CHAPTER 54 Nuclear Medicine Imaging of Myocardial Perfusion
The basic principles of MPI have been validated in animal models and clinical trials over the past several decades.1–4 Accepted clinical guidelines for use, technical aspects of quality control, and protocols for MPI stress testing and imaging for positron emission tomography (PET) and single photon emission computed tomography (SPECT) have been summarized in joint statements from the American Heart Association, American Society of Nuclear Cardiology, and American College of Cardiology,5 and the European Association of Nuclear Medicine and the European Society of Cardiology.6 This chapter highlights and summarizes the current clinical aspects of MPI. Emphasis is placed on describing the rationale and principles of MPI. The authors acknowledge variations in clinical practice based on local expertise and preference; however, space limitations do not permit a detailed discussion of all issues.
The first section reviews current clinical MPI using SPECT. Topics include radiotracers, instrumentation, procedures, and data analysis, including quantification and interpretation. Test performance and prognosis are reviewed under the general topic of interpretation. Assessment of myocardial viability with MPI also is briefly summarized (this is discussed more extensively in Chapter 55). Gated MPI to assess ventricular function is discussed in Chapter 56. Planar imaging produces lower image contrast and is reserved for special circumstances such as claustrophobic patients or patients unable to undergo SPECT imaging. An extensive review has been published.7 The second section reviews MPI with PET. The same general outline is followed in an abbreviated format with salient differences discussed between PET and SPECT.
MYOCARDIAL PERFUSION IMAGING WITH SPECT
Technical Aspects
Radiotracers
After administration, thallium 201 begins to redistribute significantly as it equilibrates with the extracellular concentration. In ischemic territories of lower MBF at rest, lower washout of radiotracer also contributes to equilibration. This more uniform myocardial radiotracer distribution at delayed (typically 4 hours) time points (“redistribution”) is deemed “reversible” compared with stress perfusion, and a functionally significant coronary stenosis can be noninvasively diagnosed. Previous studies have verified that administration of a small reinjection of thallium 201 of 37 MBq (1 mCi) at rest improves the detection of viable myocardium.8 This is a widely accepted clinical procedure to enhance the detection of viable myocardium.
Tc 99m-sestamibi is a lipophilic cationic compound. Tc 99m-tetrofosmin is a diphosphine lipophilic cationic complex of Tc 99m. Tetrofosmin is reported to have faster clearance from the liver and lungs; however, the clinical significance with respect to improved diagnostic accuracy has yet to be established. A large retrospective study has shown that Tc 99m-tetrofosmin scans are essentially equivalent to Tc 99m-sestamibi in determining prognosis in high-risk patients.9 Examples of normal Tc 99m-sestamibi studies are shown in Figures 54-1 and 54-2. A gated study showing the utility of aiding in identifying a breast attenuation artifact is shown in Figure 54-3.
Instrumentation
A modest improvement in image resolution may not be deemed necessary if the diagnostic accuracy is not significantly improved, particularly if increasing imaging time results in a higher frequency of patient motion artifacts. Advanced instrumentation and software are currently being tested, with the goal of reducing imaging time while preserving image quality. The specific details of typical clinical instrumentation, including gamma cameras, collimators, and quality assurance (QA), have been well described in a detailed review article.10
Techniques in SPECT
Indications
The American College of Cardiology Foundation and the American Society of Nuclear Cardiology jointly published guidelines for appropriateness criteria.11 The indications deemed most appropriate were those in which patients presented with intermediate or high pretest probabilities in the categories described subsequently. MPI as a screening tool in very low pretest probability patients is generally considered inappropriate.
Appropriate patient populations include the following categories: (1) detection of CAD in symptomatic or asymptomatic patients (chest pain, newly diagnosed heart failure or diastolic dysfunction, newly diagnosed arrhythmias including atrial fibrillation and ventricular fibrillation); (2) risk assessment in patients with intermediate or high pretest probability of CAD; (3) risk assessment in patients with known coronary disease; and (4) evaluation of myocardial viability. Although other indications may be warranted, the above-listed indications were judged to be most appropriate in most referred cases. Typical MPI patterns are shown in Figures 54-4 through 54-6.
A unique clinical scenario for MPI is in the evaluation of acute coronary syndromes in patients presenting to the emergency department.12 In patients without a history of prior myocardial infarction (MI) and an intermediate probability of CAD, the sensitivity for detection is very high. In this population actively having chest pain at the time of radiotracer administration, the negative predictive value of normal MPI is 99% to 100%. If the chest pain has resolved at the time of radiotracer administration, test sensitivity is modestly reduced, and current guidelines recommend repeating radiotracer administration within 2 hours of symptom abatement.12 Because Tc 99m perfusion agents do not have significant redistribution for 6 hours, imaging can be performed after resolution of chest pain and still reflects the myocardial perfusion at the time administration. In patients presenting to the emergency department with chest pain that has resolved, and in whom recent myocardial injury has been excluded by a chest pain protocol including ECG and cardiac enzymes, a subsequent stress MPI study can be safely performed to exclude functional CAD.
Pitfalls and Solutions
Quality Assurance
A QA program for gamma camera SPECT operation is essential to avoid artifacts, which could result in misinterpretation of test results. Proof of the establishment and diligent adherence to an equipment QA program is an integral part of laboratory accreditation by the American College of Radiology and the Intersocietal Commission for the Accreditation of Nuclear Medicine Laboratories. Conventional planar and specific SPECT and PET imaging QA procedures should be performed and recorded periodically. Daily QA includes ensuring correct isotope energy peak, and “daily floods” to assess gamma camera imaging field uniformity. Weekly QA includes resolution and linearity checks with “bar phantoms.” Many manufacturers include software that can automatically compute planar measurements including differential and integral flood field uniformity and intrinsic linear resolution from bar phantoms.10
Many SPECT systems include attenuation correction components, using radioactive scanning line sources, low-end CT devices, or diagnostic-quality CT. These attenuation-correcting devices require their own set of daily, weekly, and annual QA procedures. Likewise, PET and PET/CT scanners have specific QA requirements, as specified by the equipment manufacturers and as mandated by laboratory accreditation agencies. Typical PET and PET/CT QA procedures have been reviewed in a more recent publication.10
Description of Techniques and Protocols
Radionuclide Imaging Protocols
Pharmacologic Protocols—Adenosine
Adenosine is a coronary vasodilator commonly used in combination with MPI.13 This is a purine base, endogenously produced by myocardial smooth muscle and vascular endothelium. It is derived through extracellular dephosphorylation of adenosine triphosphate (ATP) and adenosine diphosphate (ADP). There are four known receptor subtypes specific for adenosine. A2A is considered a cardiac specific receptor, through which coronary vasodilation is initiated after intravenous adenosine administration.
Pharmacologic Protocols—Dipyridamole
Dipyridamole is also a commonly used vasodilator for MPI stress testing.14 It is an indirect vasodilator that acts by blocking the cellular metabolism of adenosine. This results in a high local interstitial concentration, which subsequently results in coronary vasodilation. Dipyridamole is infused at a rate of 142 µg/kg/min over 4 minutes. The most common side effects include headache, flushing, hypotension, nausea, and chest discomfort. Because of a relatively longer biologic half-life of dipyridamole, the radiotracer is administered at 6 minutes after the start of the dipyridamole infusion. In addition, side effects may last for several minutes, but can be rapidly reversed by intravenous infusion of aminophylline, which acts by competitively inhibiting adenosine binding to its receptors.
Pharmacologic Protocols—Dobutamine
Dobutamine is a β-adrenergic agent with a biologic half-life of 2 minutes. It acts by increasing myocardial contraction and heart rate, which subsequently increases myocardial oxygen demand and blood flow.15 It is considered an alternative stress agent when an exercise or pharmacologic vasodilator study cannot be performed. Intravenous administration starts at an initial dose of 5 to 10 µg/kg/min for 3 minutes, with subsequent increases to doses of 20 µg/kg/min, 30 µg/kg/min, and 40 µg/kg/min in 3-minute stages, or until the goal of reaching 85% of maximal heart rate target is achieved. At approximately 1 to 2 minutes after target heart rate is achieved, the radiopharmaceutical is administered intravenously.
SPECT Imaging Protocols
Several protocols using thallium 201, Tc 99m, or both radiotracers have been well studied and characterized with respect to accuracy. Although modest differences exist, overall accuracy of the most common protocols is comparable. When using same-day protocols with Tc 99m radiotracers, however, the resting study should be performed first to avoid “false-positive” fixed defects.16 If stress imaging is performed first, the higher MBF at stress combined with a relatively high stress radiotracer injection activity may lead to overestimation of MI because the subsequent resting images also reflect stress tracer distribution. If the resting study is performed first, the subsequent stress activity is much higher (approximately three times higher), and the increased blood flow at stress (approximately 2.5 to 3 times higher) produces a higher “weighting” on the stress images; this effectively reduces the contribution of the previous resting activity on the stress images. The number of potential false-negative results for ischemia is small because the resting activity has a relatively small contribution to the stress imaging.
Image Interpretation
Postprocessing
Automated boundary detection programs define endocardial and epicardial borders of the myocardium. Applied to all cycles of gated studies, the left ventricular volumes provide measurements of global and regional left ventricular function and ejection fraction. Several groups and manufacturers have implemented their algorithms into commercially available software. Because of differences in physical modeling assumptions, algorithm implementation, and boundary detection methods, different software packages have different normal values and may produce significantly different results.17 Direct comparisons between values produced from different software packages should be performed carefully and should take into account algorithm differences, including the use of algorithm-specific databases of normal limits.
Interpretation
If there is a concern that an apparent perfusion defect may represent an artifact owing to patient motion, repeat imaging (Tc 99m radiotracers or resting thallium 201) should be considered to clarify the issue. Although motion correction software may reduce the effect of patient motion, software may be unable to eliminate completely the effect of patient motion on the final reconstructed tomographic images. Any application of motion-correcting software must be reviewed carefully to verify that the algorithm performed the intended correction appropriately, as part of routine patient-specific QA. Generally, vertical motion errors are detected and corrected more reliably than horizontal and bulk body motion errors. An example of a motion artifact is shown in Figure 54-7.
Three physiologic parameters can be assessed from the projection data. The first is left ventricular cavity size. It is possible to appreciate markedly decreased counts within the left ventricular cavity, and a relatively large cavity compared with the myocardial thickness indicates left ventricular dilation. Measurements of ventricular size from the computer-generated boundaries may also confirm left ventricular enlargement. In patients with prior apical infarction and left ventricular dysfunction, ungated images may show a markedly decreased left ventricular cavity activity because of abnormally and severely decreased wall motion adjacent to infarction. In patients with an area of severely decreased activity at the apex because of prior infarction, severely decreased wall motion or dyskinesis may contribute to an almost entirely absent apical defect of attenuating blood pool (“black hole sign”; Fig. 54-8) that is associated with a higher incidence of apical aneurysm.
When interpreting and reporting MPI, important aspects include perfusion defect size, severity, and reversibility. Although a visual interpretation may be accurate with respect to the presence or absence of coronary disease, quantitative information has prognostic significance. The size and severity of the perfusion defect are predictive of cardiac events, including MI and cardiac death.18 These are important for risk stratification and patient management. Small, mild defects are associated with low cardiac mortality of less than 1% per year.19 Severe defects and extensive ischemia are associated with significantly higher cardiac events and worse short-term prognosis. In addition, the short-term outcome for cardiac events in patients with mild ischemia is worse for patients undergoing revascularization.20 In contrast, the short-term cardiac event rate for patients with severe defects is lower for patients undergoing revascularization compared with medical management. Quantitative measures of myocardial perfusion scintigraphy may determine the most appropriate subsequent therapy in patients with known CAD.20
As discussed in Chapter 55, global and regional left ventricular function are very important parameters for clinical management. Left ventricular functional assessment by left ventricular ejection fraction is highly predictive of mortality. Regional functional assessment may also influence clinical management. A segment of dysfunctional myocardium with preserved viability may be important from the perspective of potential revascularization.
Other Artifacts and Normal Variants
Misregistration of SPECT and Computed Tomography
With the introduction of fast multislice CT, it is possible that a similar “artifactual” defect could be seen on SPECT if corrected with high temporal resolution CT. Cardiac PET studies have shown clearly potential artifactual “perfusion” defects owing to misalignment of the PET emission and the transformed CT transmission image used for attenuation correction. This is due to differences in the respiratory cycle during CT and the average PET emission diaphragm position. Data for a single high-resolution CT transaxial slice can be acquired in a fraction of a second, whereas PET emission data typically are acquired over several minutes to 15 to 30 minutes. Cardiac PET data may be ECG gated, but data are not currently gated with respiratory cycle information. The frequency of misalignment is high (42% in a retrospective study21); however, impact on the emission corrected images is still under investigation.
Test Accuracy
Detection of Coronary Artery Disease
Stress perfusion scintigraphy with SPECT has the highest accuracy among all imaging modalities in the noninvasive detection of functionally significant CAD. Overall, the diagnostic accuracy is approximately 85% to 90%, with sensitivity and specificity varying depending on the patient population and methodology of the study. In women with functionally significant CAD (>70% stenosis), gated Tc 99m-sestamibi reported diagnostic sensitivity is 80%, and specificity is 92%.22 Other studies have reported higher sensitivities and specificities of 90% and 93%.3,23
Myocardial Viability
A more recent study comparing rest-redistribution thallium 201 with myocardial delayed hyperenhancement by MRI confirmed high quantitative correlation of infarct size (r = 0.90).24 Thallium 201 rest-redistribution also correlates well with viability by 18FDG-PET.8 Despite being “fixed” perfusion defects, mild and moderate (<50% decreased counts compared with normal areas) defects are considered viable. Viable regions by thallium 201 showed a 98% concordance with viability by 18FDG-PET. Only a relatively small proportion (approximately 30%) of severe fixed defects by thallium 201 showed discordant viability by 18FDG-PET.8 This detection of myocardial viability can determine the suitability of a candidate for revascularization.
Rest-redistribution and stress-redistribution-reinjection thallium 201 with SPECT are considered highly accurate in evaluating myocardial viability; however, PET has better spatial resolution and attenuation correction, and is generally considered superior for this indication. MRI has shown comparable results for viability determination, and is able to determine the extent of subendocardial infarction with better spatial resolution.25
MYOCARDIAL PERFUSION IMAGING WITH PET
Clinical Indications
Patients with a high body mass index are especially well suited for PET because of (1) routine use of high-quality attenuation correction, and (2) higher photon detection sensitivity, owing to a lower proportion of photon scatter and tissue attenuation compared with SPECT. In addition, PET scans generally contain more than 100 times as many counts as do SPECT scans, particularly for three-dimensional data acquisitions, as opposed to two-dimensional PET data acquisitions. An example of an ammonia N 13 dipyridamole PET study is shown in Figure 54-9.
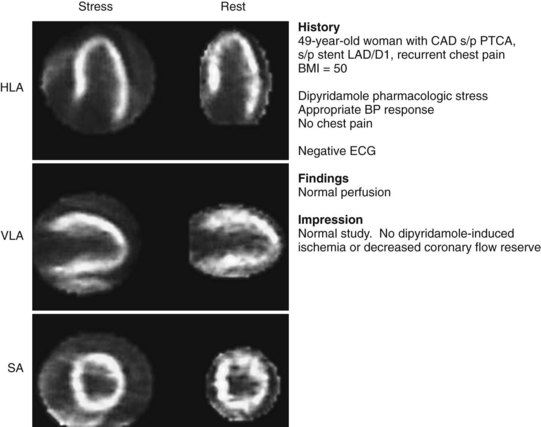
FIGURE 54-9 Normal ammonia N 13 PET myocardial perfusion pharmacologic stress imaging with dipyridamole. Note excellent spatial resolution and more uniform radiotracer distribution compared with traditional SPECT Tc 99m-sestamibi imaging (see Figs. 54-1 and 54-2), despite the patient’s high body mass index (BMI) of 50. BP, blood pressure; HLA, horizontal long axis; LAD/D1, first diagonal branch of the left anterior descending artery; PTCA, percutaneous transluminal coronary angioplasty; SA, short axis; VLA, vertical long axis.
Description of Technique and Protocols
Myocardial Viability
The diagnostic accuracy of 18FDG-PET in the evaluation of myocardial viability is well established.26,27 Revascularization improved left ventricular function in 85% of segments with preservation of 18FDG uptake; no functional improvement was seen in 92% of segments with matched absence of blood flow and 18FDG uptake.26 More recently, 18FDG-PET myocardial viability used to select revascularization candidates was associated with significantly lower in-hospital death rate, improved 12-month survival, and reduced complicated perioperative recovery.28 Fluorine 18 is a positron imaging isotope with a physical half-life of 110 minutes. 18FDG is a glucose analogue, which is transported intracellularly via glucose transporters. It is subsequently phosphorylated to FDG-6-phosphate, and trapped intracellularly without further metabolism because of its stereochemistry. The radiation dose of typical injected activity of 370 MBq (10 mCi) of 18FDG is approximately 11 mSv (1.1 rem).
Myocardial viability with regional dysfunction may be present in a spectrum of overlapping physiologic scenarios ranging from decreased to normal resting MBF. This complex issue is currently evolving as further studies report new data. Classically, hibernating myocardium by PET is defined by reduced resting MBF with preserved myocardial metabolism evidenced by 18FDG uptake. If resting perfusion is normal, intermittent episodes of myocardial ischemia produced by episodes of increased oxygen demand may produce a condition termed stunning. This repetitive, intermittent stress-induced ischemia may be sufficient to produce chronic myocardial dysfunction. In this clinical scenario, resting perfusion by PET may be normal, and there may be preservation of 18FDG uptake in regions of myocardial dysfunction. A matched reduction in blood flow and metabolism is considered nonviable myocardium or infarction. An example of a myocardial viability study is shown in Figure 54-10.
FUTURE DIRECTIONS
The more recent availability of rapid acquisition CT and combined PET/CT and SPECT/CT instrumentation provides the possibility of assessing coronary artery calcifications and coronary anatomy. The technical factors potentially limiting coronary angiography with CT include (1) coronary artery calcification interfering with intraluminal assessment; (2) cardiac motion degrading image quality in patients with high heart rates; (3) radiation dosimetry considerations; and (4) reconstruction streak artifacts caused by high-attenuation structures, such as metallic surgical clips or defibrillators. In contrast to radionuclide techniques that provide information regarding functional significance of stenosis, coronary CT reveals information regarding subclinical CAD. Combined PET/CT to assess CAD and the correction techniques for its limitations are currently active areas of investigation.29,30
CONCLUSION
KEY POINTS

Baggish AL, Boucher CA. Radiopharmaceutical agents for myocardial perfusion imaging. Circulation. 2008;118:1668-1674.
Di Carli MF, Dorbala S, Meserve J, et al. Clinical myocardial perfusion PET/CT. J Nucl Med. 2007;48:783-793.
Hendel RC, Berman DS, Di Carli MF, et al. Appropriate use criteria for cardiac radionuclide imaging. A Report of the American College of Cardiology Foundation Appropriate Use Criteria Task Force, the American Society of Nuclear Cardiology, the American College of Radiology, the American Heart Association, the American Society of Echocardiography, the Society of Cardiovascular Computed Tomography, the Society for Cardiovascular Magnetic Resonance, and the Society of Nuclear Medicine. Circulation. 2009;119:e561-e587.
Russell RR3rd, Zaret BL. Nuclear cardiology: Present and future. Curr Probl Cardiol. 2006;31:557-629.
Shaw LJ, Narula J. Risk assessment and predictive value of coronary artery disease testing. J Nucl Med. 2009;50:1296-1306.
Zoghbi GJ, Dorfman TA, Iskandrian AE. The effects of medications on myocardial perfusion. J Am Coll Cardiol. 2008;52:401-416.
1 Gerson MC. Cardiac Nuclear Medicine, 3rd ed. New York: McGraw-Hill; 1997.
2 Iskandrian AE, Garcia EV. Nuclear Cardiac Imaging: Principles and Applications, 3rd ed. New York: Oxford University Press; 2003.
3 Zaret BL, Beller G. Clinical Nuclear Cardiology: State of the Art. St Louis: Mosby; 1993.
4 Heller GV, Hendel R. Nuclear Cardiology: Practical Applications. New York: McGraw Hill; 2004.
5 Klocke FJ, Baird MG, Lorell BH, et al. ACC/AHA/ASNC guidelines for the clinical use of cardiac radionuclide imaging—executive summary: a report of the American College of Cardiology/American Heart Association Task Force on Practice Guidelines (ACC/AHA/ASNC Committee to Revise the 1995 Guidelines for the Clinical Use of Cardiac Radionuclide Imaging). Circulation. 2003;108:1404-1418.
6 Hesse B, Tagil K, Cuocolo A, et al. EANM/ESC procedural guidelines for myocardial perfusion imaging in nuclear cardiology. Eur J Nucl Med Mol Imaging. 2005;32:855-897.
7 Peter LT, Frans JT. Myocardial perfusion planar imaging (abstract). J Nucl Cardiol. 2006;13:e91-e96.
8 Dilsizian V, Perronefilardi P, Arrighi JA, et al. Concordance and discordance between stress-redistribution-reinjection and rest-redistribution thallium imaging for assessing viable myocardium—comparison with metabolic-activity by positron emission tomography. Circulation. 1993;88:941-952.
9 Borges-Neto S, Tuttle RH, Shaw LK, et al. Outcome prediction in patients at high risk for coronary artery disease: comparison between Tc-99m tetrofosmin and Tc-99m sestamibi. Radiology. 2004;232:58-65.
10 Nichols KJ, Bacharach SL, Bergmann SR, et al. Instrumentation quality assurance and performance. J Nucl Cardiol. 2006;13:e25-e41.
11 Brindis R. ACCF/ASNC appropriateness criteria for single-photon emission computed tomography myocardial perfusion imaging (SPECT MPI)—a report of the American College of Cardiology Foundation Quality Strategic Directions Committee Appropriateness Criteria Working Group and the American Society of Nuclear Cardiology. J Am Coll Cardiol. 2005;46:1587-1605.
12 Wackers FJT, Brown KA, Heller GV, et al. American Society of Nuclear Cardiology position statement on radionuclide imaging in patients with suspected acute ischemic syndromes in the emergency department or chest pain center. J Nucl Cardiol. 2002;9:246-250.
13 Verani MS. Pharmacological stress with adenosine for myocardial perfusion imaging. Semin Nucl Med. 1991;21:266-272.
14 Botvinick EH, Dae MW. Dipyridamole perfusion scintigraphy. Semin Nucl Med. 1991;21:242-265.
15 Geleijnse ML, Elhendy A, Fioretti PM, et al. Dobutamine stress myocardial perfusion imaging. J Am Coll Cardiol. 2000;36:2017-2027.
16 Taillefer RF, Gagnon AF, Laflamme LF, et al. Same day injections of Tc-99m methoxy isobutyl isonitrile (hexamibi) for myocardial tomographic imaging: comparison between rest-stress and stress-rest injection sequences. Eur J Nucl Med. 1989;15:113-117.
17 Arik W, Piotr JS, Mathews BF, et al. Quantitative myocardial-perfusion SPECT: comparison of three state-of-the-art software packages (abstract). J Nucl Cardiol. 2008;15:27-34.
18 Hachamovitch R, Hayes SW, Friedman JD, et al. Stress myocardial perfusion single-photon emission computed tomography is clinically effective and cost effective in risk stratification of patients with a high likelihood of coronary artery disease (CAD) but no known CAD. J Am Coll Cardiol. 2004;43:200-208.
19 Metz LD, Beattie MF, Hom RF, et al. The prognostic value of normal exercise myocardial perfusion imaging and exercise echocardiography: a meta-analysis. J Am Coll Cardiol. 2007;49:227-237.
20 Hachamovitch RF, Hayes SW, Friedman JD, et al. Comparison of the short-term survival benefit associated with revascularization compared with medical therapy in patients with no prior coronary artery disease undergoing stress myocardial perfusion single photon emission computed tomography. Circulation. 2003;107:2900-2907.
21 Goetze S, Wahl RL. Prevalence of misregistration between SPECT and CT for attenuation-corrected myocardial perfusion SPECT. J Nucl Cardiol. 2007;14:200-206.
22 Taillefer R, DePuey EG, Udelson JE, et al. Comparative diagnostic accuracy of Tl-201 and Tc-99m sestamibi SPECT imaging (perfusion and ECG-gated SPECT) in detecting coronary artery disease in women. J Am Coll Cardiol. 1997;29:69-77.
23 Beller GA, Zaret BL. Contributions of nuclear cardiology to diagnosis and prognosis of patients with coronary artery disease. Circulation. 2000;101:1465-1478.
24 Fieno DS, Louise EJT, Piotr S, et al. Quantitation of infarct size in patients with chronic coronary artery disease using rest-redistribution Tl-201 myocardial perfusion SPECT: correlation with contrast-enhanced cardiac magnetic resonance (abstract). J Nucl Cardiol. 2007;14:59-67.
25 Schinkel AF, Poldermans DF, Elhendy AF, et al. Assessment of myocardial viability in patients with heart failure. J Nucl Med. 2007;48:1135-1146.
26 Tillisch J, Brunken R, Marshall R, et al. Reversibility of cardiac wall-motion abnormalities predicted by positron tomography. N Engl J Med. 1986;314:884-888.
27 Saha GB, MacIntyre WJ, Brunken RC, et al. Present assessment of myocardial viability by nuclear imaging. Semin Nucl Med. 1996;26:315-335.
28 Haas F, Haehnel CJ, Picker W, et al. Preoperative positron emission tomographic viability assessment and perioperative and postoperative risk in patients with advanced ischemic heart disease. J Am Coll Cardiol. 1997;30:1693-1700.
29 Di Carli MF, Hachamovitch R. Hybrid PET/CT is greater than the sum of its parts: relationship between CT coronary angiography and stress perfusion imaging in patients with suspected ischemic heart disease assessed by integrated PET-CT imaging. J Nucl Cardiol. 2008;15:118-122.
30 Di Carli MF, Dorbala S, Curillova Z, et al. Relationship between CT coronary angiography and stress perfusion imaging in patients with suspected ischemic heart disease assessed by integrated PET-CT imaging. J Nucl Cardiol. 2007;14:799-809.