Chapter 7 Disorders of metabolism and homeostasis
Some metabolic disorders, congenital or acquired, are specific abnormalities of metabolic pathways, often having considerable clinical effects. Congenital metabolic disorders usually result from inherited enzyme deficiencies.
Other metabolic disorders are characterised by perturbations of the body’s homeostatic mechanisms maintaining the integrity of fluids and tissues. These conditions are almost always acquired and their effects can be diverse.
INBORN ERRORS OF METABOLISM
The concept of inborn errors of metabolism was formulated by Sir Archibald Garrod in 1908 as a result of his studies on a condition called alkaptonuria, a rare inherited deficiency of homogentisic acid oxidase.
Inborn (usually inherited) errors of metabolism are important causes of illness presenting in infancy. Some require prompt treatment to avoid serious complications. Others defy treatment. All deserve accurate diagnosis so that parents can be counselled about the inherited risk to further pregnancies. Inborn metabolic errors are potentially chronic problems, because the primary abnormality is innate rather than due to any external cause that could be eliminated by treatment.
Inborn errors of metabolism are single-gene defects resulting in the absence or deficiency of an enzyme or the synthesis of a defective protein. Single-gene defects occur in about 1% of all births, but the diseases caused by them show geographic variations in incidence; this is exemplified by the high incidence of thalassaemias—due to defects in haemoglobin synthesis (Ch. 23)—in Mediterranean regions. These variations reflect the prevalence of specific abnormal genes in different populations.
Inborn errors of metabolism have four possible consequences:
Accumulation of an intermediate metabolite may have toxic or hormonal effects. However, in some conditions the intermediate metabolite accumulates within the cells in which it has been synthesised, causing them to enlarge and compromising their function or that of neighbouring cells; these conditions are referred to as storage disorders (e.g. Gaucher’s disease). Other inborn metabolic errors lead to the production of a protein with defective function; for example, the substitution of just a single amino acid in a large protein can have considerable adverse effects (e.g. haemoglobinopathies).
The genetic basis of the inheritance of these disorders is discussed in Chapter 3.
Inherited metabolic disorders may be classified according to the principal biochemical defect (e.g. amino acid disorder) or the consequence (e.g. storage disorder).
Disorders of carbohydrate metabolism
The commonest disorder of carbohydrate metabolism with an inherited component in its aetiology is diabetes mellitus (see p. 128). Much less common, but with an autosomal recessive pattern of inheritance and often presenting at an early age, are:
Disorders of amino acid metabolism
Several inherited disorders of amino acid metabolism involve defects of enzymes in the phenylalanine/tyrosine pathway (Fig. 7.1).
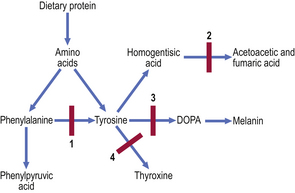
Fig. 7.1 Inborn errors of metabolism in the phenylalanine/tyrosine pathway. 1. Phenylketonuria. Lack of phenylalanine hydroxylase blocks conversion of phenylalanine to tyrosine; phenylalanine and phenylpyruvic acid appear in the urine. 2. Alkaptonuria. Lack of homogentisic acid oxidase causes accumulation of homogentisic acid. 3. Albinism. Lack of the enzyme tyrosinase prevents conversion of tyrosine via DOPA to melanin. 4. Familial hypothyroidism. Deficiency of any one of several enzymes impairs iodination of tyrosine in the formation of thyroid hormone.
Phenylketonuria
This autosomal recessive disorder affects approximately 1 in 10000 infants. It is due to a deficiency of phenylalanine hydroxylase, an enzyme responsible for the conversion of phenylalanine to tyrosine (Fig. 7.1).
In the UK and many other countries the clinical effects of phenylketonuria are now seen only very rarely, because it is detected by screening all newborn infants and treated promptly. Testing is done by analysing a drop of blood, dried on to filter paper, for phenylalanine (Guthrie test). If phenylketonuria is not tested for in this way and the affected infant’s diet contains usual amounts of phenylalanine, then the disorder manifests itself with skin and hair depigmentation, fits and mental retardation. Treatment involves a low phenylalanine diet until the child is at least 8 years old. When affected females themselves become pregnant, the special diet must be resumed to avoid the toxic metabolites damaging the developing fetus.
Alkaptonuria
This rare autosomal recessive deficiency of homogentisic acid oxidase (Fig. 7.1) is a good example of an inborn metabolic error that does not produce serious effects until adult life. The condition is sometimes recognised from the observation that the patient’s urine darkens on standing; the sweat may also be black! Homogentisic acid accumulates in connective tissues, principally cartilage, where the darkening is called ochronosis. This accumulation causes joint damage. The underlying condition cannot be cured; treatment is symptomatic only.
Homocystinuria
Like most inherited disorders of metabolism, homocystinuria is an autosomal recessive disorder. There is a deficiency of cystathionine synthase, an enzyme required for the conversion of homocystine via homocysteine to cystathionine. Homocysteine and methionine, its precursor, accumulate in the blood. Homocystine also accumulates, interfering with the cross-linking of collagen and elastic fibres. The ultimate effect resembles Marfan’s syndrome (see p. 128), but also with mental retardation and fits.
Storage disorders
Inborn metabolic defects result in storage disorders if a deficiency of an enzyme, usually lysosomal, prevents the normal conversion of a macromolecule (e.g. glycogen or gangliosides) into its smaller subunits (e.g. glucose or fatty acids). The macromolecule accumulates within the cells that normally harbour it, swelling their cytoplasm (Fig. 7.2) and causing organ enlargement and deformities. This situation is harmful to the patient because the swelling of cells often impairs their function, or that of their immediate neighbours due to pressure effects, and because of conditions resulting from deficiency of the smaller subunits (e.g. hypoglycaemia in the case of glycogen storage disorders).
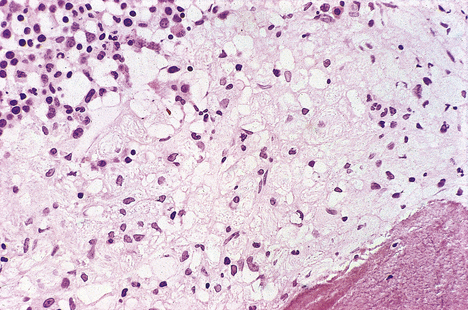
Fig. 7.2 Bone marrow biopsy revealing Gaucher’s disease. Pale foamy macrophages distended with gangliosides have displaced much of the haemopoietic tissue (top left), thereby causing anaemia.
Major categories of these autosomal recessive disorders (Table 7.1) are:
Table 7.1 Examples of inborn errors of metabolism resulting in storage disorders
Type of disease/examples | Deficiency | Consequences |
---|---|---|
Glycogenoses | Debranching enzyme |
McCardle’s syndromeMuscle phosphorylase von Gierke’s diseaseGlucose-6-phosphate dehydrogenase Pompe’s diseaseAcid maltase MucopolysaccharidosesLysosomal hydrolase
Hurler’s syndromeAlpha-l-iduronidase Hunter’s syndromeIduronate sulphate sulphatase SphingolipidosesLysosomal enzyme
Gaucher’s diseaseGlucocerebrosidase Niemann–Pick diseaseSphingomyelinase Tay–Sachs diseaseHexosaminidase A
Disorders of cell membrane transport
Inborn metabolic errors can lead to impairment of the specific transport of substances across cell membranes. Examples include:
Channelopathies
A channelopathy is a disease caused by the dysfunction of a specific ion channel in cell membranes. The ion channel dysfunction may result from:
Cystic fibrosis
Cystic fibrosis, a channelopathy, is the commonest serious inherited metabolic disorder in the UK; it is much commoner in Caucasians than in other races. The autosomal recessive abnormal gene is carried by approximately 1 in 20 Caucasians and the condition affects approximately 1 in 2000 births. The defective gene, in which numerous mutations have been identified, is on chromosome 7 and ultimately results in abnormal water and electrolyte transport across cell membranes.
Cystic fibrosis transmembrane conductance regulator (CFTR)
The commonest abnormality (ΔF508) in the CFTR gene is a deletion resulting in a missing phenylalanine molecule. The defective CFTR is unresponsive to cyclic AMP control, so transport of chloride ions and water across epithelial cell membranes becomes impaired (Fig. 7.3).
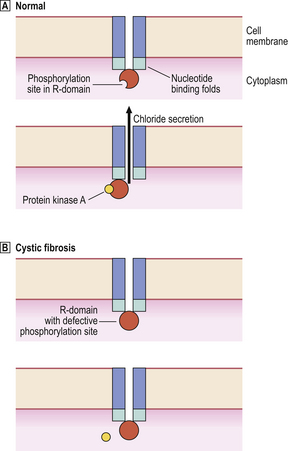
Fig. 7.3 Defective chloride secretion in cystic fibrosis. The normal CFTR is a transmembrane molecule with intracytoplasmic nucleotide binding folds and a phosphorylation site on the R-domain. In normal cells, interaction of the R-domain with protein kinase A results in opening of the channel and chloride secretion.
In cystic fibrosis, a common defect prevents phosphorylation of the R-domain with the result that chloride secretion is impaired.
Clinicopathological features
Cystic fibrosis is characterised by mucous secretions of abnormally high viscosity. The abnormal mucus plugs exocrine ducts, causing parenchymal damage to the affected organs. The clinical manifestations are:
Porphyrias
The porphyrias, transmitted as autosomal dominant disorders, are due to defective synthesis of haem, an iron–porphyrin complex, the oxygen-carrying moiety of haemoglobin. Haem is synthesised from 5-aminolaevulinic acid. The different types of porphyrin accumulate due to inherited defects in this synthetic pathway (Fig. 7.4).
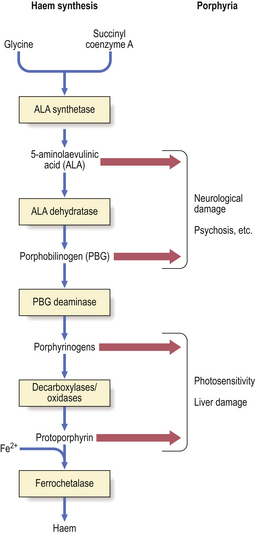
Fig. 7.4 Porphyrias. Enzyme deficiencies in the pathway of synthesis of haem from glycine and succinyl coenzyme A through 5-aminolaevulinic acid result in the accumulation of toxic intermediate metabolites. Removal of product inhibition due to deficient synthesis of haem enhances the formation of intermediate metabolites. Accumulation of 5-aminolaevulinic acid or porphobilinogen tends to be associated with neurological damage and psychiatric symptoms. Accumulation of porphyrinogens, of which there are several types (uro-, copro-, proto-), tends to be associated with photosensitivity.
Clinicopathological features
Accumulation of porphyrins can cause clinical syndromes characterised by:
The pain and psychiatric disturbances are episodic. During the acute attacks, the patient’s urine contains excess 5-aminolaevulinic acid and porphobilinogen. Consequently, the urine may gradually become dark red, brown or even purple (‘porphyria’ is derived from the Greek word ‘porphura’ meaning purple pigment) on exposure to sunlight.
Acute attacks of porphyria can be precipitated by some drugs, alcohol and hormonal changes (e.g. during the menstrual cycle). The most frequently incriminated drugs include barbiturates, sulphonamides, oral contraceptives and anticonvulsants; these should therefore be avoided.
The skin lesions are characterised by severe blistering, exacerbated by light exposure, and subsequent scarring. This photosensitivity is a distressing feature, but it has led to the beneficial use of injected porphyrins in the treatment of tumours by phototherapy with laser light.
Disorders of connective tissue metabolism
Most inherited disorders of connective tissue metabolism affect collagen or elastic tissue. Examples include:
Osteogenesis imperfecta
Osteogenesis imperfecta is a group of disorders in which there is an inborn error of type I collagen synthesis (Ch. 25). Type I collagen is most abundant in bone, so the principal manifestation is skeletal weakness resulting in deformities and a susceptibility to fractures; the other names for this condition are ‘fragilitas ossium’ and ‘brittle bone disease’. The teeth are also affected and the sclerae of the eyes are abnormally thin, causing them to appear blue. It occurs in dominantly and recessively inherited forms with varying degrees of severity.
Marfan’s syndrome
Marfan’s syndrome is a combination of unusually tall stature, long arm span, dislocation of the lenses of the eyes, aortic and mitral valve incompetence, and weakness of the aortic media predisposing to dissecting aneurysms (Ch. 13). The condition results from a defect in the FBN1 gene encoding for fibrillin, a glycoprotein essential for the formation and integrity of elastic fibres.
ACQUIRED METABOLIC DISORDERS
Many diseases result in secondary metabolic abnormalities. In others the metabolic disturbance is the primary event. For example, renal diseases almost always result in metabolic changes that reflect the kidneys’ importance in water and electrolyte homeostasis. In contrast, a disease like gout is often due to a primary metabolic disorder that may secondarily damage the kidneys. This section deals with metabolic abnormalities as both consequences and causes of disease. Acquired metabolic disorders frequently cause systemic problems affecting many organs: for example, diabetes mellitus is associated with microvascular damage in the retinas, nerves, kidneys and other organs; electrolyte imbalance compromises the function of cells in all tissues.
Two of the disorders discussed in this section—diabetes mellitus and gout—are categorised as ‘acquired’ largely because they occur most commonly in adults, but both have a significant genetic component in their aetiology.
Diabetes mellitus
Diabetes mellitus is a group of diseases characterised by impaired glucose homeostasis resulting from a relative or absolute insufficiency of insulin. Insulin insufficiency causes hyperglycaemia and glycosuria. Diabetes is covered in Chapter 17, but a brief account is relevant here.
The aetiology is multifactorial; although the disorder is acquired, there is a significant genetic predisposition. Diabetes mellitus is subclassified into primary and secondary types. Primary diabetes is much more common than diabetes secondary to other diseases.
Primary diabetes mellitus
Primary diabetes mellitus (DM) is subdivided into:
Juvenile-onset diabetes, usually appearing before the age of 20 years, is almost always of IDDM type. There is an inherited predisposition associated with human leukocyte antigens (HLA) DR3 and DR4. The initiating event may be a viral infection of the insulin-producing beta-cells of the islets of Langerhans, precipitating their immune destruction.
Diabetes mellitus developing in adults (over 25 years of age) is most likely to be NIDDM type. It is associated with an acquired resistance to insulin. There is no HLA association, but there is a familial tendency to develop the disease. The affected patients are often, but not always, obese.
Complications of diabetes mellitus
Good control of blood sugar levels reduces the risk of complications. Nevertheless, many diabetics develop complications of their disease. These are covered in more detail in the relevant chapters, but a summary is given here:
To this list should be added hypoglycaemia, which is a frequent and troublesome complication of insulin therapy in IDDM.
There are two possible biochemical explanations for the tissue damage that results from long-term diabetes mellitus:
Gout
Gout is a common disorder resulting from high blood uric acid levels. Uric acid is a breakdown product of the body’s purine (nucleic acid) metabolism (Fig. 7.5), but a small proportion comes from the diet. Most uric acid is excreted by the kidneys. In the blood, most uric acid is in the form of monosodium urate. In patients with gout, the monosodium urate concentration may be very high, forming a supersaturated solution, thus risking urate crystal deposition in tissues causing:
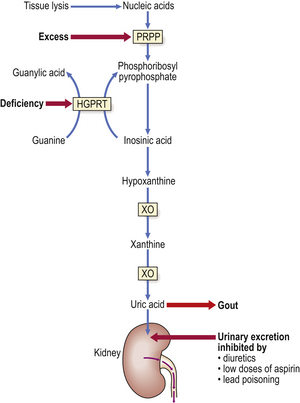
Fig. 7.5 Pathogenesis of gout. The metabolic pathway shows the synthesis of uric acid from nucleic acids. Primary gout can arise from an inherited (X-linked) deficiency of hypoxanthine guanine phosphoribosyl transferase (HGPRT) or excessive activity of 5-phosphoribosyl-1-pyrophosphate (PRPP). Secondary gout results either from increased tissue lysis (e.g. due to tumour chemotherapy) liberating excess nucleic acids or from inhibition of the urinary excretion of uric acid. Xanthine oxidase (XO) is inhibited by allopurinol, an effective long-term remedy for gout.
Gout occurs more commonly in men than in women, and is rare before puberty. A rare form of gout in children—Lesch–Nyhan syndrome—is due to absence of the enzyme HGPRT (hypoxanthine guanine phosphoribosyl transferase) (Fig. 7.5) and is associated with mental deficiency and a bizarre tendency to self-mutilation.
Aetiology
Like diabetes mellitus, the aetiology of gout is multifactorial. There is a genetic component, but the role of other factors justifies the inclusion of gout under the heading of acquired disorders. Aetiological factors include:
Some of these factors may, of course, be interdependent. Accordingly, gout can be subdivided into primary gout, due to some genetic abnormality of purine metabolism, or secondary gout, due to increased liberation of nucleic acids from necrotic tissue or decreased urinary excretion of uric acid.
Clinicopathological features
The clinical features of gout are due to urate crystal deposition in various tissues (Fig. 7.6). In joints, a painful acute arthritis results from phagocytosis of the crystals by neutrophil polymorphs, in turn causing release of lysosomal enzymes along with the indigestible crystals, thus accelerating and perpetuating a cyclical inflammatory reaction. The first metatarsophalangeal joint is typically affected.
Water homeostasis
Water and electrolyte homeostasis is tightly controlled by various hormones, including antidiuretic hormone (ADH), aldosterone and atrial natriuretic peptide, acting upon selective reabsorption in the renal tubules (Ch. 21). The process is influenced by the dietary intake of water and electrolytes (in food or drinking in response to thirst or social purposes) and the adjustments necessary to cope with disease or adverse environmental conditions.
Many diseases result in problems of water and electrolyte homeostasis. Disturbances can also occur in post-operative patients receiving fluids and nutrition parenterally. Fortunately, any changes are fairly easy to monitor and control by making adjustments to the fluid and electrolyte intake.
Water is constantly lost from the body—in urine, in faeces, in exhaled gas from the lungs, and from the skin. The replenishment of body water is controlled by a combination of the satisfaction of the sensation of thirst and the regulation of the renal tubular reabsorption of water mediated by ADH.
Water excess
Excessive body water may occur in patients with extensive oedema or if there is inappropriate production of ADH (e.g. as occurs with small-cell lung carcinoma) or if the body sodium concentration increases due to excessive tubular reabsorption (for example, due to an aldosterone-secreting tumour of the adrenal cortex). Water overload can be caused iatrogenically by excessive parenteral infusion of fluids in patients with impaired renal function; this should be avoided by carefully monitoring fluid input and output.
Dehydration
Dehydration results from either excessive water loss or inadequate intake or a combination of both. Inadequate water intake is a common problem in regions of the world affected by drought and famine.
Excessive water loss can be due to:
Dehydration is recognised clinically by a dry mouth, inelastic skin and, in extreme cases, sunken eyes. The blood haematocrit (proportion of the blood volume occupied by cells) will be elevated, causing an increase in whole blood viscosity. This results in a sluggish circulation and consequent impairment of the function of many organs.
The plasma sodium and urea concentrations are typically elevated, reflecting haemoconcentration and impaired renal function.
Oedema and serous effusions