Chapter 50 Noninvasive Ventilation
Concepts and Practice
Historical Perspective
Dalziel, in 1832, reported the use of a bellows-operated box with a seal around the neck or shoulders to provide artificial respiration to a drowned seaman.1 In 1867, Woillez designed the first workable noninvasive negative-pressure ventilator, which was operated manually.2 Doe, in 1989 described a box used for resuscitating newborns.3 Drinker developed the first tank ventilator in 1928 that was subsequently used with great clinical value in patients with poliomyelitis.4 Negative-pressure ventilation was introduced for use with neonates in the 1960s.5,6 In 1953, Lassen reported that positive-pressure ventilation administered using an endotracheal tube or a tracheostomy was more successful that negative-pressure ventilation in treating patients with poliomyelitis.7 Major technologic advances in the design of positive-pressure ventilators led to the marked decrease in negative-pressure ventilation. Noninvasive positive-pressure ventilation by facemask was first used by Barach et al.8 for the treatment of hypoxemic respiratory failure secondary to acute pulmonary edema. Gregory et al.,9 in 1971, reported that constant positive airway pressure (CPAP) through nasal prongs was effective in improving oxygenation in neonates with hyaline membrane disease. Vidyasagar and Chernick showed that constant negative pressure applied about the chest and abdomen was as effective as CPAP in improving oxygenation in neonates with hyaline membrane disease.10 Despite these early reports, noninvasive ventilation did not become popular until more recently. There is a renewed interest in noninvasive ventilation coinciding with technologic advances in the manufacture and design of noninvasive ventilators. NIV through a mask for neuromuscular disorders was pioneered in the 1980s by Rideau et al.11 and subsequently by Bach et al.12
Short-Term Noninvasive Ventilation
The indications for use of NIV in acute care and critical care situations are given in Box 50-1. The selection guidelines are given in Box 50-2. The first step is to determine whether a patient has a reversible cause of respiratory failure. The second step is to determine whether the patient needs positive pressure support to maintain gas exchange. Before attempting NIV in acute respiratory failure, the clinician must answer the following questions:
Figure 50-1 shows the algorithm to be followed for initiation of NIV in acute respiratory failure. If NIV is used in acute situations, there should be a determination within 1 to 3 hours whether the patient has improved. Signs of success and failure are given in Box 50-3. A good response to NIV is indicated by: (1) reduction in respiratory rate, (2) reduction in work of breathing, (3) reduction in dyspnea, (4) improvement in pH, (5) improvement in oxygenation, and (6) reduction in PaCO2. Additionally, there may be hemodynamic effects such as a reduction in heart rate, improved blood pressures, and perfusion. Generally, for short-term use, NIV is used continuously until the patient improves or fails NIV. The goals of short-term NIV are given in Box 50-4.
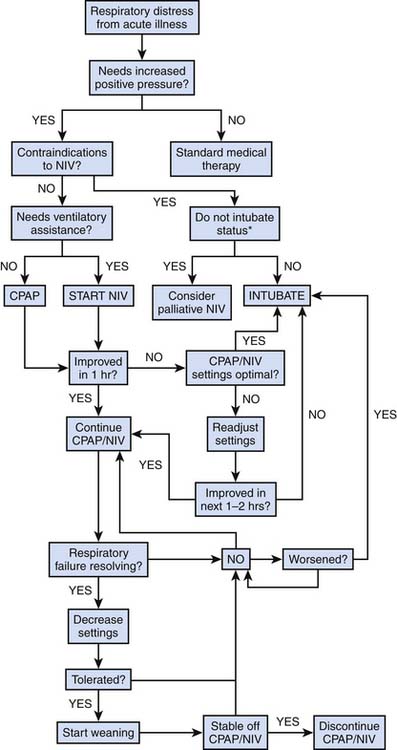
Figure 50–1 Algorithm for initiation and weaning of short-term continuous positive airway pressure and NIV.
Adult Studies on the Short-Term Use of Noninvasive Ventilation
Adult studies have shown that NIV can be used in hypoxemic respiratory failure (PaO2/fraction of inspired oxygen [FiO2] ratio <300) with bilateral parenchymal disease to prevent endotracheal intubation. Ferrer et al. showed that NIV reduced the intubation rate from 52% to 25% with a reduction in intensive care unit (ICU) mortality rate from 39% to 18%.13 But, this finding must be tempered by studies that show that NIV may not reduce the need for endotracheal intubation.14,15 CPAP, not strictly a form of ventilatory assistance, was described by Barach et al.8 for the treatment of acute cardiogenic pulmonary edema. Since then, several studies have demonstrated that both CPAP and NIV can decrease the rate of intubation, improve work of breathing and improve gas exchange.16–18 In adults with acute exacerbations of chronic obstructive pulmonary disease, NIV has been shown to improve both short-term and long-term outcomes.19–21 It is reasonable to expect a similar response to acute asthma because many of the pathophysiologic features are similar. Uncontrolled studies and one randomized controlled study showed improvement in patients having an acute asthma attack.22–24 It is desirable to reduce the probability of nosocomial infections in immunocompromised patients. Therefore, it is appealing to apply NIV to avoid endotracheal intubation in these patients. Adult studies have shown that NIV can reduce the rate of intubation and may improve short term mortality.25–27
Adult studies have shown that respiratory insufficiency that develops postoperatively in patients can be successfully treated with NIV. NIV reduces the rate of intubation and is more effective than CPAP or chest physiotherapy.28,29 In patients after lung resection surgery, NIV reduced the rate of intubation and mortality compared with conventional therapy.30 Children with restrictive chest diseases who develop respiratory failure after surgery such as spinal fusion are good candidates for NIV if they develop postoperative respiratory failure provided they do not have any other contraindications.
Endotracheal intubation and invasive mechanical ventilation are associated with complications including ventilator-associated pneumonia, ventilator-induced lung injury, and airway trauma. To minimize these complications, it is desirable to extubate the patient as quickly as possible. Generally, extubation coincides with the end of weaning (i.e., the patient is not placed on any form of positive pressure support postextubation). In some patients, who take a long time to wean, but require fairly low ventilatory assistance, it is possible to extubate them to NIV. Theoretically, it would reduce the incidence of complications associated with endotracheal intubation and invasive mechanical ventilation. Udwadia et al. were among the first to test this paradigm in 22 adult patients who were successfully stabilized on NIV after extubation.31 Three adult studies have demonstrated that the use of NIV resulted in a reduction in the duration of mechanical ventilation, a reduction in the incidence of nosocomial pneumonia, and a reduction in the duration of hospital stay.32–34 Similar pediatric studies are lacking. NIV to facilitate extubation should be carried out only if the patient meets the following criteria: (1) is awake and alert, (2) has intact bulbar function with a preserved cough reflex, (3) has minimal airway secretion, (4) is able to breathe spontaneously, (5) has stable hemodynamic status, (6) does not have gastric distension, and (7) has a requirement for 40% or less supplemental oxygen. Recently, some adult studies have examined the use of NIV for postextubation respiratory failure (i.e., respiratory failure that develops after extubation on complete spontaneous breathing). The rationale for the use of NIV in this circumstance is the avoidance of endotracheal intubation. In at least one study in adults, this approach was shown to increase the time from respiratory failure to intubation with an increase in mortality rate.35 It is difficult to extrapolate from adult studies to pediatrics. It is possible that some children may benefit from the institution of NIV for postextubation respiratory failure.
Pediatric and Neonatal Studies
In 1981, Sullivan et al.36 first demonstrated that nasal CPAP was effective in reversing obstructive sleep apnea in four adults and one teenager. Guilleminault et al.,37 in 1986, reported that nasal CPAP reversed or reduced obstructive sleep apnea in some children. There were problems related to poor cooperation from parents and difficulty in preventing leaks around the nasal mask. Since the first report, several studies have shown that nasal CPAP was effective in obstructive sleep apnea in infants and children.37–42 In 1995, Guilleminault et al.43 reported that nasal CPAP was successful in 70 of 72 infants with sleep-disordered breathing. In many instances, it provided an interim solution while allowing the patient to grow and postponing surgery.
Patients in a fairly wide age range appear to tolerate NIV therapy in the acute setting, provided they are adequately monitored and sedated. In 1993, Akingbola et al.44 published a case report describing effective NPPV therapy in two pediatric patients with acute respiratory distress. Since that time, NIV has been applied to pediatric patients with a variety of respiratory disorders associated with acute hypoxemic respiratory failure, including pneumonia, pulmonary edema, postoperative respiratory decompensation in sleep apnea syndrome, status asthmaticus, spinal muscular atrophy, and end-stage cystic fibrosis.45–51
Padman et al.52 published a preliminary report on 15 patients ages 4 to 21 years who had respiratory failure because of cystic fibrosis (four patients) and neuromuscular disease (11 patients). In 14 patients, the placement of an artificial airway was avoided. They observed significant improvement in hospital days, respiratory rate, heart rate, serum bicarbonate, arterial carbon dioxide, dyspnea, activity tolerance, and quality of sleep. In 1995, Fortenberry et al. reported a retrospective study on the efficacy and complications of biphasic positive airway pressure (Bi-PAP) in 28 children with acute hypoxemic respiratory insufficiency.47 Bi-PAP significantly decreased respiratory rate and improved both oxygenation and ventilation. The use of Bi-PAP decreased hospitalization rate and increased patient comfort. Only three of 28 patients required intubation or reintubation. Padman et al. conducted a prospective study in 34 patients with impending failure all of whom required airway or oxygenation/ventilation support and required admission to the pediatric ICU.50 A decrease in respiratory rate, heart rate, and dyspnea score, and an improvement in oxygenation were noted in more than 90% of patients studied. The frequency of intubation in these patients was only 8%. Birnkrant et al.51 reported their experience with Bi-PAP in six patients with spinal muscular atrophy and three patients with other causes of respiratory failure. This uncontrolled study showed that in these patients noninvasive positive-pressure ventilation facilitated extubation. Teague et al.53 reported that NIV treatment acutely improved oxygenation and reduced cardiorespiratory distress in 19 of 26 patients. This group of “NIV responders” had significantly shorter lengths of stay in both the ICU and hospital. However, the seven nonresponders required endotracheal intubation as respiratory distress progressed despite sedation. Teague et al.53 also showed that NIV improves gas exchange in children with upper airway obstruction In a prospective, randomized, controlled trial of NIV in children with acute respiratory failure, Yan et al.54 showed that NIV improved hypoxemia and the signs and symptoms of acute respiratory failure and reduced the rate of endotracheal intubation. Thill et al.,55 in a randomized, crossover study in children with lower airway obstruction, showed that NIV decreased signs of work of breathing such as respiratory rate, accessory muscle use, and dyspnea as compared with standard therapy with no serious associated morbidity. Pancera et al.56 recently published their experience with NIV in immunocompromised pediatric patients. NIV as the first-line therapy was compared to invasive mechanical ventilation. Seventy-five percent of the patients treated with NIV did not require endotracheal intubation showing that NIV is a viable first-line therapy for respiratory failure in immunocompromised patients for preventing endotracheal intubation.56 More recently, NIV has been suggested as an alternative to endotracheal intubation for the perioperative management of scoliosis correction in patients with muscular dystrophy.57
Interfaces
A properly fitting facial appliance is essential for the optimal application of NIV. There are currently several devices in use: (1) oral-nasal mask, (2) nasal mask, (3) total face mask, (4) Adam’s circuit, (5) head hood, (6) nasal prongs, and (7) mouthpieces. A correctly sized interface minimizes leaks, improves effectiveness of positive pressure support, and improves comfort.
Equipment
A detailed description of the devices available for NIV at home is beyond the scope of this chapter and the reader is referred to several excellent reviews.58,59 This discussion focuses on the characteristics of positive-pressure ventilators that can be used for providing NIV. Negative-pressure ventilators are discussed elsewhere in the chapter. The ventilators that are used for NIV can be classified into three categories: (1) conventional ICU ventilators with a double limb circuit without leak compensation, (2) devices with a single limb circuit with leak compensation, and (3) devices that combine the above two categories to include both leak compensation and having a double-limb circuit. Category 1 devices can only be used in the hospital. Category 2 and 3 devices can be used both in the hospital and at home. The examples of these devices would be any of the conventional ICU ventilators that can provide pressure support with positive end-expiratory pressure (category 1), Respironics Bi-PAP (category 2), and the Pulmometrics LTV ventilator (category 3). The performances of these ventilators vary widely in delivered tidal volumes, air-leak compensation, response to simulated effort, inspiratory trigger, expiratory cycling, rebreathing, response to high ventilatory demands, and patient-ventilator synchrony.60 Category 1 or ICU ventilators operate with high-pressure gas sources with an oxygen blending system. Category 2 and 3 devices can operate without a high-pressure gas source. Bilevel ventilators do not have a blender; therefore the delivered FiO2 is unpredictable depending on the oxygen flow rate, ventilator settings, amount of leak, site of oxygen enrichment, and the type of exhalation port.61
Optimizing Patient-Ventilator Interaction
Optimal patient-ventilator interaction requires that the ventilator be able to detect the patient’s inspiratory efforts as quickly as possible and terminate inspiration (expiratory cycling) as close to the beginning of the patient’s expiration as possible. Inspiratory trigger function differs significantly among the ventilators.62–64 Factors affecting the inspiratory trigger function include trigger response to the inspiratory flow, leak-induced auto-triggering, and pressure-time and flow-time waveform heterogeneity.62–64 Strategies to optimize expiratory cycling include setting a suitable threshold for the inspiratory time and adjusting the expiratory cycling flow threshold. Patients with obstructive lung disease tend to do better with high inspiratory flow, whereas patients with neuromuscular disease seem to do better with low inspiratory flows.65 An adjustable backup rate is available in most modern ventilators used for NIV. This is particularly useful when sedation is used to improve patient compliance with NIV. Leaks around the interface reduce its effectiveness. Some amount of leak around the interface is expected with NIV. Complete elimination of air leak is not desirable because it comes at the expense of a very tight-fitting mask that may lead to patient discomfort and skin breakdown. Ventilators differ in their capacity to compensate for leaks. One of the drawbacks of a large airleak is auto-triggering. Humidification is important to prevent mucosal drying. Humidification can be provided using a heated humidifier, HME, or a pass-over humidifier.
Ventilator Settings
With CPAP, the pressure level is selected based on clinical need. There should be relief of symptoms within 1 to 2 hours of application of CPAP. With short-term NIV, two strategies have been employed. One approach is to start with a high inspiratory pressure (about 20 to 25 cm H2O). The goal of such an approach is rapid relief of symptoms. If successful, a lower level that also is tolerated is selected. This approach is called the high-low approach. The other approach starts with a lower inspiratory pressure (about 8 to 10 cm H2O) and gradually increased to produce relief of symptoms. The aim of this approach is to maximize patient comfort and tolerance. This is called the low-high approach. Within the first 1 to 3 hours, subsequent adjustments may be necessary depending on the patient response. Expiratory pressure is used routinely during short-term NIV. Maximal FIo2 with bilevel ventilators is usually about 45% to 50%. If a higher FIO2 is required, and endotracheal intubation is not indicated, then an ICU-type ventilator may be used.
Complications and Concerns During Short-Term Noninvasive Ventilation
Long-Term Noninvasive Ventilation
Long-term NIV is indicated in conditions that result in chronic respiratory failure. This can be either progressive or nonprogressive. Generally, long-term NIV is intermittent with periods off NIV. The most common form of long-term NIV is nocturnal NIV with off-periods during the daytime. The goals of long-term NIV are given in Box 50-4. The indications for long-term NIV are given in Box 50-5.
Physiologic Effects and Outcomes of Long-Term Noninvasive Ventilation
Intermittent NIV consistently improves daytime gas exchange.66,67 Patients with neuromuscular and chest wall disorders often have sleep-related disturbances.68 NIV ameliorates hypoventilation, intermittent sleep apneas, and other sleep-disordered breathing.69 In children with neuromuscular disorder, symptoms of daytime sleepiness, headache, and sleep quality improved after initiation of NIV.70 Hospitalization rates and health care costs decreased. Quality of life remained stable after NIV, despite disease progression.70 NIV, applied appropriately, improves the quality of life with restrictive thoracic disorders.71 Children with spinal muscular atrophy (types 1 and 2) often develop thoracoabdominal asynchrony, especially during sleep. NIV improved sleep breathing parameters and thoracoabdominal coordination during sleep in spinal muscular atrophy Types 1 and 2.72 Long-term NIV combined with mechanically assisted coughing has also been shown to increase survival in patients with Duchenne muscular dystrophy.73
Negative-Pressure Ventilation
Design and Modes of Negative-Pressure Ventilators
All negative-pressure ventilators have a chamber in which subatmospheric pressure is generated and a pump which generates this pressure. The chamber may cover only the chest and the upper abdomen (cuirass) or all of the extracranial portions of the body (tank respirator, isolette, or a body suit). The tank respirator has both the chamber and the pump in one unit. In all other cases the two units are separate. The cuirass can be prefabricated or custom designed to fit the contours of the chest. Custom-designed cuirasses are especially useful in patients with skeletal or spinal deformities. All body suits fit over a hard shell similar to the cuirass placed over the chest and the upper abdomen. Most negative pressure pumps in use today are pressure cycled. Some volume-cycled pumps have been used but their use has been limited because the pump cannot compensate for the variable amounts of air leak. Additionally, some ventilators provide an inspiratory assist mode. At the present time, there are four modes of negative pressure application: (1) cyclical negative pressure, (2) negative/positive pressure, (3) continuous negative extrathoracic pressure (CNEP), and (4) negative pressure/CNEP. Cyclical negative-pressure ventilation refers to a mode where the ventilator generates the preset subatmospheric pressure during inspiration while expiration is passive. Negative/positive-pressure ventilation refers to a mode which is a combination of negative pressure during inspiration with positive pressure during expiration. CNEP refers to a mode in which a constant subatmospheric pressure is provided throughout the respiratory cycle and the patient breathes spontaneously. Negative pressure/CNEP refers to a mode where negative pressure inspiratory cycles are superimposed on CNEP.
Clinical Applications
Box 50-6 shows the indications, advantages, disadvantages, contraindications, and clinical side effects of negative-pressure ventilation. In 1965, Stahlman et al.74 first reported on the use of a tank respirator to treat neonates with respiratory distress syndrome. Vidyasagar and Chernick,10 in 1971, were the first to show that CNEP improved oxygenation in neonatal respiratory distress syndrome. In the 1970s, several controlled and uncontrolled studies showed that negative-pressure ventilation was effective in the management of neonatal respiratory syndrome.75–77 However, the utility of this technique was limited because of problems such as upper airway obstruction, difficulties in achieving access to patients, difficulties in achieving an adequate seal, sores from the neck seal, and inability to maintain an adequate neutral thermal environment. Negative-pressure ventilation has been reintroduced in the treatment of neonatal respiratory distress syndrome. A recent randomized control study showed that application of CNEP was associated with fewer intubations and decreased the total duration of oxygen therapy.78 However, CNEP was associated with a slightly higher (not statistically significant) mortality rate, cranial ultrasound abnormalities, and pneumothoraces. Further studies are warranted to assess the utility and safety of negative-pressure ventilation in neonatal respiratory distress syndrome.
Box 50–6 Indications, Advantages, Disadvantages, and Contraindications of Negative Pressure Ventilation
Disadvantages
Respiratory Dysfunction and Failure
During the polio epidemics in the 1930s and 1940s, negative-pressure tank ventilators reduced mortality in patients with spinal polio.79–81 There has been a resurgent interest in negative-pressure ventilation in patients with neuromuscular diseases and skeletal deformities. In adults with kyphoscoliosis and neuromuscular disorders with chronic hypoventilation, intermittent use of negative-pressure ventilation, mostly nocturnal, decreased or reversed day time symptoms of hypoventilation and gas exchange abnormalities.82,83 These studies show that negative-pressure ventilation is useful in acute or chronic respiratory failure associated with skeletal or neuromuscular disorders. Isolated case reports in adults have been published about using CNEP in adult respiratory distress syndrome and in persistent flail chest deformity.84–87 CNEP has been used to assist ventilation in children with diffuse alveolar disease and progressive respiratory failure.88–90 In 1975, Sanyal et al.89 published a case series of five children with progressive respiratory failure from Pneumocystis carinii pneumonitis. Three patients improved with CNP and survived, whereas the other two continued to worsen and died. Sanyal et al.,90 in 1977, published a larger series where CNEP was used to assist ventilation in 14 children who had progressive respiratory insufficiency caused by diffuse bilateral alveolar disease. Within 6 hours after therapy was started, oxygenation improved. The improvement was sustained and within 24 hours permitted a decrease in fractional concentration inspired oxygen. There was a concomitant decrease in intrapulmonary right-to-left shunt and a decrease in respiratory rate. Four of the 14 patients developed pneumothorax that was successfully decompressed. Ten patients survived. These observations establish CNEP therapy as an effective means of improving arterial oxygenation in spontaneously breathing older children, avoiding endotracheal intubation and prolonged use of muscle relaxants and sedatives.
Cardiovascular Disorders
Raine et al.91 in 1992 published a pilot study in 10 children with respiratory failure and found the CNEP, introduced either in conjunction with intermittent positive-pressure ventilation or alone, did not produce large changes in cardiac output. In patients with Fontan-type operations, pulmonary blood flow and cardiac output increases with spontaneous inspiration and positive-pressure ventilation decreases antegrade pulmonary blood flow and may increase pulmonary valvular incompetence in patients with restrictive right heart physiology after repair of tetralogy of Fallot.92 In children after repair of total cavopulmonary connection and tetralogy of Fallot and after Fontan-type procedures, negative-pressure ventilation provided using a Hayek external high-frequency oscillator improved cardiac output by 42% to 46% almost entirely by an increase in stroke volume with improvement in mixed venous oxygen saturation.93 Systemic and pulmonary vascular resistances decreased significantly as well. A similar finding was observed in children after transcatheter occlusion of an asymptomatic patent ductus arteriosus and after open heart surgery.94 Raine et al.95 in 1992 reported in an uncontrolled study that negative-pressure ventilation using either CNEP or intermittent negative-pressure ventilation was a viable alternative to positive-pressure ventilation in patients with phrenic nerve palsy after pediatric cardiac surgery, reduced the need for diaphragmatic plication, and facilitated weaning from positive-pressure ventilation. These studies show that negative-pressure ventilation is a useful technique in selected patients after cardiac surgery where positive-pressure ventilation is not desirable or results in unwanted hemodynamic effects.
1. Dalziel J. On sleep and an apparatus for promoting artificial respiration. British Association for Advancement of Science. 1838;2:127-134.
2. Woillez E. Du spirophore, appareil de sauvetage pour le traitment de l’asphyxie, et principalement de l’asphyxie des noyes et de nouveaunes. Bulletin Academie Medicine (Paris). 1876;2:611-619.
3. Doe O.W. Apparatus for resuscitating asphyxiated children. Boston Medical Surgical Journal. 1889;120:9-17.
4. Drinker P., Shaw L.A. An apparatus for the prolonged administration of artificial respiration. J Clin Invest. 1929;7:229-247.
5. Silverman W.A., Sinclair J.C., MGG, Finster M. A controlled clinical trial of management of respiratory distress syndrome in a body-enclosing respirator. Pediatrics. 1967;39:740-748.
6. Fanaroff A.A., Cha C.C., Sosa R. Controlled clinical trial of continuous negative external pressure in the treatment of severe respiratory distress syndrome. J Pediatr. 1973;82:921-928.
7. Lassen H.C.A. A preliminary report on the 1952 epidemic of poliomyelitis in Copenhagen with special reference to the treatment of acute respiratory insufficiency. Lancet. 1953;i:37-41.
8. Barach A.L., Martin J., Eckman M. Positive-pressure respiration and its application to the treatment of acute pulmonary edema and respiratory obstruction. Proc Am Soc of Clin Invest. 1937;16:664-680.
9. Gregory G.A., Kitterman J.A., Phibbs R.H. Treatment of the idiopathic respiratory distress syndrome with continuous positive airway pressure. N Engl J Med. 1971;284:1333-1340.
10. Vidyasagar D., Chernick D. Continuous positive transpulmonary pressure in hyaline membrane disease — a simple device. Pediatrics. 1971;48:296-299.
11. Rideau Y., Janoski L.W., Grellet G. Respiratory function in the muscular dystrophies, Muscle Nerve. 1981;4:155-164.
12. Bach J.R., Alba A., Mosher, et al. Intermittent positive pressure ventilation via nasal access in the management of respiratory insufficiency. Chest. 1987;94:168-170.
13. Ferrer M., Esquinas A., Leon M., et al. Noninvasive ventilation in severe hypoxemic respiratory failure: a randomized clinical trial. Am J Respir Crit Care Med. 2003;168:1438-1444.
14. Honrubia T., Garcia Lopez F., Franco N., et al. Noninvasive vs conventional mechanical ventilation for acute respiratory failure. Chest. 2005;128:3916-3924.
15. Antonelli M., Conti G., Moro M.L., et al. Predictors of failures of noninvasive positive pressure ventilation in patients with acute hypoxemic respiratory failure: a multicenter study. Intensive Care Med. 2001;27:1718-1728.
16. Rasanen J., Heikkila J., Downs J., et al. Continuous positive pressure by facemask in acute cardiogenic pulmonary edema. Am J Cardiol. 1985;55:296-300.
17. Lin M., Chiang H.T. The efficacy of early continuous positive airway pressure therapy in patients with acute cardiogenic pulmonary edema. J Formos Med Assoc.. 1991;90:736-743.
18. Nava S., Carbone G., DiBattista N., et al. Noninvasive ventilation in cardiogenic pulmonary edema. A multicenter randomized trial. Am J Respir Crit Care Med. 2003;168:1432-1437.
19. Bott J., Carroll M.P., Conway J.H., et al. Randomized controlled trial of nasal ventilation in acute ventilatory failure due to chronic obstructive airways disease. Lancet. 1993;341:1555-1557.
20. Kramer N., Meyer T.J., Meharg J., et al. Randomized prospective trial of noninvasive positive pressure ventilation in acute respiratory failure. Am J Respir Crit Care Med. 1995;151:1799-1806.
21. Vitacca M., Clinic E., Rubini F., et al. Noninvasive mechanical ventilation in severe chronic obstructive lung disease and acute respiratory failure: Short term and long-term prognosis. Intensive Care med. 1996;22:94-100.
22. Meduri G.U., Cook T.R., Turner R.E., et al. Noninvasive positive pressure ventilation in status asthmaticus. Chest. 1996;110:767-774.
23. Fernandez M.M., Villagra A., Blanch L., et al. Noninvasive mechanical ventilation in status asthmaticus. Intensive Care Med. 2001;27:486-492.
24. Sorosky A., Stav D., Shpirer I. A pilot prospective, randomized, placebo-controlled trial of bi-level positive airway pressure in acute asthmatic attack. Chest. 2003;123:1018-1025.
25. Conti G., Marino P., Cogliati A., et al. Noninvasive ventilation for treatment of acute respiratory failure in patients with hematologic malignancies. A pilot study. Intensive Care Med. 1998;24:1283-1288.
26. Antonelli M., Conti G., Bufi M., et al. Noninvasive ventilation for treatment of acute respiratory failure in patients undergoing solid organ transplantation. A randomized trial. JAMA. 2000;283:235-241.
27. Hilbert G., Gruson D., Vargas F., et al. Noninvasive ventilation in immunosuppressed patients with pulmonary infiltrates, fever, and acute respiratory failure. New Engl J Med. 2001;344:481-487.
28. Matte P., Jacquet L., Van Dyck M., et al. Effects of conventional physiotherapy, continuous positive airway pressure and noninvasive ventilatory support with bi-level positive airway pressure after coronary artery bypass grafting. Acta Anaesthesiol Scan. 2000;44:75-81.
29. Joris J.L., Sottiaux T.M., Pons S., et al. Effect of bi-level positive airway pressure (BiPAP) nasal ventilation on the postoperative pulmonary restrictive syndrome in obese patients undergoing gastroplasty. Chest. 1997;111:665-670.
30. Auriant I., Jallot A., Herve P., et al. Noninvasive ventilation reduces mortality in acute respiratory failure following lung resection. Am J Respir Crit Care Med. 2001;164:1231-1235.
31. Udwadia Z.F., Santis G.K., Steven M.H., Simonds A.K. Nasal ventilation o facilitate weaning in patients with chronic respiratory insufficiency. Thorax. 1992;47:715-718.
32. Nava S., Ambrosino N., Clini E. Noninvasive mechanical ventilation in the weaning of patients with respiratory failure due to chronic obstructive pulmonary disease. A randomized, controlled trial. Ann Intern Med. 1998;128:721-728.
33. Girault C., Daudenthun I., Chevron V. Noninvasive ventilation as a systematic extubation and weaning technique in acute-on-chronic respiratory failure: a prospective, randomized controlled study. Am J Respir Crit Care Med. 1999;160:86-92.
34. Ferrer M., Esquinas A., Leon M. Noninvasive ventilation in severe hypoxemic respiratory failure: a randomized clinical trial. Am J Respir Crit Care Med. 2003;168:1438-1444.
35. Esteban A., Frutos-Vivar F., Ferguson N.D., Noninvasive positive pressure ventilation for respiratory failure after extubation, N Engl J Med 2004:350;2462–2460
36. Sullivan C.E., Issa F.G., Jones M.B., Eves L. Reversal of obstructive sleep apnea by continuous positive airway pressure applied through the nares. Lancet. 1981;1:862-865.
37. Guilleminault C., Nino-Murcia G., Heldt G. Alternative treatment to tracheostomy in obstructive sleep apnea syndrome: nasal continuous positive airway pressure in young children. Pediatrics. 1986;78:797-802.
38. Waters K.A., Everett F.M., Bruderer J.W., Sullivan C.E. Obstructive sleep apnea: the use of nasal CPAP in 80 children. Am J Resp Crit Care Med. 1995;152:780-785.
39. Berry R.B., Block A.J. Positive nasal airway pressure eliminates snoring as well as obstructive sleep apnea. Chest. 1984;85:15-20.
40. Brooks L.J. Treatment of otherwise normal children with obstructive sleep apnea. Ear, Nose, Throat J. 1993;72:77-79.
41. Rains J.C. Treatment of obstructive sleep apnea in pediatric patients. Behavioral intervention for compliance with nasal continuous positive airway pressure. Clin Pediatr. 1995;34:535-541.
42. Zwacka G., Scholle S. Experiences with therapy of pediatric sleep apnea syndrome and obstructive nasopharyngeal respiratory pattern with nasal BIPAP and CPAP therapy [in German]. Pneumologie. 1995;49:152-154.
43. Guilleminault C., Pelayo R., Clerk A. Home nasal continuous positive airway pressure in infants with sleep-disordered breathing. J Pediatr. 1995;127:905-912.
44. Akingbola O.A., Servant G.M., Custer J.R., Palmisano J.M. Noninvasive bi-level positive pressure ventilation: management of two pediatric patients. Respir Care. 1993;38:1092-1098.
45. Akingbola O., Palmisano J., Servant G. Bi-PAP mask ventilation in pediatric patients with acute respiratory failure. Crit Care Med. 1994;22:144.
46. Marino P., Rosa G., Conti G., Cogliati A.A. Treatment of acute respiratory failure by prolonged non-invasive ventilation in a child. Can J Anesth. 1997;44:727-731.
47. Fortenberry J.D., Del Toro J., Jefferson L.S. Management of pediatric acute hypoxemic respiratory insufficiency with bi-level positive pressure (BiPAP) nasal mask ventilation. Chest. 1995;108:1059-1064.
48. Rosen G.M., Muckle R.P., Mahowald M.W. Postoperative respiratory compromise in children with obstructive sleep apnea syndrome: can it be anticipated? Pediatrics. 1994;93:784-788.
49. Teague W.G., Lowe E., Dominick J., Lang D. Non-invasive positive pressure ventilation (NPPV) in critically ill children with status asthmaticus. Am J Respir Crit Care Med. 1998;157:542.
50. Padman R., Lawless S.T., Kettrick R.G. Non-invasive ventilation via bi-level positive airway pressure support in pediatric practice. Crit Care Med. 1998;26:169-173.
51. Birnkrant D.J., Pope J.F., Eiben R.M. Pediatric noninvasive nasal ventilation. J Child Neurol. 1997;12:231-236.
52. Padman R., Lawless S., Von Nessen S. Use of BiPAP by nasal mask in the treatment of respiratory insufficiency in pediatric patients: preliminary investigation. Pediatr Pulmonol. 1994;17:119-123.
53. Teague W.G., Kervin L., Dawadkar V., Scott P. Nasal bi-level positive airway pressure acutely improves ventilation and oxygen saturation in children with upper airway obstruction. Am Rev Respir Dis. 1991;143:505.
54. Yan L.J., Yunge M., Emilfork M., et al. A prospective, randomized, controlled trial of noninvasive ventilation in pediatric acute respiratory failure. Pediatr Crit Care Med. 2008;9:484-489.
55. Thill P.J., McGuire J.K., Baden H.P., et al. Noninvasive positive-pressure ventilation in children with lower airway obstruction. Pediatr Crit Care Med. 2004;5:337-342.
56. Pancera C.F., Hayashi M., Fregnani J.H., et al. Noninvasive ventilation in immunocompromised pediatric patients: eight years of experience in a pediatric oncology intensive care unit. J Pediatr Hematol Oncol. 2008;30:533-538.
57. Bach J.R., Sabharwal S. High pulmonary risk scoliosis surgery: role of noninvasive ventilation and related techniques. J Spinal Disord Tech. 2005;18:527-530.
58. Scala R., Naldi M. ventilators for noninvasive ventilation to treat acute respiratory failure. Respir Care. 2008;53:1054-1080.
59. White GC: Equipment theory for respiratory care, ed 4, Thomson Delmar Learning, pp 583–660.
60. Smith IE, Shneerson JM: A laboratory comparison of four positive pressure ventilators used in the home, Eur Respir J 9(11):2410–2415, 1996.
61. Kacmarek R.M., Hill N.S. Ventilators for noninvasive positive pressure ventilation: technical aspects. Eur Respir Mon. 2001;16:76-101.
62. Chatmongkolchart S., Williams P., Hess D.R., Kacmarek R.M. Evaluation of inspiratory rise time and inspiration termination criteria in new-generation mechanical ventilators: a lung model study. Respir Care. 2001;46:666-677.
63. Vignaux L., Tassaux D., Jolliet P. performance of noninvasive ventilation modes on ICU ventilators during pressure support: A bench model study. Intensive Care Med. 2007;33:1444-1451.
64. Lofaso F., Brochard L., Thierry H. Home versus intensive care pressure support devices. Experimental and clinical comparison. Am J Crit Care Med. 1996;153:1591-1599.
65. Kacmarek R.M., Hill N.S. Ventilators for noninvasive positive pressure ventilation: technical aspects. Eur Respir Mon. 2001;16:76-105.
66. Leger P., Jennequin J., Gerard M., Robert D. Home positive pressure ventilation via nasal masks in patients with neuromuscular weakness and restrictive lung or chest wall disease. Respir Care. 1989;34:73-79.
67. Perrin C., D’Ambrosio C., White A., Hill N.S. Sleep in restrictive and neuromuscular respiratory disorders. Semin Respir Crit Care Med. 2005;26:117-130.
68. Hill N.S., Redline S., Carskadon M.A., et al. Sleep-disordered breathing in patients with Duchenne muscular dystrophy using negative pressure ventilators. Chest. 1992;102:1656-1662.
69. Masa Jimenez J.F., deCos Escuin J.S., Vicente C.D., et al. Nasal intermittent positive pressure ventilation. Analysis of withdrawal. Chest. 1995;107:382-388.
70. Young H.K., Lowe A., Fitzgerald D.A., et al. Outcome of noninvasive ventilation in children with neuromuscular disease, Neurology. 2007;68:198-201.
71. Nauffal D., Domenech R., Martinez Garcia M.A., et al. Noninvasive positive pressure home ventilation in restrictive disorders. Outcome and impact on health-related quality of life. Respir Med. 2002;96:777-783.
72. Petrone A., Pavone M., Chiarini Testa M.B. Noninvasive ventilation in children with spinal muscular atrophy types 1 and 2. Am J Phys Med Rehabil. 2007;86:216-221.
73. Gomez-Merino E., Bach J.R. Duchenne muscular dystrophy: Prolongation of life by noninvasive ventilation and mechanically assisted coughing. Am J Phys Med Rehabil. 2002;81:411-415.
74. Stahlman M.J., Young W.C., Gray J. The management of respiratory failure in the idiopathic respiratory distress syndrome of prematurity. Ann N Y Acad Sci. 1965;121:930-941.
75. Bancalari E., Gerhardt T., Monkus E. Simple device for producing continuous negative pressure in infants with IRDS. Pediatrics. 1973;52:128-131.
76. Outerbridge E.W., Roloff D.W., Stern L. Continuous negative pressure in the management of severe respiratory distress syndrome. J Pediatr. 1972;81:384-391.
77. Alexander G., Gerhardt T., Bancalari E. Hyaline membrane disease. Comparison of continuous negative pressure and nasal positive airway pressure in its treatment. Am J Dis Child. 1979;133:1156-1159.
78. Samuels M.P., Raine J., Wright T., et al. Continuous negative extrathoracic pressure in neonatal respiratory failure. Pediatrics. 1996;98:1154-1160.
79. Brahdy M.B., Lenarsky M. Respiratory failure in acute epidemic poliomyelitis. J Pediatr. 1936;8:420-433.
80. Crone N.L. The treatment of acute poliomyelitis with the respirator. N Engl J Med. 1934;210:621-623.
81. Wilson J.L. Acute anterior poliomyelitis. N Engl J Med. 1932;206:887-893.
82. Splaingard M.L., Frates R.C.Jr., Jefferson L.S. Home negative pressure ventilation: report of 20 years of experience in patients with neuromuscular disease. Arch Phys Med Rehabil. 1985;66:239-242.
83. Goldstein R.S., Stradling J.R. An artifact induced by negative pressure ventilation. Chest. 1992;101:563-565.
84. Sanyal S.K., Bernal R., Hughes W.T., Feldman S. Continuous negative chest-wall pressure. Successful use for severe respiratory distress in an adult. JAMA. 1976;236:1727-1728.
85. Sawicka E.H., Spencer G.T., Branthwaite M.A. Management of respiratory failure complicating pregnancy in severe kyphoscoliosis: a new use for an old technique? Br J Dis Chest. 1986;80:191-196.
86. Morris A.H., Elliott C.G. Adult respiratory distress syndrome: successful support with continuous negative extrathoracic pressure. Crit Care Med. 1985;13:989-990.
87. Hartke R.H.Jr., Block A.J. External stabilization of flail chest using continuous negative extrathoracic pressure. Chest. 1992;102:1283-1285.
88. Sanyal S.K., MacGaw D., Hughes W.T. Continuous negative chest-wall pressure as therapy for severe respiratory distress in an older child: preliminary observations. J Pediatr. 1974;85:230-232.
89. Sanyal S.K., Mitchell C., Hughes W.T. Continuous negative chest-wall pressure as therapy for severe respiratory distress in older children. Chest. 1975;68:143-148.
90. Sanyal S.K., Avery T.L., Thapar M.K. Continuous negative chest-wall pressure therapy for assisting ventilation in older children with progressive respiratory insufficiency. Acta Paediatrica Scandinavica. 1977;66:451-456.
91. Raine J., Redington A.N., Benatar A. Continuous negative extrathoracic pressure and cardiac output–a pilot study. Eur J Pediatr. 1993;152:595-598.
92. Shapiro S.H., Ernst P., Gray-Donald K., et al. Effect of negative pressure ventilation in severe chronic obstructive pulmonary disease. Lancet. 1992;340:1425-1429.
93. Shekerdemian L.S., Bush A., Shore D.F. Cardiopulmonary interactions after Fontan operations: augmentation of cardiac output using negative pressure ventilation. Circulation. 1997;96:3934-3942.
94. Shekerdemian L.S., Bush A., Lincoln C. Cardiopulmonary interactions in healthy children and children after simple cardiac surgery: the effects of positive and negative pressure ventilation. Heart. 1997;78:587-593.
95. Raine J., Samuels M.P., Mok Q. Negative extrathoracic pressure ventilation for phrenic nerve palsy after paediatric cardiac surgery. Br Heart J. 1992;67:308-311.