to have grown at a rate of 1 mm per day for a maximum distance of 90 mm during the cats’ 12-week recovery period. This penetration of axons occurred because of the absence of scar tissue.
The finding that axons grew through the omental-collagen preparation was considered extremely important, but it was necessary to demonstrate whether these penetrating axons actually made synaptic connections with distal neural elements. Such penetration and connections of these axons were proven to occur by the use of the axonal marker hydroxystilbamidine (Fluoro-Gold; Fluorochrome, LLC, Denver, CO). When this dye marker was injected into the distal SC of a normal cat, the dye followed a retrograde axonal pathway up to the brain and was clearly seen in the cytoplasm and processes of neurons located in the locus coeruleus, subcoeruleus, Kolliker-Fuse nucleus, and substantia nigra. However, when cats simply had their SCs transected, the injection of Fluoro-Gold into their SCs caudal to the transection did not travel retrograde up axonal pathways toward the brain and was never observed in various locations within their brains. In contrast, when Fluoro-Gold was injected into the distal SC of cats in the presence of an omental-collagen bridge created after SC transection, Fluoro-Gold was found in the brain in the same locations as the injected Fluoro-Gold found in the brains of nontransected animals13 (Fig. 121-5).
Histologic studies of the SCs of animals that had undergone omental-collagen reconstruction following SC transection were carefully evaluated. They showed that following the exposure of these SCs to the synaptogenic marker synaptophysin, this marker was found adjacent to preganglionic synaptic neurons located rostral and caudal to the omental-collagen bridge.11 This finding indicated that synaptic remodeling (regeneration) by previously denervated supraspinal axons had occurred. Both the Fluoro-Gold and synaptophysin observations strongly suggested that following complete SC transection in cats, an omental-collagen bridge inserted at an SC transection site allowed disconnected supraspinal fibers to regenerate over long distances and apparently engage in synaptic remodeling with target tissue located at a distant site.
It is uncommon to encounter a patient with a transected SC. Interestingly, however, several years after successful omental-collagen bridging in transected SCs in animals, a 23-year-old woman who had suffered an SCI in a high-speed skiing accident 3 years earlier was treated with this technique. An MRI taken shortly after that injury showed a complete anatomic transection of her SC at the T6-7 level (Fig. 121-6). Over the next 3 years she continued to exhibit complete motor and sensory loss below this level.
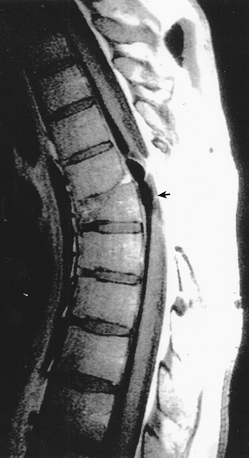
FIGURE 121-6 MRI taken shortly after injury reported as complete anatomic transection at the T6-7 level (arrow).
Over the next several years, the patient regained extensive motor activity following intensive physical therapy. At the end of 4 years she could walk, but required the use of a walker (Fig. 121-7). Serial MRIs were taken of her SC on a yearly basis, and after 4 years the separated SC on MRI examination was shown to have anatomically reconstructed itself in an incontinuity longitudinal connection (Fig. 121-8). This case has been carefully documented.14
Summary
The aim of SCI research and clinical care is to find new approaches that will improve the functional outcome of patients with an SCI.6 An adequate blood supply is necessary for healing of an SCI to take place. Such an additional source of blood for a healing SCI can be provided by placing an omental pedicle on an acute SCI. Of even greater importance in improving the treatment of an SCI is finding a way to limit the production of scar tissue within the SC following an SCI. Such scar tissue has been known for many years to be a major physical obstacle that prevents axons from penetrating through the scar. Such action blocks connections of axons to neural tissue in the distal SC. Because the omentum can revascularize the SC and limit scarring within the SC, it seems possible that it would improve the results following surgical treatment of an acute SCI. It seems reasonable to suggest that clinical evaluation of omental transposition be undertaken, because its success could generate the clinical improvement that we continue to seek for patients who sustain an SCI.
de la Torre J.C., Goldsmith H.S. Supraspinal fiber outgrowth and apparent synaptic remodeling across transected-reconstructed feline spinal cord. Acta Neurochir. 1992;114:118-127.
de la Torre J.C., Goldsmith H.S. Coerulospinal fiber regeneration in transected feline spinal cord. Brain Res Bull. 1994;35:413-417.
Goldsmith H.S. Treatment of acute spinal cord injury by omental transposition: a new approach. J Am Coll Surg. 2009;208:289-292.
Goldsmith H.S., de la Torre J.C. Axonal regeneration after spinal cord transection and reconstruction. Brain Res. 1992;589:217-224.
Goldsmith H.S., Duckett S., Chen W.F. Spinal cord revascularization by intact omentum. Am J Surg. 1975;129:262-265.
Goldsmith H.S., Fonseca A.Jr., Porter J. Spinal cord separation: MRI evidence of healing after omental-collagen reconstruction. Neurol Res. 2005;27:115-123.
Ramon y Cajal S., May R., editor. Degeneration and regeneration of the nervous system. New York: Hafner Publishing. 1950:749-750.
1. Nemecek S.T., Peter R., Suba P., et al. Longitudinal extension of edema in experimental spinal cord injury. Acta Neurochir. 1977;37:7-16.
2. Goldsmith H.S., de los Santos R., Beattie E.J. The relief of chronic lymphedema by omental transposition. Ann Surg. 1967;166:571-585.
3. Levander B., Zwetnow N.W. Bulk flow of CSF through a lumbo-omental pedicled graft in the dog. Acta Neurochir. 1978;41:147-155.
4. Goldsmith H.S., Duckett S., Chen W.F. Spinal cord revascularization by intact omentum. Am J Surg. 1975;129:262-265.
5. Goldsmith H.S., Steward E., Duckett S. Early application of pedicled omentum to the acutely traumatized spinal cord. Paraplegia. 1985;23:100-112.
6. Goldsmith H.S. Treatment of acute spinal cord injury by omental transposition: a new approach. J Am Coll Surg. 2009;208:289-292.
7. Ramon y Cajal S., May R., editor. Degeneration and regeneration of the nervous system. New York: Hafner Publishing. 1950:749-750.
8. Heimburger R.F. Is there hope for return of function in lower extremities paralyzed by spinal cord injury. J Am Coll Surg. 2006;202:1001-1004.
9. Zhang Q.X., MaGovern C.J., Mack C.A., et al. Vascular endothelial growth factor is the major angiogenic factor in omentum: mechanisms of the omentum-mediated angiogenesis. J Surg Res. 1997;67:147-154.
10. Goldsmith H.S., de la Torre J.C. Axonal regeneration after spinal cord transection and reconstruction. Brain Res. 1992;589:217-224.
11. de la Torre J.C., Goldsmith H.S. Collagen-omental graft in experimental spinal cord transection. Acta Neurochir. 1990;102:152-163.
12. de la Torre J.C., Goldsmith H.S. Coerulospinal fiber regeneration in transected feline spinal cord. Brain Res Bull. 1994;35:413-417.
13. de la Torre J.C., Goldsmith H.S. Supraspinal fiber outgrowth and apparent synaptic remodeling across transected-reconstructed feline spinal cord. Acta Neurochir. 1992;114:118-127.
14. Goldsmith H.S., Fonseca A.Jr., Porter J. Spinal cord separation: MRI evidence of healing after omental-collagen reconstruction. Neurol Res. 2005;27:115-123.