Chapter 163 Nuclear Replacement
Spinal surgeons contend with back pain on a daily basis. Back pain has acquired epidemic proportions in industrialized nations. It has a prevalence of 60% to 90% and is second only to the common cold as a reason for a physician visit.1,2 With the total costs associated with back pain ranging from 100 billion dollars to 200 billion dollars annually, back pain is a major health and socioeconomic burden.3 Most episodes of back pain are short in duration. However, many cases have a recurrent course, and further acute episodes affect 20% to 44% of patients in the working population within 1 year. The lifetime recurrence rate can be 85%.4
Lumbar disc degeneration is the most common cause of back pain. Disc degeneration generally results from reduced proteoglycan content in the nucleus and reduced nuclear hydration. Resulting biomechanical changes in the disc lead to loss of disc height and increasing biomechanical demand on the anulus with imbalance in the stress distribution across the disc space.5,6 When tension in the anulus is lost, ventral and dorsal instability of the motion segment can ensue. Increasing loads on the anulus can lead to anular tears with or without disc herniations. Continued loss of disc height can lead to osteophyte formation, facet arthrosis, and stiffness of the motion segment. Pain from disc degenerative disease (DDD) can occur at any stage of this degenerative cascade from early disc degeneration to instability and deformity.
Traditional treatment modalities for symptoms resulting from disc degeneration are focused on decompression with or without fusion. These treatment modalities do not attempt to halt the degenerative cascade and, in many instances, can lead to further progression of degeneration. Although short-term outcomes after lumbar discectomy have been shown to be superior compared with conservative care,7,8 long-term outcomes have been compromised by persistent back pain and a high risk of reoperations with a significant number of reherniations.9,10 Arthrodesis of the motion segment is still the “gold standard” for treatment of chronic disabling back pain of discogenic origin. However, it is difficult to predict the clinical response to arthrodesis. The outcome depends on multiple factors, such as the initial diagnosis, previous surgeries, prior fusion attempts, and the number of levels requiring fusion. Long-term studies have shown a fusion rate of 87% and clinical success rate of 76% for DDD.11,12 There are several disadvantages inherent to arthrodesis. Most importantly, arthrodesis can change the biomechanical loading of the adjacent segment, leading to accelerated degeneration.
Treatment of discogenic pain is fraught with difficulty and unpredictable outcomes. Additionally, in patients with early disc degeneration with minimal or no loss of disc height, there are no reliable methods for the diagnosis of the origin of discogenic back pain. Discography has been used to aid in the diagnosis; however, its reliability has been questioned.13–17
History of Nucleus Replacement
Early attempts at nuclear replacements consisted of injecting polymethylmethacrylate18,19 or self-curing silicone19 into the disc space after a nucleotomy. Clinical results were found to be similar to discectomy controls. Flow of the injected material and curing of the injected liquids were difficult to control. These variables led to abandonment of these procedures, but these initial attempts formed the theoretical basis of present-day preformed nuclear replacement systems.
Fernstrom20 was the first to use stainless steel balls as nuclear replacement by inserting them into the central cavity created after a discectomy. He implanted these balls in 125 patients at 191 lumbar levels. In 1966, Fernstrom20 reported that the outcome after this kind of nuclear replacement was better than discectomy alone and was similar to fusion. This procedure was largely abandoned owing to the subsidence of steel balls into the vertebral end plates. In 1995, McKenzie21 reported long-term outcomes of the Fernstrom ball. At 17 years of follow-up of 67 patients implanted with Fernstrom balls, he reported an 83% success rate in patients with one or more disc protrusions and a 75% success rate in patients with DDD. Although the Fernstrom ball never found mainstream acceptance as a nuclear replacement device, it did suggest that nuclear replacement can be a viable alternative to either a stand-alone discectomy or a fusion.
In 1981, Edeland22 suggested a design for an elastodynamic disc replacement device that would mimic the native disc biomechanically and biologically. Such a device would allow influx or efflux of water molecules in response to the load applied to it. Finding an elastodynamic material remained the most limiting factor. Attempts at finding such material in the 1980s and 1990s focused on either silicone polymers or a hydrogel nucleus composed of polyvinyl alcohol that was used to make soft contact lenses. Hou et al.23 designed a solid preformed silicone rubber horseshoe-shaped implant termed the lumbar intervertebral replacement prosthesis. Biomechanical cadaver studies and animal studies in monkeys showed restoration of the disc height and mechanical stability. Human studies with this implant have not been reported.
In 1991, Bao and Hingham patented a hydrogel nucleus made of polyvinyl alcohol that imbibed and extruded water and could replicate the biomechanical viscoelastic properties of the native nucleus. An animal study by Allen et al.24 showed no evidence of systemic or local toxicity but a high incidence of implant extrusion. Despite this setback, proven preclinical benefits with hydrogel acted as a springboard for other nucleus replacement technologies. With its first clinical use in 1996, the prosthetic disc nucleus (PDN) is the most well-known nucleus replacement device made of hydrogel.25 The device is made of hydrogel enclosed in a polyethylene terephthalate (PET) jacket to restrict expansion on hydration. Multiple design and material changes have followed after its first use (Fig. 163-1).
Current Nuclear Replacement Technology
HydraFlex (Raymedica, Bloomington, IN) is the latest version of the PDN; it is inserted in a dehydrated form that rehydrates on implantation (Fig. 163-2). The hydrogel component is a block copolymer of polyacrylamide-polyacrylonitrile (Hypan, Lipo Technologies, Vandalia, OH) formed by solution casting. The jacket is formed of a dense woven circular tube of PET, which is a thermoplastic polymer. The PET jacket is inelastic but flexible, which helps the hydrogel core to maintain its shape when subject to compressive forces. The hydrogel core absorbs water on implantation. Hypan80, which is currently being used, absorbs 80% of its weight by water, which makes it softer and flexible. HydraFlex takes approximately 32 hours to reach hydration equilibrium.
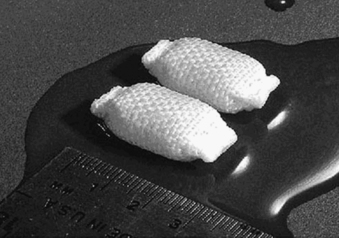
FIGURE 163-2 PDN replacement.
(From Berlemann U, Schwarzenbach O: An injectable nucleus replacement as an adjunct to microdiscectomy: 2 year follow-up in a pilot clinical study. Eur Spine J 18:1706–1712, 2009.)
DASCOR (Disc Dynamics, Eden Prairie, MN) is a two-part in situ curable polyurethane. It consists of a polyether polyurethane core encapsulated and adhered to a polycarbonate polyurethane balloon, which has cavity expansion and conforming capabilities. The device is fabricated by mixing a two-part, liquid, pre–polyether polyurethane reactive system and injecting a liquid mixture in a polycarbonate polyurethane balloon delivery catheter placed within the nucleus cavity (Fig. 163-3). A custom electromechanical injection system is used to apply a computer-controlled pressure profile designed to deliver the liquid polymer.26
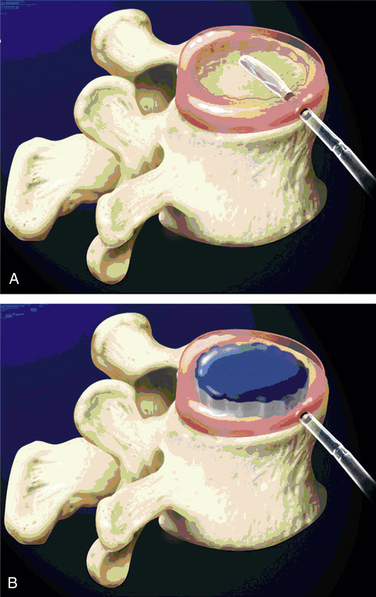
FIGURE 163-3 DASCOR nuclear replacement.
Implant placement (A) and deployment (B). (From Ahrens M, Tsantrizos A, Donkersloot P, et al: Nucleus replacement with the DASCOR disc arthroplasty device: interim two-year efficacy and safety results from two prospective, non-randomized multicenter European studies. Spine [Phila Pa 1976] 34:1376–1384, 2009.)
NuCore Injectable Disc Nucleus (Spine Wave, Shelton, CT) is an in situ curing protein polymer hydrogel that mimics the properties of the natural nucleus. The polymer chain is composed of silk and elastin components designed for elasticity and toughness. The polymer (P27K) is mixed with a cross-linking agent at the time of implantation and is injected as a fluid through the anular defect where it adheres to the surrounding intradiscal tissue as it cures. There is no measurable temperature increase produced during the cure process. NuCore has been studied as an adjunct to discectomy27 and is in the process of being studied for treatment of DDD (Fig. 163-4).
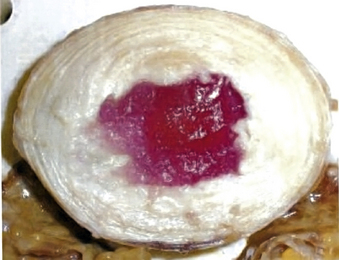
FIGURE 163-4 NuCore Injectable Disc Nucleus (red), shown interdigitating with normal disc after injection into nucleotomy defect.
(Used with permission of Spine Wave, Shelton, CT.)
The NeuDisc device (Replication Medical, Cranbury, NJ) is composed of a material that is a hydrolyzed polyacrylonitrile hydrogel (Aquacryl). In the absence of mechanical restrictions, the hydrogel imbibes 90% volume as a liquid. When implanted in a dehydrated state, this hydrogel implant is substantially smaller than the volume of resected nucleus and is easily placed though an incision in the anulus. After hydration, it expands anisotropically, in the axial direction principally, and becomes substantially larger than the incision. The axial expansion is not constrained by a jacket and results from its structure of polymer layers tied together with an internal substructure28 (Fig. 163-5; see also Fig. 163-1).