CHAPTER 64 Non-surgical ultrasonic lipoplasty
Physical evaluation
• The safety profile for the procedure is excellent, with no abnormal findings in blood analyses and with very few side effects/complications, none of which are significant.
• The procedure is extremely precise. The product is designed so that energy is delivered only to the target tissue, with no effect to the skin or to the non treated surrounding tissues or organs.
• The procedure is non-surgical and ultimately results in reduction of the targeted fat layer with minimal, if any, risk of infection.
• Speed and efficiency for the procedure are impressive. The learning curve for product operation is short. The treatments take well under an hour to complete.
• The procedure is well-tolerated by patients. There is no general anesthesia required. There is minimal post-treatment discomfort and essentially no patient down-time.
• Clinical efficacy is quite promising as the early results are visibly evident upon viewing before and after clinical photographs.
• Patient satisfaction is high, as most patients experience a noticeable difference in fit of their clothes and indicate that they would recommend the procedure to others.
Anatomy
Each adipocyte has a thin rim of cytoplasm which contains a flat nucleus, a small amount of cytoplasm, and in general, one large lipid droplet. Up to 75% of the cell volume is made up of lipids or triglycerides. The remaining weight of the adipocyte is protein and water. Each triglyceride droplet is comprised of one glycerol and three fatty acid molecules. The fatty acid composition changes depending on factors such as age, diet and exercise.
Technical steps
The procedure itself is straightforward. The treatment head is moved across the treatment area while the device is activated using a footswitch, similar to many commercial aesthetic laser products. Figure 64.1 shows the device, and Figure 64.2 illustrates the treatment head positioned for use on the abdomen of a patient. A LipoSonix® procedure can normally be completed in less than one hour. At the end, patients usually are ambulatory and can resume normal activities that same day.
The technology works by precisely focusing HIFU energy into target areas of adipose tissue to cause controlled, localized cell disruption. The ultrasound transducer incorporated into the treatment head delivers energy across the skin surface at a relatively low intensity and therefore there is no damage superficial to the treatment. The specific geometry of the transducer and transducer positioning method allows for focusing of the ultrasound energy at variable depths from 10–20 mm beneath the skin. This focusing of the beam results in precise high intensity energy delivery to the target zones within the adipose tissue. Figure 64.3 illustrates this concept.
Once adipocytes have been disrupted, macrophage cells are attracted via chemotactic signals to phagocytose the lipids and cell debris. Figure 64.4 shows a typical histology section of a treated area (two weeks post-procedure) using Masson’s trichrome stain. Macrophages containing lipid droplets are clearly visible. The macrophages migrate to the lymph nodes and on to the liver, where the lipids are processed through the body’s normal biochemical pathways. Our research shows that the majority of treated tissue is resorbed over an 8–12 week period. The resulting reduction in adipose tissue volume leads to an observable aesthetic effect, as evidenced by quantitative measurements and by clinical photography.
Aesthetic results
Figures 64.5 and 64.6 show representative pre-procedure and 3-month post-procedure aesthetic photos of a female and male patient, respectively. Patients will typically see peak response, both in visible contour change and reduction in circumferential measurement, between 8 and 12 weeks post-procedure.
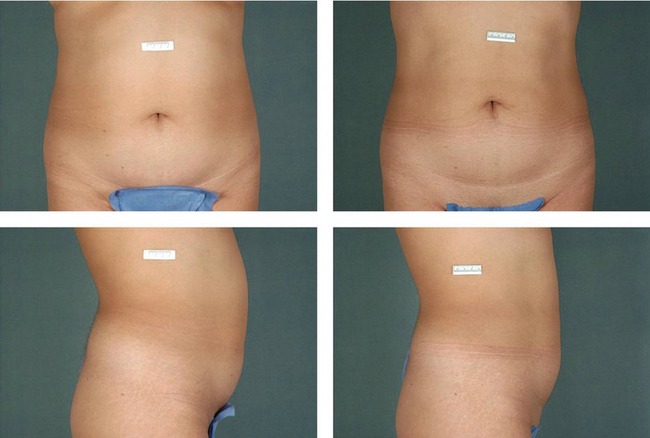
Fig. 64.5 Representative pre-procedure and 3 month post-procedure aesthetic photos of a female patient.
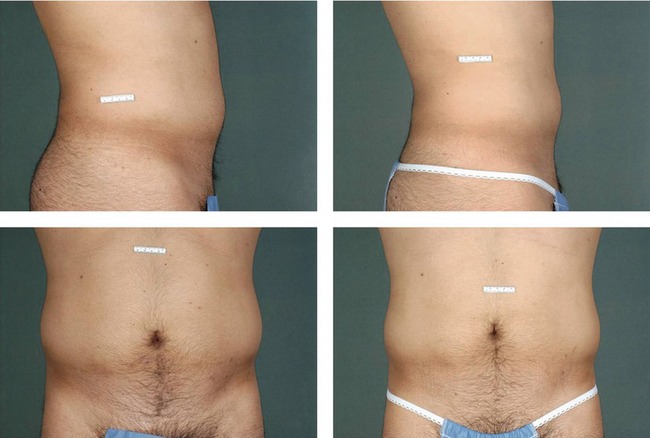
Fig. 64.6 Representative pre-procedure and 3 month post-procedure aesthetic photos of a male patient.
Pearls & pitfalls
Pearls
• As with lipoplasty, patient selection is key. It is not a remedy for obesity. The ideal candidates are in good health, with good skin elasticity, and with specific localized adipose deposits that have been resistant to diet and exercise.
• Be sure to properly manage patient expectations – results are not immediate and are not as dramatic as with the more invasive lipoplasty procedures.
• Take your time during the planning stage to accurately place the treatment grid. This will save time during the actual treatment.
• Optimize patient position and the placement of the LipoSonix® device to facilitate good operator posture and ergonomics during the application. This will result in a more comfortable treatment session.
Pitfalls
• Do not treat patients with less than 2.5 cm of adipose tissue in depth at the treatment site. So doing could result in inadvertent application of energy to the muscle layer below the adipose tissue.
• Do not press down too aggressively with the treatment head as it could compress the subjacent adipose layer. Apply light, uniform pressure.
• Use liberal amounts of coupling fluid during the procedure to keep the patient comfortable and avoid damage to the treatment head.
• Avoid treating bony areas, the umbilicus, or scars.
• Keep the treatment head parallel to the skin as much as possible to ensure an even energy delivery.
Adams WM, Higgins PD, Siegfried L, et al. Chronic response of normal porcine fat and muscle to focused ultrasound hyperthermia. Radiat Res. 1985;104:140–152.
Fodor PB, Hedrick MH. Discussion: Comparative lipoplasty analysis of in vivo-treated adipose tissue. Plast Reconstr Surg. 2000;105:2159–2160.
Fodor PB, Watson J. Personal experience with ultrasound-assisted lipoplasty: A pilot study comparing ultrasound-assisted lipoplasty with traditional lipoplasty. Plast Reconstr Surg. 1998;101:1103–1116. discussion 1117–1119
Garcia-Murray E, Adan Rivas OE, Stecco KA, et al. Evaluation of the acute and chronic systemic and metabolic effects from the use of high intensity focused ultrasound for adipose tissue removal and non-invasive body sculpting. Plast Reconstr Surg. 2005;116(3):151–152.
Garcia-Murray E, Adan Rivas OE, Stecco KA, et al. The use and mechanism of action of high intensity focused ultrasound for adipose tissue removal and non-invasive body sculpting. Plast Reconstr Surg. 2005;116(3):222–223.
Kenkel JM, Robinson JB, Jr., Beran SJ, et al. The tissue effects of ultrasound-assisted lipoplasty. Plast Reconstr Surg. 1998;102(1):213–220.
Linke CA, Carstensen EL, Frizzell LA, Elbadawi A, Fridd CW. Localized tissue destruction by high-intensity focused ultrasound. Arch Surg. 1973;107(6):887–891.
Lynn J, Zwemer R, Chick A, et al. A new method for generation and use of focused ultrasound in experimental biology. J Gen Physiol. 1942;26:179–193.
Rohrich RJ, Morales DE, Krueger JE, et al. Comparative lipoplasty analysis of in vivo-treated adipose tissue. Plast Reconstr Surg. 2000;105(6):2152–2158.
Scuderi N, DeVita R, d’Andrea F, Vonella M. Nuove prospective nella liposuzione: La lipoemulsificazione. Giorn Chir Plast Riconstr Estet. 1987;2:1.
ter Haar G, Sinnett D, Rivens I. High intensity focused ultrasound – a surgical technique for the treatment of discrete liver tumours. Phys Med Biol. 1989;34(11):1743–1750.