Nutrition
A Carbohydrate (CHO) metabolism
1. CHO usually makes up 40% to 45% of the total food intake but may reach 60%.
2. Amylases and disaccharidases hydrolyze complex starches and sugars to monosaccharides before they are absorbed in the small intestine.
a. One half of the ingested CHO is digested to glucose, and the rest are digested mainly to fructose and galactose.
b. Most of the glucose circulates as blood sugar and is taken up by the body cells and metabolized for energy.
c. Fructose, galactose, and some glucose are converted to glycogen, some in the liver and the rest in muscle.
3. Glucose is also manufactured from amino acids and other products of intermediary metabolism by a process called gluconeogenesis, which protects the glycogen reserves.
a. In the fasting state, liver glycogen is stimulated by catecholamine and glucagon to undergo glycogenolysis and form blood glucose.
b. Glucose then undergoes glycolysis to pyruvate.
(1) Pyruvate may undergo three basic metabolic processes:
(a) It can move into the mitochondria and be converted to acetyl coenzyme A (acetyl-CoA) for oxidation in the citric acid cycle.
(b) Diversion of pyruvate for oxidation to CO2 and H2O in the citric acid cycle and the respiratory chain is known as aerobic glycolysis.
(2) When O2 is not present for aerobic glycolysis, pyruvate is reduced to lactate.
(3) Anaerobic glycolysis is a rapid method for energy production.
(a) It does not need large quantities of O2.
(b) However, only limited energy production occurs via this mechanism.
(c) Pyruvate can also be carboxylated to oxaloacetate in the mitochondria by pyruvate carboxylase.
(d) Oxaloacetate can then form glucose in a process that is the reverse of glycolysis, known as gluconeogenesis.
1. Lipid is the main energy substrate in the body.
a. Lipid provides approximately 90% of the total body caloric reserve.
b. Most body fat exists as fatty acids that are esterified with glycerol to form tri-glycerides.
2. Dietary lipid is hydrolyzed in the intestinal tract, absorbed, and then resynthesized to triglycerides.
a. These are transported from the jejunal wall as lipid-protein complexes called chylomicrons.
b. Chylomicrons enter the blood via the intestinal lymphatics (lacteal) and thoracic duct.
c. Short- and medium-chain free fatty acids enter directly into the portal blood without conversion into chylomicrons.
3. Lipoproteins may be modified in the liver before going to adipose tissue or may go there directly.
a. In adipose tissue, lipoproteins are hydrolyzed, releasing fatty acids.
b. The fatty acids are reesterified and stored as triglycerides.
4. Triglycerides may also be formed from CHOs by lipogenesis.
a. When CHO intake is decreased, triglycerides are mobilized to glycerol and fatty acids.
b. The glycerol is partly converted to glucose by gluconeogenesis.
5. The free fatty acids are oxidized to produce acetyl-CoA, which is converted to energy via the Krebs cycle.
1. Protein normally comprises approximately 20% of the lean body mass or approximately 15% of the total body weight.
2. An intake of approximately 0.8 to 1 g/kg of body weight is needed each day in a normal individual.
3. If CHOs and lipids meet energy demands, protein is used entirely for:
4. Specific enzymes in the intestinal tract hydrolyze ingested protein to peptides and ultimately to amino acids.
5. The body cannot synthesize essential amino acids:
6. Amino acids not used anabolically for protein synthesis undergo catabolism (e.g., they may be transaminated or deaminated). After deamination the residue may be converted by way of either:
7. The nitrogen released is excreted in the urine as urea.
8. Branched chain amino acids (e.g., valine, leucine, and isoleucine) are used by muscle for metabolism.
d. Branched chain amino acids given intravenously can be used as a source of energy for muscles and can preserve lean body mass.
9. Alanine is the major gluconeogenic amino acid.
D Summary of energy metabolism
1. Glucose is the principal energy source of anaerobic metabolism:
2. The Krebs cycle is the main source of aerobic metabolism.
a. It requires some glucose to prime it.
b. Total energy needs cannot be supplied by fatty acid metabolism.
4. Triglycerides can be manufactured from excess CHO, lipid, and protein intake.
II Determination of Caloric and Protein Needs
1. Energy is commonly measured in units of calories or joules.
B Energy used by the body can be measured by direct or indirect calorimetry.
1. Direct calorimetry is based on the postulate that all energy expended in the body eventually becomes heat.
a. In a resting person, the amount of energy expended over a given time, or metabolic rate, can be determined by measuring the heat liberated.
2. Indirect calorimetry is based on the postulate that >95% of the energy expended by a person is derived from the reaction of oxygen with food (oxidation).
1. The BMR is defined as the rate of energy expenditure under a given set of basal conditions.
2. The BMR gives an estimate of energy expended when no extra demands are placed on a patient and would include such functions as:
D Resting energy expenditure (REE)
1. If energy expenditure is measured after eating, energy expenditure is increased.
2. The REE is defined as the BMR plus the component of SDA of food.
3. The Harris-Benedict equation will give an estimation of REE:
< ?xml:namespace prefix = "mml" />


where A = age in years, H = height in centimeters, and W = weight in kilograms.
1. The final factor that must be included to determine the total energy expenditure (TEE) is exercise or physical work performance and degree of stress.
2. TEE = (REE × Activity factor) × Stress factor (Table 24-1)
TABLE 24-1
Factors Indicating Increases in Basal Metabolic Rate Under Certain Physiologic Conditions
Condition | Factor |
Confined to bed | 1.2 |
Normal activity | 1.3 |
Minor operation | 1.2 |
Skeletal trauma | 1.35 |
Major sepsis | 1.6 |
Severe thermal burn | 2.1 |
3. The BMR has been found to increase under certain conditions (see Table 24-1).
1. The normal man engaging in an average amount of exercise requires 0.8 to 1.0 g of protein/kg body weight/day.
2. In the moderately stressed individual (e.g., infection and major surgery) protein requirement is 1.5 to 2.0 g/kg body weight/day.
3. In the severely stressed person (e.g., burns and major trauma) protein requirement is 2.0 to 4.0 g/kg body weight/day.
4. A calorie-to-nitrogen ratio of 150:1 is adequate for most catabolic patients. Higher ratios are used for those in severe stress.
5. Daily protein requirements are:

III Vitamin Requirements and Deficiency States
A General functions of vitamins
1. Vitamins generally participate in metabolism in the interconversion and degradation of protein and amino acids.
2. They also participate in the extraction of energy from CHO and fat sources.
a. Function in biochemical systems
(1) Cellular hydrogen transport
(2) Component of coenzymes nicotinamide adenine dinucleotide (NAD) and nicotinamide adenine dinucleotide phosphate (NADP)
7. Vitamin B12 (cyanocobalamin)
a. Function in biomedical systems
b. Requires gastric intrinsic factor and distal ileum for absorption
IV Interrelationship Between Pulmonary Disease and Nutritional Status
A Pulmonary function and nutritional status are closely interrelated.
1. Malnutrition increases the risk of acute and chronic respiratory failure (CRF).
2. Inattention to nutritional requirements in patients with CRF results in increased morbidity and mortality.
B Providing nutrients essential for respiratory function enhances pulmonary function.
1. Calories and protein should be provided in proportions to place the least stress on respiratory capacity.
2. The amount of O2 consumed and the amount of CO2 produced are different for each major nutrient.
a. For each calorie of CHO metabolized, 0.20 L of O2 is consumed and 0.20 L of CO2 is produced.
b. For each calorie of protein metabolized, 0.24 L of O2 is consumed and 0.19 L of CO2 is produced.
c. For each calorie of fat metabolized, 0.22 L of O2 is consumed and 0.15 L of CO2 is produced.
3. The ratio of CO2 produced to O2 consumed is called the respiratory quotient (RQ).
4. A diet with a decreased amount of CHO and a proportionally increased amount of fat (50%) should be provided to patients with hypercapnia.
C Protein requirements and ventilatory drive
1. Protein intake should match requirement.
2. Low protein intake and high CHO intake will:
a. Decrease elimination of theophylline.
b. Require adjustment in dosage to avoid theophylline toxicity.
3. Long-term use of steroids promotes:
4. Excessive protein intake can be detrimental to a person who is unable to increase minute ventilation.
5. High-protein diets stimulate ventilatory drive and minute ventilation.
6. High-protein diets may increase the clearance of theophylline.
7. Adequate protein should be provided to allow for anabolism.
8. Nitrogen balance is a useful means to determine the adequacy of protein intake (see Section VII, Nutritional Assessment).
V Weight Loss and Pulmonary Disease
A Decreased body weight is a complication of chronic obstructive pulmonary disease (COPD).
1. The incidence varies between 25% and 65% of patients with COPD.
2. Body weight correlates with the percent of predicted diffusion capacity and forced expiratory volume in 1 second.
3. Sustained weight loss is associated with a poor prognosis.
B Factors that result in decreased calorie intake
1. Twenty percent to 25% of patients with COPD have peptic ulcers, and gastrointestinal discomfort may cause these patients to shun food.
2. Bronchodilators, both sympathomimetic and theophylline derivatives, are gastric irritants.
3. Chronic sputum production may alter the desire for and taste of food.
4. A full stomach restricts descent of the diaphragm and makes breathing difficult after large meals.
5. Shortness of breath may hamper the patient’s ability to prepare meals or to eat with comfort.
C Factors that increase caloric expenditure
1. Infections common in acute respiratory illness increase metabolic demand.
2. The respiratory disease itself is capable of increasing energy expenditure.
3. The elevated energy requirement may be related to the increased work of breathing in patients with respiratory disease.
a. With diffuse bronchial obstruction, the mechanical work necessary to overcome nonelastic resistance increases.
b. The respiratory muscles require oxygen to perform this mechanical work.
4. The caloric cost of breathing increases in patients with pulmonary disease.
A Respiratory muscles are catabolized to meet the body’s energy needs in those with malnutrition.
1. Autopsy studies provide evidence of decreased weight of the diaphragm muscle in patients with emphysema.
2. Malnutrition decreases the degree to which the diaphragm can contract.
B Respiratory muscle function is decreased in those with malnutrition.
A The systematic evaluation of a patient’s current state of nutrition using physical and biochemical means
B Nutrition history parameters
C Anthropometric parameters (somatic compartment) (Table 24-2)
TABLE 24-2
Triceps Skinfold (mm) | Arm Muscle Circumference (cm) | |
Male | 12.5 | 25.3 |
Female | 16.5 | 23.2 |
% deficit in anthropometric measurements = ![]() |
2. Triceps skinfold (TSF; see Table 24-2)
3. Arm muscle circumference (AMC; see Table 24-2)
4. Not as reliable as originally thought; many subpopulations require additional study.
D Laboratory parameters (visceral compartment)
TABLE 24-3
Normal | Mild Deficit | Moderate Deficit | Severe Deficit | |
Albumin (g/dl) | 3.5-5.0 | <3.5 | <3.0 | <2.5 |
Transferrin (mg%) | >200 | <200 | <180 | <160 |
Total lymphocyte count (number/mm3) | 2000-4000 | <1800 | <1500 | <900 |
a. Normal serum albumin is 3.5 to 5.0 g/dl.
(1) Normal serum albumin level is the equilibrium point between production, distribution, and degradation.
b. Count of 2.8 g% is the cutoff point for albumin oncotic pressure; <2.8 g%, edema will usually be present.
c. Hypoalbuminemia is more commonly a marker for systemic inflammatory response and rarely present in cases of isolated calorie malnutrition.
a. Uses gas analyzers to determine oxygen consumption (o2) and carbon dioxide production (
co2).
b. Carbon dioxide production (co2) divided by oxygen consumption (
o2) is RQ (e.g., RQ =
co2/
o2); normal value = 0.8 to 1.0.
c. Can be done at the bedside.
(1) Needs a well-trained operator for consistent results.
(2) Frequent calibration required for gas analyzers.
(3) Incompatible with newer generation ventilators that incorporate a continuous bias flow.
d. Indirect calorimeters require a constant inspiratory and expiratory volume for determination of o2 and
co2.
e. Requires 30 to 45 minutes of an equilibrium state.
f. Controversial whether indirect calorimetry is needed with the change in nutritional management from total parenteral nutrition (TPN) to enteral tube feeding (ETF).
(1) TPN is characterized by “hyperalimentation” and excessive nutritional support.
(a) Indirect calorimetry has a role in identifying individual caloric requirements and minimizing overfeeding.
(b) Passive method of providing nutrients.
(c) Risk of aspiration is lower with patients receiving TPN because of less content within the stomach.
(2) ETF is a more physiologic route for providing nutritional support.
a. Adipose tissue has a higher water content than does lean muscle mass (LMM).
b. Using electrodes spaced over the body, usually hand and ankle, a small current is passed.
4. End-tidal carbon dioxide monitoring
a. CO2 is a by-product of metabolism.
b. Sensor attached to the airway uses mainstream infrared absorption of expired gases to obtain CO2 concentration.
(1) When paired with a pneumotachometer, it is possible to integrate airway flow and end-tidal CO2 measurement on a breath-by-breath basis (Figure 24-1, A).
(a) Allows for determination of co2.
i. co2 is extrapolated from the graphical plot of expired gas; at the intersection point where the slope of end-tidal CO2 is measured, and a vertical line drawn at the phase of the breath where CO2 is beginning to be expired.
ii. When this is matched with airway flow measurements, co2 is determined as the area under this curve (see Figure 24-1, B).
iii. Using this technique and a partial CO2 rebreathing method, the cardiac output can also be determined (see Figure 24-1, C).
c. REE can be determined using the Harris-Benedict equation.
d. This technique assumes either low or no intrapulmonary shunting.
e. Easy to use and can be performed at the bedside.
f. Using a modified Fick equation, cardiac output can also be determined.
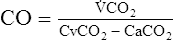

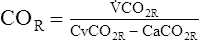

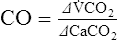
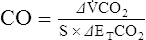
Cvco2 = Mixed venous CO2 content (equivalent to ETco2)
Caco2 = Arterial CO2 content (equivalent to ETco2)
CO, Cvco2, and Vd/Vt are constant during the measurement period.
A shunt correction is added to the final equation based on FIO2 and hemoglobin saturation.
Calculate the cardiac output using the following data:
Normal Breathing | Partial CO2 Rebreathing |
![]() |
![]() |
ETCO2N = 36 mm Hg | ETCO2R = 43 mm Hg |
SpO2N = 97% | SpO2R = 97% |
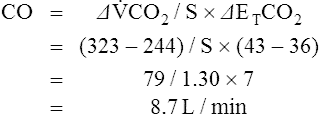
Note: S is the slope of ΔETco2 by the device.
Calculate the REE for a patient with a co2 of 279 ml/min.
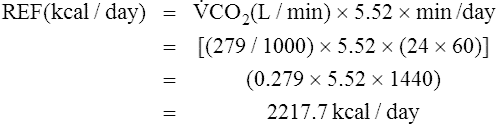
where 5.52 is the standard number of kcal consumed to produce 1 L of CO2.
5. Total iron-binding capacity or transferrin
a. Values of ≤200 mg% correlate with malnutrition.
b. Protein secreted by the liver
c. Protein that transports iron
d. Secretion declines in protein-depleted states.
e. Protein is manufactured by the endoplasmic reticulum in liver cells, which depend on adequacy of amino acid, whether from diet, muscle, gut, or cutaneous tissue.
f. First protein to increase as a result of nutritional repair.
g. Not useful to assess malnutrition because of the metabolic half-life of transferrin.
6. Total lymphocyte count (TLC; see Table 24-3)
a. The nitrogen balance is calculated to assess whether protein is being depleted and to assess the estimated degree of hypermetabolism.
b. Twenty-four-hour UUN is ordered.
c. Nitrogen balance = N in − N out.
d. In the healthy individual, nitrogen intake and nitrogen excretion are equal (approximately 11 g/day) and in balance.
e. Positive nitrogen balance more than +2 indicates net tissue growth.
f. Negative nitrogen balance more than -2 indicates net tissue breakdown.
8. Creatinine height index (CHI)
a. Creatinine is given off by muscle at a constant rate.
b. An accurate indicator of lean body mass.
c. Uses 24-hour urine test for creatinine.
d. Creatinine excreted in 24 hours is compared with normal creatinine-to-height ratios.
1. When nutrient intake is insufficient to meet requirements, malnutrition develops.
2. Kwashiorkor (protein malnutrition)
a. The patient who has developed kwashiorkor is one whose caloric intake was adequate or more than adequate in CHO and fat but contained little, if any, protein.
b. The patient will have adequate somatic stores.
A Basic goals of dietary intervention for patients with chronic pulmonary disease:
1. Prevent nutritional depletion.
2. Ensure that the mix of nutrients can be handled easily by the impaired respiratory system.
B General principles of tube feeding the patient with chronic respiratory disease and/or who is mechanically ventilated
1. Provide a caloric intake to meet caloric needs of nutritional maintenance.
2. Gradually increase the caloric intake beyond maintenance and monitor the respiratory effect when nutritional rehabilitation is the goal.
3. Avoid overfeeding of protein.
a. Provide enough protein so that nitrogen intake equals nitrogen output when maintenance is the goal.
b. Provide enough protein to achieve a positive nitrogen balance if repletion is the goal.
4. Restrict fluid and/or sodium as needed to lower pulmonary vascular pressure and decrease extravascular lung water.
5. Monitor serum phosphate levels to avoid hypophosphatemia.
a. Lean muscle contains approximately 100 mEq of phosphate/kg of wet tissue.
b. Phosphate deficiency occurs when the patient’s previous state of malnutrition is underestimated or if inadequate phosphate is provided.
c. Deficiencies can develop in <48 hours.
d. Hypophosphatemia can occur if increased requirements for phosphorus are not met.
6. Hypercapnic and ventilator-dependent patients should have less CHO and more fat to minimize the demand on the respiratory system to eliminate CO2.
a. They should have ≥50% fat calories and ≤30% CHO calories.
b. Sufficient CHO should be provided to prevent ketosis.
c. The caloric distribution of the feeding can be manipulated by adding fat (corn oil) to a commercial feeding.
d. Pulmocare, a commercial enteral product, contains >50% calories of fat.
A Nonhypercapnic patients: dietary recommendations
1. Make food preparation easier so fatigue does not result.
2. Rest just before eating so respiratory demand is low.
3. Eat in a quiet, relaxed atmosphere.
4. Emphasize the meals early in the day if fatigue becomes worse as the day continues.
5. Avoid foods that produce gas or bloating, which will cause distention and displacement of the diaphragm.
B Hypercapnic patients: dietary recommendations
1. Gradually increase caloric intake and monitor respiratory effect.
2. Substitute lower cholesterol fat for high CHO foods.