34 Olfactory and limbic systems
Olfactory System
The olfactory system is remarkable in four respects:
Olfactory epithelium
The olfactory epithelium occupies the upper one-fifth of the lateral and septal walls of the nasal cavity. The epithelium contains three cell types (Figure 34.1):
Olfactory bulb (Figure 34.1)
Central connections
Mitral cell axons run centrally in the olfactory tract (Figure 34.2). The tract divides in front of the anterior perforated substance into medial and lateral olfactory striae.
Points of clinical interest are mentioned in Clinical Panel 34.1.
Limbic System
The limbic system comprises the limbic cortex (so-called limbic lobe) and related subcortical nuclei. The term ‘limbic’ (Broca, 1878) originally referred to a limbus or rim of cortex immediately adjacent to the corpus callosum and diencephalon. The limbic cortex is now taken to include the three-layered allocortex of the hippocampal formation and septal area, together with transitional mesocortex in the parahippocampal gyrus, cingulate gyrus, and insula. The principal subcortical component of the limbic system is the amygdala, which merges with the cortex on the medial side of the temporal pole. Closely related subcortical areas are the hypothalamus, reticular formation, and the nucleus accumbens. Cortical areas closely related to the limbic system are the orbitofrontal cortex and the temporal pole (Figure 34.3).
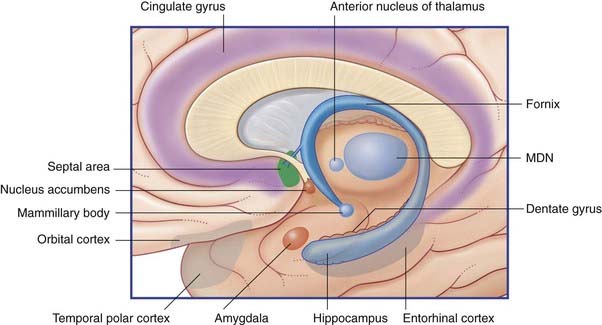
Figure 34.3 Medial view of cortical and subcortical limbic areas. MDN, mediodorsal nucleus of thalamus.
Figure 34.4 is a graphic reconstruction of mainly subcortical limbic areas.
Hippocampal complex
The hippocampal complex (or hippocampal formation) comprises the subiculum, the hippocampus proper, and the dentate gyrus (Figure 34.5). All three are composed of temporal lobe allocortex which has tucked itself into an S-shaped scroll along the floor of the lateral ventricle. The band-like origin of the fornix from the subiculum and hippocampus is the fimbria. The hippocampus is also known as Ammon’s horn (after an Egyptian deity with a ram’s head). For research purposes, it is divided into four cornu ammonis (CA) zones (Fig. 34.6A).
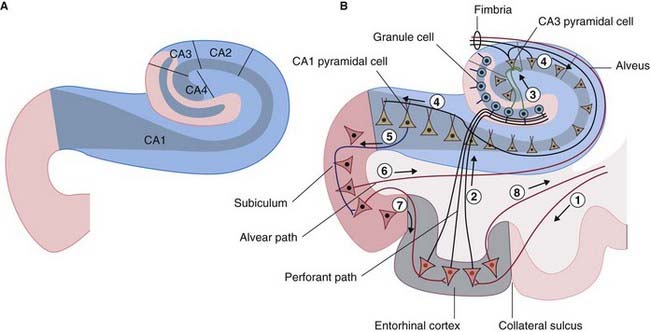
Figure 34.6 (A) The four sectors of Ammon’s horn. (B) Input–output connections of the hippocampal formation.
Connections
Afferents
The largest afferent connection of the hippocampal complex is the perforant path, which projects from the entorhinal cortex onto the dendrites of dentate granule cells (Figure 34.6B). The subiculum gives rise to a second, alvear path which contributes to a sheet of fibers on the ventricular surface of the hippocampus, the alveus.
Auditory information enters the hippocampus from the association cortex of the superior and middle temporal gyri. The supramarginal gyrus (area 40) transmits coded information about personal space (the body schema described in Ch. 32) and extrapersonal (visual) space. From the occipito-temporal region on the inferior surface, information concerning object shape and color, and facial recognition, is projected to cortex called perirhinal, or transrhinal, immediately lateral to the entorhinal cortex. From here, it enters the hippocampus. A return projection from entorhinal to perirhinal cortex is linked to the temporal polar and prefrontal cortex.
Clinical Panel 34.2 Schizophrenia
Medications used to treat psychotic disorders such as schizophrenia are called antipsychotics, neuroleptics or major tranquillizers. All such antipsychotic medications block dopamine D2 receptors to some extent (e.g. haloperidol or olanzapine). In the normal brain, the D2 receptors are on spiny (excitatory) stellate cells in the mesocortical projection territory of the ventral tegmental nucleus. D2 receptors are inhibitory, for one or more of three possible reasons noted in Chapter 8. Interestingly, symptoms closely resembling the positive psychotic ones of schizophrenia may be induced by consuming excessive amounts of dopamine-stimulating drugs such as cocaine or amphetamine (’speed’). Amphetamine is known to increase the amount of dopamine in the forebrain extracellular space (Clinical Panel 34.5). In schizophrenia, dopaminergic overactivity seems not to be a matter of overproduction but of greater effectiveness through an increased number of postsynaptic dopamine receptors on the spiny stellate neurons. (The assistance of Professor Colm McDonald, Department of Psychiatry, NUI, Galway, Ireland is gratefully appreciated.)
Harrison PJ, Weinberger DR. Schizophrenia genes, gene expression, and neuropathology: on the matter of their convergence. Mol Psychiatry. 2005;10:40-68.
Leonard BE. Drug treatment of schizophrenia and the psychoses. In: Fundamentals of psychopharmacology. Chichester: Wiley; 2003:255-294.
Picchioni MM, Murray RM. Schizophrenia. BMJ. 2007;335:91-95.
Efferents
The largest efferent connection is a massive projection via the entorhinal cortex to the association areas of the neocortex. A second, forward projection is the fornix (Figure 34.5A). The fornix is a direct continuation of the fimbria, which receives axons from the subiculum and hippocampus proper. The crus of the fornix arches up beneath the corpus callosum, where it joins its fellow to form the trunk and links with its opposite number through a small hippocampal commissure. Anteriorly, the trunk divides into two pillars. Each pillar splits around the anterior commissure, sending precommissural fibers to the septal area and postcommissural fibers to the anterior hypothalamus, mammillary body, and medial forebrain bundle. The mammillary body projects into the anterior nucleus of thalamus, which projects in turn to the cingulate cortex, completing the Papez circuit from cingulate cortex to hippocampus, with return to cingulate cortex via fornix, mammillary body, and anterior thalamic nucleus (Figure 34.7).
The term medial temporal lobe is clinically inclusive of the hippocampal complex, parahippocampal gyrus, and amygdala. The term is most often used in relation to seizures (Clinical Panel 34.3).
Clinical Panel 34.3 Temporal lobe epilepsy
Complex focal (partial) seizures are synonymous with temporal lobe epilepsy. The initial event, or aura, may be a simple partial seizure whose electrical activity escapes into the temporal lobe. Many originate in a focus of runaway neural activity within the temporal lobe and spread over the general cortex within seconds to trigger a secondarily generalized tonic–clonic seizure (Figure CP 34.3.1) as mentioned in Chapter 30. Types of temporal lobe auras include well-formed visual or auditory hallucinations (scenes, sound sequences), a sense of familiarity with the surrounding scene (’déjà vu’), a sense of strangeness (‘jamais vu’) or a sense of fear. Attacks originating in the uncus are ushered in by unpleasant olfactory or gustatory auras. Bizzare psychic auras can occur, where the patient has an ‘out of body experience’ in the form of a sensation of floating in the air and looking down at themselves and any others present.
Emerson RG, Pedley TA. EEG and evoked potentials. In: Bradley WG, Daroff RB, Finichel GM, Jankovic J, editors. Neurology in clinical practice: principles of diagnosis and management. Philadelphia: Elsevier; 2004:465-490.
Bernasconi N, Bernasconi A, Caramanos Z, et al. Mesial temporal damage in temporal lobe epilepsy: a volumetric MRI study of the hippocampus, amygdala and parahippocampal region. Brain. 2003;126:462-469.
Jay V, Becker LE. Surgical pathology of epilepsy. Pediatr Pathol. 2003;14:731-750.
Kempermann G. The neurogenic reserve rehypothesis: what is adult hippocampal neurogenesis good for? Trends Neurosci. 2008;89:1169-1176.
Niedergerger E, Kuhlein H, Geissler G. Update on the neuropathology of neuropathic pain. Expert Rev proteomics. 2008;5:719-818.
Shin C. Mechanism of epilepsy. Ann Rev Med. 1994;45:379-389.
Willis WD, Westland KR. Pain system. In: Paxinos G, Mai JK, editors. The human nervous system. ed 2. Amsterdam: Elsevier; 2004:1125-1170.
Glossary
Clinical and experimental observations
Long-term potentiation (LTP) is uniquely powerful in the dentate gyrus and hippocampus. It is regarded as vital for preservation (consolidation) of memory traces. Under experimental conditions, LTP is most easily demonstrated in the perforant path–dentate granule cell connections and in the Schaffer collateral–CA1 connections. A strong, brief (milliseconds) stimulus to the perforant path or Schaffer collaterals induces the target cells to show long-lasting (hours) sensitivity to a fresh stimulus. LTP is associated with a cascade of biochemical events in the target neurons, following activation of appropriate glutamate receptors, as described in Chapter 8 in the context of pain sensitization. Repetitive stimuli may cause cAMP to increase its normal rate of activation of protein kinases involved in phosphorylation of proteins that regulate gene transcription. The outcome is increased production of proteins (including enzymes) required for transmitter synthesis, and of other proteins for construction of additional channels and synaptic cytoskeletons.
LTP is described as an associative phenomenon, because the required expulsion of the magnesium plug from the NMDA receptor (Figure 34.8) is facilitated when the powerful depolarizing stimulus is coupled with a weaker stimulus to the depolarized neuron from another source. Norepinephrine and dopamine are suitable associative candidates, one or both being released during elevation of the attentional or motivational state at the appropriate time.
Cholinergic activity in the hippocampus