Neurologic, muscular, and sensory systems
Maternal physiologic adaptations
During pregnancy the neurologic, muscular, and sensory systems are influenced by the altered hormonal milieu and by alterations in other systems. The effects of these changes on the muscular and sensory systems are well documented. Many of the hormones of pregnancy also have CNS activity, although their specific effects on neurologic function are not as well understood as their effects on other body systems. As a result, there is little known about specific changes in the function of the neurologic system during pregnancy, other than effects on endocrine glands (see Chapter 19). During the intrapartum period, maternal physiologic and psychologic responses are altered by the discomfort and pain of labor. These responses can have a significant impact on fetal homeostasis in addition to having important implications for management of the woman in labor.
Antepartum period
Ocular changes
The eyes of the pregnant woman undergo several alterations during pregnancy that result from physiologic and hormonal adaptations. The pregnant woman develops a mild corneal edema, particularly during the third trimester. The cornea becomes slightly thicker, which (along with the fluid retention) changes its topography and may slightly alter the refractory power of the eye. Corneal hyposensitivity may sometimes also develops during this period, probably because of the increased thickness and fluid retention.172,182,217,238 A few women experience an increase in corneal epithelial pigmentation (known as Krukenberg spindles), possibly secondary to increases in estrogens, progesterone, adrenocorticotropic hormone (ACTH), and melanocyte-stimulating hormone.45,182 The composition of tears changes slightly with an increase in secretion of lysozyme.172 Disruption of lacrimal acinar cells can lead to dry eyes.182
Most studies report that intraocular pressure falls about 10%, especially during the second half of gestation (after 28 weeks), although variations are seen among women in the degree of change.45,54,77,182,196,217 This change is believed to be due to the effects of progesterone, relaxin, and human chorionic gonadotropin combined with an increase in aqueous outflow and decreased episcleral pressure.45,224,238 Similar findings have been reported for women on oral contraceptives. The decrease in intraocular pressure is greater in multiparas than primiparas.196 Changes in intraocular pressure are independent of changes in systemic blood pressure.77 An increase in intraocular pressure has been reported in women with preeclampsia. This increase is not correlated with blood pressure, but may be due to increased extracellular fluid volume and decreased aqueous humor outflow in the preeclamptic woman.77
Ptosis, usually unilateral, occasionally develops for unknown reasons, but may be related to changes in the levator aponeurosis due to hormonal and fluid changes or stress.172,182,238 Ptosis may also occur as a complication of lumbar anesthesia, either as an isolated finding or as part of Horner syndrome. Horner syndrome is characterized by ptosis, miosis, and anhidrosis secondary to interruption of sympathetic innervation.238 Some women experience an increased pigmentation of the face and eyelids known as melasma (see Chapter 14).
During pregnancy there is a progressive decrease in blood flow to the conjunctiva, which is sensitive to estrogen. This change is most marked in women with preeclampsia as a result of spasm and ischemia.11 In these women, changes in the conjunctival vessels may occur earlier than in the retinal vessels. Subconjunctival hemorrhages may occur spontaneously during pregnancy or in labor, with spontaneous resolution.
Otolaryngeal changes
Changes in the ear, nose, and larynx are related to modifications in fluid dynamics and vascular permeability, increased protein synthesis, vasomotor alterations of the autonomic nervous system, and increased vascularity, along with hormonal (especially estrogen) influences.65,135,208,215 As a result of these alterations, the nasal mucosa becomes congested and hyperemic. The pregnant woman experiences nasal stuffiness and obstruction associated with serous rhinorrhea or postnasal discharge. Altered olfactory perception with increased sensation to smell has also been reported.35,129
Pregnancy rhinitis (defined as nasal congestion that lasts 6 or more weeks without signs of infection or allergy) is seen in up to 30% of all pregnant women and in approximately two thirds of those who smoke.65,129,183 These symptoms may appear anytime but usually begin in the second trimester and parallel increasing estrogen levels.65,195,242 The symptoms may interfere with sleep and the sense of smell. The cause is unknown, but placental growth hormone has been implicated as a stimulator of nasal mucosal growth.65 The rhinitis may also be due to increased sensitivity to allergens in women with subclinical allergy.129 Vascular congestion may result in epistaxis from rupture of superficial blood vessels. External nasal alar dilators or nasal washings with a saline solution may provide some relief.65 Systemic antihistamines should be used with caution and only for short periods. Pseudoephedrine should be avoided, especially in the first trimester due to a reported increase in the risk of gastroschisis.215 Prolonged use (over 3 to 5 days) of topical sympathomimetic nasal sprays should be avoided because they can cause rebound congestion.65,135,195,242
The pregnant woman may also complain of ear stuffiness or blocked ears unrelieved by swallowing. This change is believed to result from estrogen-induced changes in mucous membranes of the eustachian tube, edema of the nasopharynx, and alterations in fluid dynamics and pressures of the middle ear.129,135,195,242 Some women may experience a transient, mild hearing loss, and an increased risk of serous effusion. Management is usually supportive. Increased estrogen and progesterone leads to transient vertigo in some women.129,216,242 Tinnitus is more common during pregnancy, possibly due to hyperdynamic circulation, increased peripheral lymphatic fluid, and hormonal changes.129,216 Symptoms resolve with delivery. Ménière’s disease may be exacerbated during pregnancy because of fluid retention.242
Laryngeal changes during pregnancy are hormonally induced and include erythema and edema of the vocal cords accompanied by vascular dilation and small submucosal hemorrhages.139,195,242 The woman may note hoarseness, deepening or cracking of the voice, persistent cough, or other vocal changes. Some women report the quality of their voice improves during the first two trimesters due to better lubrication of the vocal cords.94 Laryngeal changes can complicate administration of endotracheal anesthesia. Similar changes are reported in women premenstrually and with use of progesterone-dominated oral contraceptives.195 Maximal phonation time decreases and vocal fatigue is more prevalent during pregnancy, possibly due to the decreased thoracic cage volume with uterine growth.94 The airway becomes hyperemic with mucosal edema and a decreased diameter of the upper airway, which may increase snoring and sleep disorders.29,75 The edema arises from the increased plasma volume and capillary congestion.29,139
Musculoskeletal changes
Pregnancy is characterized by changes in posture and gait. Relaxin and progesterone affect the cartilage and connective tissue of the sacroiliac joints and the symphysis pubis. This, along with external rotation of the femurs, increases the mobility of these joints and leads to the characteristic “waddle” gait seen in many pregnant women.71,116,165 Widening and increased mobility of the sacroiliac synchondroses and symphysis pubis begins by 10 to 12 weeks.103 The symphysis pubis may widen up to 10 mm.26,116
Musculoskeletal changes during pregnancy may also be due to changes in gait to compensate for the increase in and redistribution of body mass.71 The altered gait in pregnancy may increase the load on the lateral side of the foot and hindfoot, leading to lower limb pain. Ligamentous laxity increases due primarily to the effects of estrogen.26,203 Estrogen and progesterone receptors on fibroblasts within the walls of the blood vessels supplying the anterior cruciate ligaments increase during pregnancy, with decreased collagen synthesis and increased relaxin. Relaxin remodels collagen fibers and reduces their diameters.26 The risk of ligament injury is increased during pregnancy.116 Muscle cramps are more frequent in pregnant women, especially during the second half of pregnancy and at night (see Chapter 17).105,116
Pregnant women often experience back pain and other discomforts (see section on Musculoskeletal Discomforts). Distention of the abdomen with growth of the fetus tilts the pelvis forward, shifting the center of gravity. The woman compensates by developing an increased curvature (lordosis) of the spine that may strain muscles and ligaments of the back. Stretching and decreased tone of the abdominal muscles also contribute to the lordosis. Diastasis of the rectus abdominis muscles in the third trimester may persist after delivery. Breast tenderness, heaviness, tingling, and occasionally pain occur by 6 weeks. These changes are due to estrogens, progesterone, human placental lactogen (hPL), increased blood volume, and venous stasis. Long-term bed rest can lead to muscle atrophy and change gastrocnemius muscle metabolism.20,154 Muscle recovery postpartum can take more than six weeks.20,154
Sleep
Sleep patterns are altered during pregnancy and the postpartum period. Sleep quality and insomnia tend to be most prominent in the first and third trimesters.59,106,123,137,212,218 With advancing gestation total sleep hours progressively decrease.123 Sleep time also decreases prior to labor onset with an increase in night awakenings reported beginning about 5 days prior to labor onset.17 Both hormonal and mechanical factors alter the pregnant woman’s sleep-wake patterns (Figure 15-1).209,212 Sleep is divided into rapid eye movement (REM) and non–rapid eye movement (NREM) or deep sleep, which is subdivided into four stages. Hormonal changes alter sleep during pregnancy. Progesterone has a sedative effect and increases NREM sleep.209,212 Estrogens and cortisol decrease REM, whereas prolactin increases both REM and NREM sleep.61,212 Changes in sleep stages are seen by 11 to 12 weeks.139 In general, pregnant women tend to have less deep and lighter sleep and more awakenings (from less than 5% to 10% by the third trimester).139
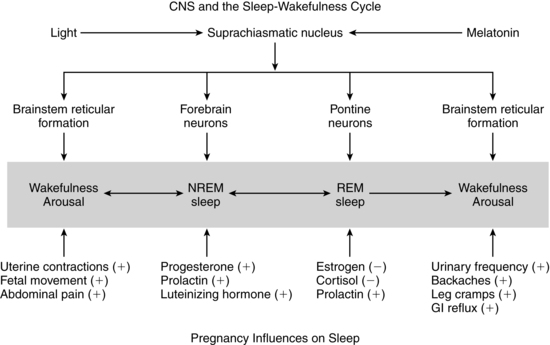
During the first trimester, total sleep time increases, as does napping. By the second half of gestation, pregnant women have less overall sleep time and more night awakenings than nonpregnant women do. The pregnant woman has decreased REM sleep in the third trimester, along with increased awakenings and napping, and diminished alertness during the day.59,104,106,137,212 Early-onset REM sleep is reported in pregnant women with depression or mood disorders.139 Alterations in NREM sleep with an increase in stage 1 (sleep latency or transition between wakefulness and sleep) and a decrease in stages 2 and 4 (delta sleep or deep sleep stage) during late pregnancy have also been noted.122,137 The decrease in stage 4 NREM sleep has implications for the pregnant woman’s functioning, because this stage is important for basic biologic processes such as tissue repair and recovery from fatigue.140 In summary, changes to sleep reported during the first trimester include increased total sleep, napping, daytime sleepiness and insomnia, and decreased REM, and stage 3 and stage 4 NREM sleep; changes during the second trimester included increased awakenings with normal total sleep times and decreased REM, stage 3 NREM, and stage 4 NREM sleep; third trimester changes included increased daytime sleepiness, insomnia, nocturnal awakenings, and stage 1 NREM sleep, and decreased REM, stage 3 NREM, and stage 4 NREM sleep.59.106,122,137,212,218,236 During pregnancy, night awakenings are often associated with nocturia, dyspnea, heartburn, uterine activity, nasal congestion, muscle aches, stress, and anxiety and can lead to sleep disturbances and insomnia. The major reasons given by women for sleep alterations include urinary frequency, backache, leg cramps, restless legs, heartburn, and fetal activity.61,104,139,236 Interventions include establishing regular sleep-wake habits and periods, avoiding caffeine, relaxation techniques, massage, heat and support for lower back pain, modifying the sleep environment, and limiting fluids in the evening.212 Sleep medications should be avoided because these drugs alter the physiologic mechanisms of sleep by suppressing REM and NREM stages 3 and 4 and may cross the placenta to the fetus. Sleep loss (i.e., sleeping less than 6 hours per night) in the last 3 to 4 weeks of pregnancy may increase labor length and need for cesarean birth.76,94,138,259
A pregnancy-associated sleep disorder has been described by the American Sleep Disorders Association.9 Individuals with sleep disorder breathing may have an increase in severity during pregnancy due to partial airway obstruction by otolaryngeal changes.29,208,209 Increases are also reported in snoring and possibly obstructive sleep apnea (OSA), although the prevalence of OSA during pregnancy is not well documented.29,75,208,209 The prevalence of snoring during pregnancy is 15% to 30% by the third trimester.139 An increase in hypertension, preeclampsia, gestational diabetes, and fetal growth restriction has been reported in pregnant women who snore.28,29,75,168
Intrapartum period
Pain and discomfort during labor
The woman in labor experiences two types of pain: visceral and somatic. Visceral pain is related to contraction of the uterus and dilation and stretching of the cervix. Uterine pain during the first stage of labor results from ischemia caused by constriction and contraction of the arteries supplying the myometrium. Somatic pain is caused by pressure of the presenting part on the birth canal, vulva, and perineum. Visceral pain is experienced primarily during the first stage of labor; somatic pain is experienced during transition and the second stage.68 Somatic pain is more intense and localized.
The corpus of the uterus is relatively denervated by late pregnancy while the cervix remains densely innervated.240 This suggests that the cervical area maybe the major site of pain in labor.240 Near term numbers of nerve cells and fibers in the sensory area of the spinal column decrease accompanied by increased excitability of mechanosensitive efferents in the cervix.125,181 The pain threshold may be altered in late pregnancy, enhanced by elevated β-endorphins, leading to a proposed “pregnancy-induced hypoanalgesia” (see next section).79,125,181
Pain from uterine contractions and dilation of the cervix during the first stage of labor is transmitted by afferent fibers to the sympathetic chain of the posterior spinal cord at T10 to T12, and L1. In early labor, pain is transmitted primarily to T11 to T12 (Figure 15-2).68,79,206 As activation of peripheral small A-delta (myelinated) and C afferent (unmyelinated) nerve fibers of these nerve terminals (by kinin-like substances released from the uterine and cervical tissues) intensifies, transmission spreads to T10 and L1. Pain during the first stage may be referred; that is, the nerve impulses from the uterus and cervix stimulate spinal cord neurons, innervating both the uterus and the abdominal wall. As a result, the woman experiences pain over the abdominal wall between the umbilicus and symphysis pubis, around the iliac crests to the gluteal area, radiating down the thighs, and in the lumbar and sacral regions.79,231 During transition and the second stage, somatic pain impulses from distention of the birth canal, vulva, and perineum by the presenting part are transmitted by the pudendal nerves through the posterior roots of the parasympathetic chain at S2, S3, and S4.41,79
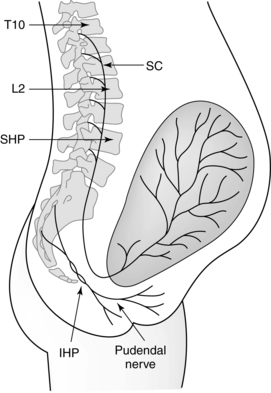
Peripheral tissue injury and inflammation change the transcription and function of channels and receptors in the peripheral and central nervous systems.102,240 Inflammation (mediated by prostaglandins, cytokines, granulocytes, integrins, and metalloproteases) activates nociceptive nerve fibers, decreasing their threshold and increasing action potentials.102,127 This visceral hypersensitivity is mediated by ion- and acid-sensitive channels, and by prostaglandin E2, N-methyl-d-aspartate (NMDA), and other receptors.102 Stimulation of dorsal root NMDA receptors mediates central hypersensitivity.127
As the A-delta and C nerve fibers enter the dorsal horn of the spinal cord, they synapse and ascend to the brainstem by the spinothalamic tract (see Figure 15-2). The pain impulses entering the brain stimulate a variety of neurons, including cortical neurons and those of the brainstem reticular formation (integrative function), thalamus, hypothalamus, and limbic system.206 The result is a conscious sensation of pain as well as a variety of ventilatory, circulatory, and metabolic responses. Spatial and temporal summation is processed in the cortex.151,206 These responses include increases in ventilation, cardiac output, peripheral resistance, gastric acid secretion, metabolic rate, oxygen consumption, and catecholamine release.
The perception of pain is influenced by physiologic, psychologic, and cultural factors. Pain can lead to anxiety and influence maternal physiologic responses and the course of labor. For example, physical manifestations of anxiety may include muscular tension, hyperventilation, increased sympathetic activity, and norepinephrine release, which can lead to increased cardiac output, blood pressure, metabolic rate, and oxygen consumption and impaired uterine contractility (Figure 15-3).18,119,205 Anxiety can also increase fear and tension, reducing pain tolerance, which decreases uterine contractility.18,119,151 Relaxation techniques such as progressive muscle relaxation, touch, breathing, imagery, and autosuggestion help reduce anxiety and prevent or stop this cycle.119
Descending spinal tracts in the dorsal horn modulate nociception via input from the cortex and limbic system with release of endogenous opioids such as β-endorphins and enkephalins (see next section).205 These modulating factors are produced by the placenta as well as the mother and may include an opioid-enhancing factor.205 Other modulating factors include analgesia induced by mechanical stimulation of the hypogastric (uterine mechanical stimulation) and pelvic (vaginal distention) nerves.206 Exogenous modification of labor pain includes both pharmacologic (opioids, sedatives, analgesics, anesthetics) interventions and nonpharmacologic cognitive, behavioral, and sensory techniques.70,156 These techniques include relaxation, cognitive and behavioral childbirth preparation, hypnosis, acupuncture, movement, positioning, vocalizations, touch, massage, music, biofeedback, transcutaneous electrical nerve stimulation (TENS), and hydrotherapy (Table 15-1).13,15,18,41,62,70,113,119,206,225,227,229
Table 15-1
Proposed Mechanisms of Labor Pain Reduction with Various Nonpharmacologic Measures
MECHANISMS | ACUPUNCTURE/ACUPRESSURE | AROMATHERAPY | BREATHING/FOCUS | CHILDBIRTH EDUCATION | COLD | EMOTIONAL SUPPORT | HEAT | HYDROTHERAPY | HYPNOSIS | INTRADERMAL WATER BLOCK | MASSAGE/TOUCH | MOVEMENT AND POSITIONING | MUSIC/AUDIOANALGESIA | RELAXATION | TENS |
Counterirritation analgesia (brief intense stimulation of trigger points) | X | X | X | ||||||||||||
Increases endorphins | X | X | X | X | |||||||||||
Provides stimuli from peripheral sensory receptors to inhibit pain awareness | X | X | X | X | X | X | X | ||||||||
Increases joint mobility | X | X | X | ||||||||||||
Alters pressures within pelvis and on soft tissues | X | X | X | ||||||||||||
Improves energy flow along meridians crucial to labor progress and comfort | X | X | |||||||||||||
Decreases muscle tension | X | X | X | X | X | X | X | ||||||||
Alters nerve conduction velocity (slows pain transmission to CNS) | X | ||||||||||||||
Decreases anxiety/fear, provides reassurance | X | X | X | X | X | X | X | X | X | ||||||
Increases woman’s sense of control, reducing pain perception | X | X | X | X | X | X | X | X | |||||||
Distraction of attention from pain | X | X | X | X | X | X | X | X | X | X | X | ||||
Enhances or changes mood, reducing pain perception | X | X | X | X | X | X | |||||||||
Cues rhythmic activity and rituals | X | X | X | X | X |
CNS, Central nervous system; TENS, transcutaneous electrical nerve stimulation.
From Simkin, P. & Bolding, A. (2004). Update on nonpharmacologic approaches to relieve labor pain and prevent suffering. J Midwifery Womens Health, 49, 491.
“The theory proposes that a neural mechanism in the dorsal horns of the spinal cord acts like a gate which can increase or decrease the flow of nerve impulses from peripheral fibers to the central nervous system. Somatic input is therefore subjected to the modulating influence of the gate before it evokes pain perception and response. The degree to which the gate increases or decreases sensory transmission is determined by the relative activity in large-diameter (A-beta) and small diameter (A-delta and C) fibers and by descending influences from the brain. When the amount of information that passes through the gate exceeds a critical level, it activates the neural areas responsible for pain experience and response.”163, p. 222
Techniques to close the gate (inhibit) include stimulation of large nerve fibers to block impulses from the smaller pain fibers. This provides a basis for use of massage and effleurage during labor. With continued use of these techniques, the large nerve fibers become habituated and stimuli from smaller fibers are no longer blocked. Thus, as labor progresses, the woman needs to stimulate other fibers (using techniques such as heat, pressure with change of position, and massage of other areas). Because descending fibers may also inhibit transmission to the brain, concentration techniques may also be useful.41,119,157
The neuromatrix theory of pain expands on the gate control theory and emphasizes that pain is a multi-dimensional, whole body-mind-spirit experience that may underlie the basis for the efficacy of nonpharmacologic pain interventions during labor.114,162,243
“The neuromatrix theory incorporates many of the same elements of the gate control theory. Both theories view the brain as the ultimate arbitrator of the multiple dimensions of pain. However, the gate control theory emphasizes the opening or closing of a gate at the level of the spinal cord as the preeminent mechanism controlling the ultimate perception of pain. The neuromatrix theory of pain recognizes the simultaneous convergence of a panoply of influences, including one’s past experiences, cultural factors, emotional state, cognitive input, stress regulation, and immune systems, as well as immediate sensory input . . . The multiple influences that create pain perception are generated from 3 parallel processing networks: sensory-discriminative (somatosensory components), affective-motivational (limbic system components), and evaluative-cognitive (thalamocortical components). Additional contributions of the autonomic nervous system, the stress response system, and immune system modulation are also recognized in this model.”243,p.483
β-endorphins
Pain during the intrapartum period may be modulated by endogenous opiate peptides such as β-endorphins (B-EPs) and enkephalins. These substances are prohormones derived from precursor proteins such as prepro-opiomelanocortin (POMC) for B-EP and preproenkephalin for enkephalin.78 POMC, found in the anterior pituitary gland, also contains the sequence for ACTH (see Chapter 19) and melanocyte-stimulating hormone. B-EP is also produced by the placenta. Endogenous opioids alter the release of neurotransmitters from afferent nerves and interfere with efferent pathways from the spinal cord to the brain. Thus, within the spinal cord, pain signals may be blocked at the level of the dorsal horns and never be transmitted to the brain. B-EP and other endogenous opioids, in addition to their analgesic role, may also alter mood during pregnancy and have a role in regulation of secretion of pituitary hormones.78 For example, B-EP appears to modulate release of luteinizing hormone, prolactin, growth hormone, and follicle-stimulating hormone by the anterior pituitary and arginine vasopressin from the posterior pituitary gland.78
Maternal plasma B-EP levels increase during pregnancy, especially from 28 weeks on, and are significantly elevated during late pregnancy and labor.46,78 Increased levels may be related to the increase in corticotropin-releasing hormone near term (see Chapters 4 and 19).46 Maximum levels of B-EP are found during parturition and decrease postpartum.260 Levels correlate with uterine muscle contraction and cervical effacement. B-EP release may be stimulated by stress as an adaptive response. Endorphins may increase the pain threshold, are associated with feelings of euphoria and analgesia, and may enable the woman to tolerate the pain of labor and delivery.
The increased levels of B-EP during labor may contribute to the decreased doses of anesthetic drugs generally required in pregnant women compared with nonpregnant women.46,79 Women with low B-EP levels at term may have a greater need for pain medication during labor.46,79 The variable decrease in doses of local anesthetics for epidural and spinal blocks is also caused by vascular congestion within the spinal canal and progesterone as well as the altered neuronal sensitivity. Increased progesterone levels also contribute to the decreased doses of inhalation anesthetics needed for pregnant women through their effect on the respiratory system.
Postpartum period
B-EP levels decrease by 24 hours after birth. Levels are higher after vaginal versus cesarean birth.260 Levels of B-EP are twice as high in colostrum as in maternal plasma and may assist the newborn in the transition to extrauterine life and mediate the stressful events of labor and delivery.260
During the postpartum period the ocular and otolaryngeal changes resolve as the physiologic and hormonal adaptations of pregnancy are reversed. Sensitivity of the cornea returns to the usual parameters by 6 to 8 weeks postpartum.172 Intraocular pressure returns to prepregnancy levels by 2 to 3 months postpartum.224 Both ptosis and subconjunctival hemorrhages disappear spontaneously.172 Nasal congestion, ear stuffiness, tinnitus, and laryngeal changes and related discomforts usually disappear within a few days after delivery.65,94,129,215,216 Headaches, generally bilateral and frontal, are a common discomfort in the first week after delivery. Postpartum headaches tend to begin around the time of postpartum weight loss and have been attributed to alterations in fluid and electrolyte balance.
Sleep during the postpartum period
Sleep is altered during the immediate postpartum period. Most of these changes normalize after the first weeks postpartum, but primiparas may experience altered sleep for up to 3 months.61,137 Stage 1 NREM sleep is longer immediately after birth than before birth. Stage 4 NREM sleep is also longer immediately after birth than before birth, with a gradual change to prepregnancy levels by about 2 weeks. REM sleep is decreased and awake time increased on the first postpartum night, with a reversal of these findings by 3 days.112,122 These changes are probably related to the initial euphoria and discomfort after childbirth, followed by fatigue and restoration. Postpartum women have less overall sleep time and more night awakenings than nonpregnant women do. These night awakenings are often associated with urination, discomfort, activity by roommates or nursing staff, and infant feeding. Opportunities for restorative sleep after delivery and during the first postpartum night are often impeded by the environment and interruptions for nursing care activities.112,140 Disturbances in maternal sleep lead to fatigue and a perceived lack of sleep effectivensss.207
The main reasons for night wakening postpartum are newborn sleep and feeding patterns.112 Maternal night wakefulness decreases significantly from weeks 3 to 12.178 This decrease is related to development of the infant’s sleep-wake rhythm. Women who breastfeed have more deep sleep and overall sleep, possibly related to prolactin secretion, less arousal due to closer infant proximity, and infant sleep-wake patterns.24,139,247
Sleep medications alter sleep physiology and suppress REM sleep and NREM stages 3 and 4 and thus should be avoided. OSA and sleep disorder breathing generally resolve postpartum as the changes in the airway and larynx resolve.29,209
Postpartum discomfort
During the first few days to weeks of the postpartum period, the woman may experience considerable discomfort. The discomfort and pain can arise from a variety of sources, including an episiotomy, lacerations, perineal trauma, incisions, uterine contractions after delivery (afterpains), hemorrhoids, breast engorgement, and nipple tenderness. Breast engorgement initially occurs in both the lactating and nonlactating woman because of stasis and distention of the vascular and lymphatic circulations. In the breastfeeding woman, secondary engorgement occurs because of distention of the breast with milk as lactation is established. Alterations in comfort not only cause physical and emotional stress but can also interfere with the ability of the woman to interact with and care for her infant. Back pain generally disappears within a few weeks or months after delivery, although 8% to 10% of women continue to report pain 1 to 2 years later.90,247
Clinical implications for the pregnant woman and her fetus
Ocular adaptations
Although ocular alterations during pregnancy generally do not have major clinical significance, they can result in minor discomforts, particularly for women who wear contact lenses. Some women may be unable to tolerate their lenses and may occasionally develop corneal edema. The basis for this intolerance is believed to be retention of water by the cornea, changes in the composition of tears, and alterations in corneal topography.182,224 Changes in tear composition can make the contact lenses feel greasy shortly after insertion and cause blurring of vision.172,182 Pregnancy may also alter healing following photorefractive keratectomy. Myopic regression and corneal haziness are reported in about 10% of postpartum women.222 Alterations in refractory power along with occasional transient insufficiency of accommodation can cause difficulty in reading and in near vision or blurred vision in someone who is farsighted.172,217,238 These alterations resolve in the postpartum period. New prescriptions for glasses or contact lenses should be delayed until several weeks after delivery.
Women with specific disorders such as preeclampsia and diabetes mellitus may have ocular complications associated with their disease process. Preeclampsia is associated with vasospasm of the conjunctival vessels and narrowing of the retinal arterioles. The latter may progress to severe arteriolar spasm or multiple retinal hemorrhages. Most of these changes reverse within 3 weeks postpartum.224,238 In severe disease retinal detachment may occur.238 Mild to moderate visual disturbances are most common; severe or complete loss of vision is rare.238 However, up to 15% of women with severe preeclampsia develop cortical blindness that is almost always transient, lasting 4 hours to 8 days.224 The changes that occur with preeclampsia may be related to vascular changes, endothelial damage, coexisting systemic vascular disease, altered cerebral autoregulation, and hypoperfusion with ischemia or hyperperfusion with edema.224
Development and progression of diabetic retinopathy in pregnant women are more closely correlated with the duration of the disorder, severity of the diabetes, coexisting hypertension or preeclampsia, and degree of glycemic control.182,217,224 Pregnant diabetic women (particularly those with established proliferative retinopathy) often demonstrate deterioration related to the metabolic and hormonal changes of pregnancy. Some women without diabetic retinopathy at the onset of pregnancy may develop mild nonproliferative diabetic retinopathy (NPDR) during pregnancy and those with NPDR may have progression.217,224 Although reversal of these pregnancy-induced changes usually occurs over the first 6 to 12 months postpartum, some women, especially those with preexisting retinopathy, do not experience complete return to their prepregnant status.54,217,224,238 Careful baseline assessment and surveillance during pregnancy are essential. Women with gestational diabetes are not at increased risk for retinopathy during pregnancy.54,217
Because intraocular pressure falls during pregnancy, the pregnant woman with glaucoma may experience an improvement with a decreased need for medications.54,182,238 This has an additional advantage of reducing fetal exposure to these agents, which should be used with caution and carefully monitored.217 Many ocular inflammatory disorders improve during pregnancy, possibly because of increased cortisol and other glucocorticosteroids, with exacerbation postpartum.
Any symptoms of eye infections in the pregnant woman must be assessed, because some sexually transmitted organisms such as herpes simplex virus and chlamydia may cause concurrent ocular infections. The presence of these organisms can have adverse consequences for the neonate if the mother also has a genital infection. For example, genital chlamydia can cause conjunctivitis and pneumonia in the newborn, and genital herpes simplex virus can cause disseminated or localized neonatal infection. Treatment of maternal ocular disorders during pregnancy should be done cautiously and with attention paid to the possible effects of the medication on the fetus. Even topical eye ointments must be used with caution because they can be absorbed systemically and cross the placenta to the fetus.238 If these agents must be used, nasolacrimal occlusion after instillation may reduce systemic absorption.224
Musculoskeletal discomforts
The pregnant woman may experience discomfort or pain associated with breast changes or stretching of the round ligament with growth of the uterus, pressure of uterine nerve roots, or pressure of the presenting part on the perineum. The latter type of pain is most prominent close to the onset of labor and is aggravated by vascular engorgement of these tissues. Increased joint mobility can result in muscle and ligament strain and discomfort. Pregnant women also have an increased risk of falls.103,159,193 Falls are reported in 27% of pregnant women and are the leading cause of emergency department visits during pregnancy.158,159 The increased risk of falls is related to weight gain, shift in the center of gravity, edema, deceased abdominal muscle strength, changes in mechanical load, ligament laxity, and changes in joint kinetics.158,159 Regular exercise may be useful in reducing the risk of falls.159
Hip and knee joint pain may occur due to ligamentous laxity, joint strain, and weight gain.26,203 A 20% weight gain increases the force on weight-bearing joints by 50% to 100% during pregnancy.203 Knee pain is due to patellofemoral dysfunction from ligamentous laxity, increased femoral torsion, and increased pelvic width.203 Preexisting knee pain may increase or pain may occur for the first time. These changes are usually benign and reverse postpartum.203 Pubic pain results from inflammation of the symphysis pubis and usually responds to rest and ice.203
Many pregnant women experience low back pain (including lumbar pain and pelvic girdle pain) during pregnancy due to a combination of hormonal and mechanical factors including exaggeration of the lumbar lordotic curve due to shifting of the center of gravity, weight gain, and relaxation of ligaments, or from muscle spasm due to pressure on nerve roots.26,95,247 The sacroiliac ligaments, which usually resist femoral rotation, are less likely to do so during pregnancy. This along with the lumbar lordosis “shifts the center of gravity anteriorly, causing a strain on the lower back and sacroiliac joint. As the strong sacroiliac ligaments become increasingly lax during pregnancy, this allows an increase in the forward shift of the uterus, thereby placing more strain on the pelvic floor and back.”203
Backache occurs in about 45% to 50% of women, most commonly after 18 weeks of pregnancy, although high backache earlier may result from breast alterations.57,90,95,103,116,165,247,254 Approximately 10% of these women experience severe back pain, peaking in intensity during the evening and night, and localized to the lower back or sacroiliac region.
An increased frequency of backache is reported in multiparas, in individuals with a history of back pain before pregnancy or with a previous pregnancy, and with increasing maternal age.103,116,203,254 Backache has not been associated with weight gain in pregnancy, height, or infant birth weight.26,103,116,203,247 Women tend to experience less pain if they are physically fit before pregnancy, exercise during pregnancy, and have had education on postural adjustments (e.g., upright posture, tucking the pelvis, rotating shoulders) to reduce back pain.247 Other recommendations include avoiding anything that increases the lordosis (e.g., high-heeled shoes), application of local heat, exercises to increase lower abdominal and back muscle tone beginning early in pregnancy, abdominal pillows, backrubs, use of a firm mattress, bed board, or back support, pelvic tilt exercise, aerobic exercise such as swimming, and conditioning before subsequent pregnancies.57,116,188,247 Acupuncture has also been found to provide relief.131,228 Backache, round ligament pain, or other discomfort should be assessed to distinguish “normal” discomfort from other processes such as preterm labor.
Exacerbation of intervertebral disk disorders is seen during pregnancy and the immediate postpartum period, probably because of postural changes and an increase in mechanical stress.11,26,230 The most frequently affected areas are the fifth lumbar and first sacral nerve roots. Management usually involves bed rest. Spondylolisthesis (slipping of one lumbar vertebra forward onto the vertebra below) especially at L4 to L5 or at L5 to S1 is aggravated.203 True herniation of the disk is rare.95,203,230
During the third trimester, the woman may experience numbness and tingling of the arms, fingers, legs, and toes. Paresthesia of the upper extremities may be caused by marked lordosis along with anterior flexion of the neck and slumping of the shoulders, placing traction on the brachial, ulnar, and median nerves. In the legs and toes, paresthesia may be caused by pressure of the uterus on the blood vessels and nerves supplying the lower extremities. Painful legs are experienced by many pregnant women and arise either from the mechanical effects of the uterus (e.g., the altered gait and posture during pregnancy and compression of the inferior vena cava and iliac veins when supine) or from systemic alterations. Systemic alterations that can lead to painful legs include musculoskeletal changes, reduced serum albumin (decreasing colloid osmotic pressure and increasing dependent edema), changes in coagulation, and alterations in calcium and phosphorus metabolism and diet. These causes must be differentiated from thromboembolic disorders, which are also more prevalent during pregnancy (see Chapter 8). Leg cramps during the last few months of gestation are common. These cramps may be related to alterations in calcium and phosphorus metabolism (see Chapter 17) or to pressure of the enlarged uterus on pelvic blood vessels or nerves supplying the lower extremities.
Restless legs syndrome
Restless legs syndrome is an idiopathic disorder seen in up to 26% of pregnant women (2 to 3 times the risk in the general population) by the third trimester and may interfere with sleep.57,64,105,239 This syndrome is often mistaken for leg cramps, and neuromuscular findings may be similar to those associated with excess caffeine consumption.57 Restless legs syndrome usually occurs 10 to 20 minutes after the woman gets into bed. It is characterized by an urge to move the legs accompanied by unpleasant sensations, a “creeping, wormy, burning ache [that] develops within their legs. The more the urge to allow the legs to fidget is resisted, the greater the urge becomes until it can no longer be withstood.”57 Restless legs syndrome is usually bilateral and symmetric, occasionally involving the arms as well.64 This transient disorder generally appears in the third trimester and disappears shortly after delivery.64,239 The neurologic examination is negative.
The cause of restless legs syndrome is unknown. It may have a genetic basis or be related to the hormonal changes of pregnancy or may be related to alterations in dopamine metabolism.64,139 If the woman is iron deficient, improvement in the symptoms of restless legs syndrome may occur with treatment of the anemia. Women who are supplemented with folic acid have been reported to be less likely to develop this disorder.57,64,96,139 Restless legs syndrome may also be associated with polyneuropathy and vascular insufficiency. Usually no treatment is indicated, other than ensuring adequate iron and folate intake. Walking may relieve some symptoms. If the disorder is severe, pharmacologic therapy may be considered; however, these may have potential risk to the fetus so risk-benefit must be carefully evaluated.57,105,239
Chorea gravidarum
Chorea gravidarum is a disorder involving rapid, brief, nonrhythmic, involuntary, jerky movements of the limbs, nonpatterned facial grimacing, and slurred speech.57 Mild cases may involve only persistent restlessness and clumsiness.11 This disorder is seen both in pregnancy (incidence of 1 in 139,000) and in women on oral contraceptives.11,57 Symptoms usually begin in the first trimester and may persist to the postpartum period.57 Approximately 30% of pregnant women with this disorder become asymptomatic by the third trimester; the remainder become asymptomatic shortly after delivery.57 A recurrence rate of 20% has been reported with subsequent pregnancies.11
The cause of chorea gravidarum is unknown, although it is related to streptococcal infection and is most common in women with a history of rheumatic fever or heart disease.11 This disorder has also been related to hormonal changes during pregnancy or contraceptive use, in that estrogen can stimulate postsynaptic dopamine receptors (inhibitory transmitters with effects in the motor cortex and basal ganglia).57 Chorea gravidarum may also have a hematologic or immunologic basis. Changes in these systems during pregnancy may exacerbate a preexisting basal ganglia disturbance.11 There are no fetal effects reported.
Headache
Heacaches are common complaints during pregnancy and the postpartum period.126 The most common forms of headaches in pregnant and breastfeeding women are those caused by muscular contraction/tension or migraines without aura.57,155 Tension headaches are characterized by a persistent bandlike or viselike pain extending from the base of the neck to the forehead. The woman often notices the headache on awakening, with worsening of symptoms during the evening.11,57 The discomfort may be aggravated by postural changes or stress. Tension headaches during pregnancy may also be due to hormonal influences, eye strain secondary to ocular changes, nasal congestion, emotional tension, muscle spasm, fatigue, altered cerebral fluid dynamics, or the mild respiratory alkalosis characteristic of pregnancy (see Chapter 10). Reproductive hormones, especially estrogens, interact with the trigeminovascular system to alter cerebral blood flow and neurochemical release.
Because headaches may also be a symptom of disorders such as preeclampsia, however, any pregnant woman complaining of headaches must be carefully evaluated. Management includes massaging neck and shoulder muscles, application of heat or ice to the neck, rest, warm baths, and minimal use of simple analgesics (acetaminophen).57 Sedatives and hypnotics are not recommended for routine use in pregnant or lactating women because they are ineffective and metabolites cross the placenta and the blood-milk barrier and are excreted more slowly by the fetus and infant.11
Migraine headaches
Migraine headaches occur in 3% to 5% of the general population. Migraines are more common in women and often occur with menses, with the initial development of migraine headaches associated with onset of menses. Migraines may also occur for the first time during the first 2 to 3 months postpartum. Although the frequency of headaches decreases with age, migraines may flare up or start with menopause.57 Headaches are also more frequent and severe in women on oral contraceptives, especially pills with higher estrogen content.11,57
Both migraines with and without aura are vascular headaches caused by cerebral vasodilation and cranial artery dilation. The severe throbbing during the initial phases is attributed to intense cerebral vasoconstriction with subsequent vasodilation.57 Migraines with and without aura may be different clinical entities.175,241 Migraines without aura (MO) are seen most frequently; migraine with aura (previously called classic migraine) are less common.155 Migraines with aura (MA) are characterized by 20 to 30 minutes of sensorimotor prodromal symptoms followed by a severe unilateral headache accompanied by nausea. The prodromal symptoms usually involve visual phenomena but may also include aphasia, hemiplegia, and paresthesia. MO is unilateral less often and not associated with prodromal symptoms.
MO generally improve or disappear during pregnancy.175,241 During pregnancy, approximately 48% to 80% of women with a history of MO experience complete remission or decreased frequency, particularly during the second and third trimesters as estrogen and progesterone levels increase.107,155,241 This change is particularly notable in women with a history of menstrual migraine (i.e., migraine associated with estrogen withdrawal). Migraines usually return within a few hours or days after delivery or with the first postpregnancy menses.155 Return of headaches is delayed in women who breastfeed, probably due to the altered hormonal milieu.175,241
MA may occur for the first time during pregnancy, usually during the first trimester, although they can occur in any trimester, and some women may experience a worsening of their migraines.57,107,241 Over 20% of women with MA have their first headache during pregnancy and about 50% of women with MA continue to have attacks during pregnancy.241
The basis for remission of migraine headaches during pregnancy is unclear. Although the cause of migraines is unknown, migraines have been attributed to estrogen deficiency, excessive gonadotropins, fluid and salt retention, and increased red blood cell (RBC) mass. Migraines are associated with periods of estrogen withdrawal such as menses or menopause, which involve changes in estrogen and progesterone levels and patterns of circulating estrogens.11 Thus migraine remission during pregnancy may be related to changes in estrogen (particularly the sustained estrogen levels), progesterone, and aldosterone, or to hematologic and cardiovascular alterations.175,241 The progressive increase in B-EP during pregnancy may also contribute to remissions.241 Migraines have been linked to an increased risk of developing gestational hypertension, preeclampsia and vascular complications.4,175
Dietary and lifestyle interventions for migraines include a balanced diet, avoidance of dietary triggers, alcohol and smoking, exercise, and relaxation. The efficacy of non-pharmacological interventions such as relaxation techniques, including biofeedback, and acupuncture for migraine prophylaxis has also been documented.3
MA headaches are often treated with ergot alkaloids. Because these compounds have oxytocic properties and are Food and Drug Administration pregnancy category X, they should not be used during pregnancy because of the risk of miscarriage, fetal distress, preterm labor, and possibly birth defects (especially intestinal atresia and Moebius syndrome).57,72,155,260 Analgesics (acetaminophen) are used for symptomatic relief. As noted above, other interventions include avoidance of triggers, biofeedback, analgesics, sedatives, and β-blockers. Propranolol has been used as a prophylactic treatment for frequent MA but its use during pregnancy is controversial because this drug has been associated with altered fetal growth as well as fetal and neonatal β-adrenergic blockade with subsequent decreased responsiveness to stress during asphyxia.11,57,155 Sumatriptan (serotonin agonist) has been used with great caution due to concerns about effects on the fetus, although specific adverse effects have generally not been reported to date in most studies.11,72,107,150,232
The pregnant woman with a chronic neurologic disorder
The physiologic and hormonal changes of pregnancy can influence the course of chronic neurologic and neuromuscular disorders such as epilepsy, myasthenia gravis, and multiple sclerosis. These disorders also have ramifications for the health and well-being of the mother and her infant. The implications of epilepsy and selected other neurologic and neuromuscular disorders during pregnancy are summarized in Table 15-2.
Table 15-2
DISORDER AND BASIS | IMPLICATIONS FOR PREGNANT WOMAN | IMPLICATIONS FOR FETUS/NEONATE |
EPILEPSY | ||
Effect of pregnancy on the course of epilepsy is variable and unpredictable.
Seizure frequency may increase, decrease, or stay the same. Seizures may appear for the first time during pregnancy or reappear after many seizure-free years. Most women return to their prepregnant pattern after delivery.
Usual physiologic changes of pregnancy alter the metabolism of anticonvulsant drugs with a risk of subtherapeutic plasma concentrations and increased risk of seizures.
May need increase in AED dosages (see Chapter 7), especially during the second half of pregnancy (reversed by 6 weeks postpartum).
Higher incidence of stillbirth and possibly preterm labor, but risks of other complications are not necessarily increased.
Maternal bleeding may occur secondary to deficiency of vitamin K–dependent clotting factors, which may be associated with phenytoin or phenobarbital use.
Major risks are from pathophysiologic consequences of maternal seizures and the use of antiepileptic drugs (AEDs).
Each AED has potential fetal and neonatal risks (see Chapter 7), such as fetal anticonvulsant syndrome, congenital anomalies, hemorrhagic disease of the newborn, folic acid deficiency, and neonatal addiction and withdrawal.
Increased frequency of congenital anomalies in both epileptic women who are on AEDs and those who are not. The risk of anomalies is also increased if the father has epilepsy but the mother does not.
Teratogenicity related to specific AEDs is described in Chapter 7.
Risk of hemorrhagic disease of the newborn may be increased due to a deficiency of vitamin K–dependent clotting factors secondary to competitive inhibition of the formation of precursor molecules by AEDs (see Chapter 8).
May improve, worsen, or stay the same (effects are variable with each pregnancy).
Most often exacerbates in first trimester and improves in second and third trimesters, possibly due to effects of blocking of IgG antibodies by immunologic changes of pregnancy (see Chapter 13) or α-fetoprotein.
Increased frequency of exacerbation in first few months postpartum.
No effect on smooth muscle and thus myometrium, so labor is not prolonged, although the woman may have difficulty with expulsive efforts.
Long-term maternal outcome is not altered.
Avoid use of muscle relaxants; magnesium sulfate for preeclampsia (can lead to apnea because hypermagnesemia inhibits release of acetylcholine); inhalation anesthetics and narcotics (because these pregnant women tend to hypoventilate if they have a bulbar muscle weakness); procaine if on pyridostigmine or neostigmine, which inhibit hydrolysis of procaine and can result in seizures (can use lidocaine); extensive regional blocks (may compromise respiration); and azathioprine (potential teratogenic effects).
Fetal myasthenia gravis does not occur, possibly due to blocking of the IgG antibody by α-fetoprotein.
About 10% to 20% of neonates develop transient myasthenia gravis due to passage of maternal IgG antibody against the acetylcholine receptors of striated muscle (see Chapter 13).
Symptoms appear within 12 to 72 hours of birth and resolve spontaneously by 3 months (usually in 1 to 3 weeks).
Infants with transient myasthenia gravis often feed poorly, have a weak cry, are hypotonic, and are at risk for respiratory difficulty and aspiration. Many of these infants require anticholinesterase therapy and some will need assisted ventilation.
Neonatal effects do not correlate with maternal disease severity or antibody titer.
Muscular weakness and myotonia may worsen, often in the second half of pregnancy (possibly due to effects of progesterone on cell membranes).
Delayed gut motility with increased constipation in pregnancy.
Increased risk of spontaneous and habitual abortion, and preterm labor.
Abnormal uterine contractions, prolonged first stage, poor voluntary expulsive efforts in second stage, poor involution with risk of postpartum hemorrhage.
Avoid the use of inhalation anesthetics (often hypoventilate with chronic respiratory acidosis) and depolarizing muscle relaxants (succinylcholine) that can cause myotonic spasms and hyperthermia.
Presence of maternal disorder does not affect infant per se.
If fetus has inherited myotonic muscular dystrophy, severe fetal and neonatal myotonia may occur. Fetal effects may include poor swallowing with hydramnios and arthrogryposis from inactivity; neonates may have respiratory distress and feeding problems.
Tendency for remission during pregnancy; rate of relapses decreases with each trimester, possibly owing to immunosuppressant effects of α-fetoprotein and estrogens with decreased proinflammatory cytokines.
Twofold to threefold increase in relapses in first 3 to 6 months postpartum, possibly due to decreased estrogens and reversal of immune changes of pregnancy.
Usually minimal effect on course of pregnancy or incidence of complications.
May experience worsening of bowel and bladder problems and urinary tract infection; for paraplegic or quadriplegic care and risks similar to those for a woman with a spinal cord injury.
Medications need to be reviewed to evaluate if changes are needed.
Compiled from references 11, 19, 27, 57, 97, 98, 99, 149, 223, 233.
The pregnant woman with epilepsy
Epilepsy is one of the most common neurologic disorders seen in pregnant women. The course of epilepsy is affected by the hormonal and physiologic changes of pregnancy, as well as by psychologic stress. Estrogens and progesterone alter seizure thresholds—estrogens by activating seizure foci, inhibiting γ-amino butyric acid (an inhibitory neurochemical), and potentiating glutamate (an excitatory neurotransmitter); and progesterone by potentiating barbiturate-like ligands at the γ-amino butyric acid channel and thus dampening activity.11,149 These responses may account for the exacerbation of seizure activity with menses in many women with severe epilepsy.57 Seizure activity or susceptibility to seizures may also be affected by water and sodium retention, sleep alterations, and the mild respiratory alkalosis of pregnancy.187 The decreased seizure activity seen in some women may also be due to improved compliance in taking their medication.11,97 The frequency of seizures before pregnancy tends to be predictive of seizure activity during pregnancy.57,97,223
An important factor affecting the pregnant epileptic is the effect of the usual physiologic changes of pregnancy on the metabolism of antiepileptic drugs (AEDs). The goal in managing the pregnant epileptic is to keep the mother free of seizures and to minimize the effects of epilepsy on both the pregnant woman and fetus, including fetal teratogenic effects.57,98,187 Fetal anomalies and an increased risk of developmental delay syndrome has been described for AEDs. Congenital anomalies seen with increased frequency in infants of women with epilepsy include neural tube defects (especially with valproic acid), cleft lip and palate, congenital heart defects, and urogenital defects.98,187 Some AEDs cause a mild folate deficiency so folate supplementation is especially important for the woman with epilepsy.11,99,149 However, excess folate should be avoided because this may alter hepatic microsomal enzyme activity and decrease AED levels.149 Use and risks of AEDs are discussed further in Chapter 7.
Peripheral neuropathies
Pregnancy, labor and delivery, and lactation are associated with an increased incidence of a variety of peripheral neuropathies. Most of these disorders, although uncomfortable, do not alter the course of pregnancy, nor are they associated with maternal or fetal and neonatal complications. Other peripheral neuropathies develop secondary to trauma or pressure injury during the intrapartum or postpartum periods. Table 15-3 summarizes these disorders and their implications.
Table 15-3
Peripheral Neuropathies during Pregnancy and Lactation
DISORDER | DESCRIPTION | IMPLICATION |
NEUROPATHIES ASSOCIATED WITH PREGNANCY | ||
Bell’s palsy (idiopathic facial paralysis) | Acute unilateral neuropathy of the seventh cranial nerve leading to facial paralysis with weakness of the forehead and lower face |
Three times more frequent during pregnancy, possibly because of an inflammatory reaction with entrapment of the medial and occasionally ulnar nerve with soft tissue edema Most often occurs in the third trimester or first 2 weeks postpartum; associated with preeclampsia Onset late in pregnancy usually associated with full recovery (but may take months) and generally requires no treatment if partial or mild |
May develop in pregnancy because of excessive fluid retention
Onset usually in second or third trimester
Nocturnal hand pain reported by 20% to 40% of pregnant women, with electromyographic (EMG) evidence of this syndrome in about 5%
Supportive treatment (splinting of wrist at night); a few may require surgery
Most cases resolve by 3 months postpartum; may recur with later pregnancies
Compiled from references 11, 26, 27, 57, 79, 101, 103, 134, 153, 203, 223.
The woman with a spinal cord injury
Successful pregnancies are not uncommon for women who are paraplegic or quadriplegic. The usual physiologic changes of pregnancy place the woman with a spinal cord transection at increased risk for certain problems, however, including increased urinary incontinence, urinary tract and other infections, constipation, and pressure ulcers.11 Most of these problems can be prevented or minimized with good care. Management of the paralyzed pregnant woman usually includes careful attention to bladder and skin care, high-bulk diet, adequate fluid intake, prevention of anemia, stool softeners, and acidification of the urine with vitamin C supplements.11,57
Labor and delivery present unique challenges and risks. Since contraction of the myometrium is relatively independent of neuronal influence (see Chapter 4), uterine contractions are usually normal. However, the level of the lesion influences the woman’s perception of contractions. Sacral anesthesia is present in all of these women. Women with cauda equina lesions have relaxed perineal muscles. Women with lesions below T10 to T11—the level at which the uterine sensory nerves enter the spinal cord—experience labor pain.57 Women with lesions at T10 and T11 may perceive contractions as abdominal discomfort or muscle spasm.
If the lesion is above T9, labor will be painless, the onset of contractions will not be felt, and delivery may be precipitous.116 These women need careful monitoring of the cervix and contractions from about 24 weeks’ gestation on because preterm labor is common.11 If the woman also has spasticity, local somatic reflex arcs may be activated by labor contractions. This may result in painful extensor and flexor muscle spasms and sustained ankle clonus.11,57
Autonomic hyperreflexia or dysreflexia (also called autonomic stress syndrome) may occur during labor in women with complete cord lesions above T5 to T6 (above the outflow of the splanchnic autonomic nerves). This syndrome is characterized by hyperstimulation of the autonomic system with brief periods of severe hypertension, throbbing headaches, reflex bradycardia, sweating, nasal congestion, cutaneous vasodilation with flushed skin, and piloerection above the level of the lesion.11,57,116,189 Symptoms generally occur with uterine contractions and are most prominent immediately before delivery. Symptoms may be mistaken for preeclampsia.11,57 Autonomic hyperreflexia occurs because of the sudden release of large amounts of catecholamines during uterine contractions. This syndrome can result in life-threatening complications including intracranial hemorrhage and cardiac arrhythmias. Treatment includes regional anesthesia, antihypertensive agents, and perhaps use of α1-adrenergic blockers or peripheral vasodilators.57,116,189
Forceps may be required during delivery if the muscles used for expulsion during the second stage are paralyzed or if severe hyperreflexia occurs.57 During the postpartum period, care involves prevention of complications of the elimination and integumentary systems. Poor wound healing is a concern and may be aggravated by anemia. Paraplegic and quadriplegic women have let-down reflexes and can successfully breastfeed.
The woman with a brain or spinal cord tumor
Brain tumors, especially meningiomas, may enlarge during pregnancy because of stimulation of tumor estrogen receptors that stimulate growth of neoplastic cells, increased vascularity and blood volume, and increased extracellular volume.177 As a result of these changes, many tumors become symptomatic during the second half of pregnancy. Tumors may temporarily regress postpartum. Brain tumors account for approximately 10% of all maternal deaths.57
Spinal cord tumors tend to exacerbate with pregnancy and menstruation. The most common spinal cord lesions in pregnant women are arteriovenous malformations (AVMs) and angiomas. An AVM is characterized by rapid shunting of blood between an artery and vein without any intervening capillaries, thus depriving adjacent areas of oxygen and nutrients. Exacerbations during pregnancy may be related to (1) mechanical pressure of the gravid uterus on the vena cava, causing partial outflow obstruction with shifting of blood flow to vertebral and epidural veins and engorgement of the venous side of the malformation; (2) increased vascularity and blood volume; or (3) dilation of the shunt by estrogens.57
Cerebral vascular disorders
The physiologic and hormonal changes of pregnancy also increase the risk of certain cerebral vascular disorders with life-threatening consequences. Although most individual disorders are relatively rare, cerebral vascular disorders as a group are not uncommon during pregnancy.11 The basis for and risks of subarachnoid hemorrhage, cerebral ischemia, and cerebral venous thrombosis during pregnancy are summarized in Table 15-4.
Table 15-4
Cerebrovascular Disorders: Possible Basis for Increased Risk during Pregnancy
Risk increased due to hormonal effects on arterial and venous intima and media organization, increased plasma volume.
May be related to alterations of arterial and venous intima by hormonal changes during pregnancy.
Bleeding during pregnancy may be associated with enlargement or increased shunting during pregnancy related to altered hemodynamics, increased cerebral blood flow (CBF), changes in coagulation, or hormonal influences altering integrity of blood vessels.
Bleeding during labor in women with these anomalies may be initiated by rapid pressure and flow changes associated with Valsalva maneuver.
Hormonal changes, anemia, and altered blood coagulation during pregnancy may be factors predisposing to emboli formation.
Often occurs secondary to other disorders such as mitral valve prolapse, hypertension, subacute bacterial endocarditis, hypotension, or sickle cell anemia, or with use of anticoagulants.
Preeclampsia and eclampsia
CNS changes occur in both preeclamptic and eclamptic women. The more severe changes markedly increase the risk of fetal and maternal mortality. CNS manifestations of preeclampsia and eclampsia may include cerebral irritability (e.g., hyperreflexia, headache, clonus, altered consciousness), visual disturbances, cerebral edema, cerebral hemorrhage, and convulsions (with eclampsia). These events are the result of arteriolar vasospasm and vasoconstriction, fluid shifts from the vascular to intravascular space, and possibly a failure of cerebral autoregulation with localized capillary rupture.57 Most changes are reversible, but some women with eclampsia may have permanent changes, although few long-term studies have been done.244
Eclampsia is the development of seizures in a pregnant woman with signs and symptoms of preeclampsia. Convulsions may be focal, multifocal, or generalized. Potential etiologic factors for seizures and coma in these women include cerebral vasospasm, hemorrhage, ischemia, edema, and hypertensive or metabolic encephalopathy. Donaldson and Duffy suggest that the cerebral manifestations of eclampsia are primarily due to severe vasoconstriction in association with failure of cerebral autoregulation to limit perfusion and pressure within the thin-walled cerebral capillaries.57 This leads to a pressure-induced rupture of thin-walled capillaries with vasogenic edema and hemorrhage. “In physiologic terms the upper limit of the autoregulation of cerebral perfusion of blood pressure, which is proportional to mean arterial blood pressure [and thus not standard diastolic or systolic values], has been exceeded. Cerebral eclampsia is hypertensive encephalopathy in previously normotensive women.”57 Magnesium sulfate, which may selectively increase cerebral blood flow and oxygen consumption, has been found to be more effective than AEDs in preventing recurrent seizures in these women.180 Hypertensive disorders in pregnancy are discussed in Chapter 9.
Summary
The neurologic system and mediation of discomfort and pain are often of major concern for women during the intrapartum period. This system is probably the one about which least is known regarding specific effects of pregnancy. The pregnant woman experiences alterations in her neuromuscular and sensory systems related to the hormonal and physiologic adaptations of gestation. These changes may result in common experiences and discomforts of pregnancy—including backaches and headaches, ocular and voice changes, nasal stuffiness, epistaxis, sleep disturbances, postpartum blues, and peripheral neuropathies—and alter the course of neurologic disorders. Recommendations for clinical practice related to the neuromuscular and sensory systems during pregnancy are summarized in Table 15-5.
Table 15-5
Recognize the usual ocular, otolaryngeal, and neuromuscular changes during pregnancy (pp. 509-512).
Provide anticipatory teaching regarding usual ocular, otolaryngeal, and neuromuscular changes during pregnancy (pp. 509-512).
Counsel women regarding intervention strategies for common problems related to ocular changes (pp. 509-510, 516).
Counsel women, when possible, to delay getting new prescriptions for glasses or contact lenses until several weeks postpartum (p. 516).
Counsel women to avoid cycloplegic or mydriatic agents and topical ophthalmic ointments during pregnancy and, if necessary, to use nasolacrimal occlusion after use (p. 516).
Counsel women regarding intervention strategies for common problems related to otolaryngeal changes (p. 510).
Counsel women regarding intervention strategies for common problems related to musculoskeletal changes (pp. 510-511, 516-517).
Assess posture and lifting techniques used by pregnant women (pp. 510-511, 516-517).
Teach pregnant women relaxation techniques and exercises to reduce muscle strain and tension (pp. 516-517).
Recognize and assess for factors associated with painful legs during pregnancy (pp. 517-518).
Assess sleep patterns of pregnant and postpartum women and implement interventions to enhance rest and sleep (pp. 511-512, 515).
Avoid use of sleep medications during pregnancy (p. 515).
Assess women complaining of backache, headache, round ligament pain, or other discomfort for signs of other disorders (pp. 516-519).
Understand the basis for maternal feelings of pain and discomfort during labor and delivery and implement appropriate interventions (pp. 512-515, Figure 15-3, and Table 15-1).
Counsel women during the postpartum period regarding alterations in comfort and implement appropriate interventions (p. 515).
Assess and counsel women with diabetes mellitus and preeclampsia regarding potential ocular complications (p. 516).
Assess eye infections in pregnant women and evaluate for pathogenesis by organisms implicated in sexually transmitted disorders (p. 516).
Counsel women with migraine headaches regarding effects of pregnancy on their disorder and remission postpartum (pp. 518-519).
Know and counsel women regarding the side effects of drugs used to treat specific neurologic disorders and potential toxicity to the woman and fetus (pp. 518-519 and Chapter 7).
Counsel women with chronic neurologic disorders regarding the impact of their disorder on pregnancy and of pregnancy on the disorder (pp. 519, 521, 523 and Table 15-2).
Know the effects of physiologic alterations during pregnancy on metabolism of anticonvulsants (p. 519, Table 15-2, and Chapter 7).
Monitor epileptic women during pregnancy and postpartum for levels of and responses to anticonvulsants (p. 519, Table 15-2, and Chapter 7).
Evaluate the infant of an epileptic woman for congenital anomalies, folate deficiency, drug withdrawal, bleeding, and feeding behaviors (p. 519 and Table 15-2).
Ensure that vitamin K is administered to newborns of epileptic women who are on anticonvulsants (p. 519 and Chapter 8).
Recognize signs and symptoms of peripheral neuropathies and implement appropriate preventive and intervention strategies (p. 519 and Table 15-3).
Evaluate the woman with a spinal cord injury for bowel, bladder, and integumentary complications and implement appropriate interventions (pp. 519, 521).
Monitor the woman with a spinal cord lesion for initiation of contractions, progression of labor, and autonomic hyperreflexia (pp. 519, 521).
Recognize the basis for and risks of cerebral vascular disorders during pregnancy (p. 523 and Table 15-4).
Recognize the cerebral manifestations of preeclampsia and eclampsia and implement appropriate interventions (pp. 523-524).
Development of the neurologic, muscular, and sensory systems in the fetus
The neurologic, muscular and sensory systems undergo a series of complex structural and functional changes to reach maturation. The central nervous system (CNS) is one of the earliest systems to begin development and the latest to completely mature. Table 15-6 summarizes CNS development and the timing and origin of specific anomalies. This section examines anatomic and functional development of the fetal nervous, sensory, and motor systems and development of sensory abilities in preterm infants. CNS development occurs via the interaction of multiple genes and signaling molecules, including Wnt, neurotropic proteins such as transforming growth factors (especially sonic hedgehog), fibroblast growth factors, platelet-derived growth factors and other neurotropins.37,194 Other factors involved in CNS development are vitamins (especially vitamin A and folic acid), essential fatty acids, thyroid hormones (see Chapter 19), and steroid hormones.194
Table 15-6
Major Stages of Human Brain Development and Related Disorders
TIME OF OCCURRENCE (WEEKS’ GESTATION) | STAGE OF HUMAN DEVELOPMENT | MAJOR EVENTS | MAJOR ANOMALIES DURING STAGE | |
3 to 4 | Neurulation | Notochord (gives rise to neural plate, neural tube, and neural crest cells) | ||
Neural plate | ||||
Neural tube | Brain differentiation of prosencephalon, mesencephalon, and rhombencephalon at 20 days |
Adapted from Hill, A. & Volpe, J.J. (1989). Fetal neurology. New York: Raven.
Anatomic development of the central nervous system
Embryonic development of the central nervous system
The development of the nervous system begins approximately 18 days after fertilization.173 The process by which the beginnings of the nervous system are laid down is called primary neurulation and includes formation of the neural plate, neural folds, and neural tube (Figure 15-4). This process occurs on the dorsal surface of the embryo and leads to formation of the brain and spinal cord (dorsal induction).50,249
The neural plate develops an invagination in its center, called the neural groove, beginning 22 to 23 days after fertilization. Bulges on both sides of the neural tube groove, called the neural folds, accompany the invagination process. The neural folds continue to enlarge, enveloping the neural groove, and fuse to form the neural tube, an entity separate from the overlying ectodermal layer.173 The neural tube forms the CNS, with the rostral (anterior) portion developing into the brain (Figure 15-5) and the caudal (posterior) portion into the spinal cord. The neocortex forms in the dorsolateral wall of the rostral portion of the neural tube.53 The ventricles and canal of the spinal cord are derived from the lumen of the neural tube.173 Closure of the neural tube begins in the area of the future lower medulla at 22 days and proceeds in cephalad and caudal directions. The rostral opening (neuropore) closes by approximately 24 days; the caudal neuropore (at L1 to L2) closes 2 days later at approximately 26 days at the level of the upper sacrum.249 Failure of these neuropores to close gives rise to neural tube defects (NTDs).
Formation of the caudal portion of the neural tube in the lower sacral and coccygeal areas begins at 28 to 32 days. This process is known as secondary neurulation. Vacuoles develop in the caudal cell mass at the end of the neural tube. These vacuoles coalesce and fuse with the end of the neural tube. This process continues until 7 weeks, followed by a regression of much of the caudal mass that continues until after birth.249
As the neural tube is forming, neuroectodermal cells at the edge of the neural plate near the border with the ectoderm migrate into the neural folds located on both sides of the developing neural groove, forming the neural crest. Neural crest cells later migrate into different areas of the developing embryo to form the craniofacial skeleton, connective tissue, and neurons; peripheral nervous system, including the cranial and spinal nerve sensory ganglia; autonomic nervous system; Schwann cells; pigment cells; the pia and arachnoid layers of the meninges; and the peripheral nerves.50,173,210 Induction of the neural crest cells occurs via signaling molecules from the mesoderm and endoderm, such as transforming growth factors (including bone morphogenetic proteins), fibroblast growth factors, and Wnt.
Ventral induction (prosencephalon development) refers to processes occurring on the ventral portion of the embryo, leading to formation of the face and forebrain, including eye formation, olfactory formation, and forebrain structures. Thus, errors of ventral induction often produce both facial and nervous system anomalies.249 Sonic hedgehog signaling (see Chapter 3) is the prominent molecular pathway in prosencephalon development.249 After closure of the rostral portion of the neural tube, three vesicles form that are the precursors of the brain. These vesicles, the forebrain (prosencephalon), midbrain (mesencephalon), and hindbrain (rhombencephalon), develop in the fourth week. With further differentiation the forebrain becomes the telencephalon and diencephalon, while the hindbrain becomes the metencephalon and myelencephalon, creating five vesicles. Adult derivatives of the vesicles are shown in Figure 15-5.
In the caudal portion of the neural tube, the alar (dorsal) and basal (ventral) plates develop. These structures are the beginning formation of the motor and sensory tracts that develop in the ventral and dorsal areas, respectively, of the cord.173 In conjunction with formation of the spinal cord from the caudal neural tube, cells from the neural crest break into groups along the length of the cord, forming spinal and cranial ganglia and ganglia of the autonomic nervous system. Further differentiation and migration of neural crest cells and their fibers result in formation of the peripheral nerves (somatic and visceral, sensory and motor) and their connections. The primitive brain structures also go through a series of flexures or foldings (Figure 15-6, A).
Common embryonic anomalies of the central nervous system
The most common CNS anomalies arise in the embryonic period during the period of primary neurulation and result from failure of neural tube closure. These anomalies include anencephaly, myelomeningocele, encephalocele, and spina bifida occulta. NTDs are usually accompanied by alterations in vertebral, meningeal, vascular, and dermal structures and arise from a complex interaction of environmental and genetic factors. NTDs have a familial pattern with an increased recurrence rate. Approximately 2% to 16% of infants with isolated NTDs have cytogenic abnormalities. NTDs also occur in infants with other congenital defects. These infants may have normal or abnormal karyotypes. A small number of NTDs occur secondary to teratogen exposure.171
Periconceptional folic acid supplementation significantly reduces the risk of NTDs in women with a history of a previous infant with an NTD and in the general public.161,171 Current recommendations are that women of childbearing age consume 0.4 mg of folic acid daily, with higher recommendations (4 mg beginning 3 months before pregnancy and lasting through the first trimester) for women who have previously had a child with an NTD.8 Research continues regarding folate-related and other metabolic pathways and NTDs, the role of gene polymorphisms, and the roles of other nutrients such as vitamin B12.50,171
α-Fetoprotein leaks into the amniotic fluid and maternal serum in infants with open NTDs. Maternal serum screening at 16 to 18 weeks is used to identify infants at risk for NTDs, with follow-up ultrasound screening for diagnostic confirmation (see Chapter 3).
Anencephaly is due to failure of the neural tube to fuse in the cranial area. Because this area forms the forebrain, anencephalic infants have minimal development of brain tissue above the midbrain. Tissue that does develop is poorly differentiated and becomes necrotic with exposure to amniotic fluid. This results in a mass of vascular tissue with neuronal and glial elements and a choroid plexus with partial absence of the skull bones.173 Because anencephaly arises from failure of the neural tube to close cranially, the insult occurs at or before 24 days.249
Encephaloceles also arise from failure of closure of part of the caudal portion of the neural tube and occur no later than 26 days.249 About 70% to 80% of these defects occur in the occipital region, with the sac protruding from the back of the head or base of the neck.23,249 The protruding sac varies considerably in size, but the size does not correlate with the presence of neural elements. Ten percent to 20% of encephaloceles contain no neural elements.249 Hydrocephalus occurs in up to 50% of occipital encephaloceles due to alterations in the posterior fossa.249 Hydrocephalus may be present at birth or develop after repair of the defect. Encephaloceles may occur in association with meningomyelocele.
Spina bifida is a general term used to describe defects associated with malformations of the spinal cord and vertebrae that usually arise from defects in closure of the caudal neuropore. Approximately 80% occur in the lumbar area, which is the final area of neural tube fusion. Thus, spina bifida, arising from defects in primary neurulation, occurs at or before 26 days.249 Defects range from minor malformations to disorders that result in paraplegia or quadriplegia and loss of bladder and bowel control. The degree of sensory and motor neurologic deficit depends on the level and severity of the defect. The two major forms of spina bifida are spina bifida occulta and spina bifida cystica.
Spina bifida occulta (occult dysraphic state) is a vertebral defect at L5 or S1 that arises from failure of the vertebral arch to grow and fuse.23,246 These are defects of secondary neurulation during formation of the caudal portion of the spinal cord. Most people with this defect have no problems and the defect may be unrecognized. A few have underlying abnormalities of the spinal cord or nerve roots, which are manifested externally by a hemangioma, dimple, tuft of hair, or lipoma in the lower lumbar or sacral area.
With a meningomyelocele, the most common (80% to 90%) form of spina bifida cystica, the sac contains spinal cord or nerve roots in addition to meninges and CSF. During development, nerve tissues become incorporated into the wall of the sac, impairing differentiation of nerve fibers.23 These infants have a neurologic deficit below the level of the sac. Most infants have hydrocephalus and an Arnold Chiari malformation in which the medulla protrudes downward below the foramen magnum and overlaps the spinal cord.246
Myeloschisis is a severe defect that occurs no later than 24 days.249 With this disorder there is no cystic covering; the spinal cord is an open, exposed, flattened mass of neural tissue. These infants have a high mortality rate and significant neurologic deficits and are at great risk for infection. This defect can involve the entire length of the spinal cord and can occur in association with anencephaly.23
Intrauterine repair of meningomyelocele has been associated with short-term benefits including reversal of hindbrain herniation, which may enhance brain growth and reduce hydrocephalus. Some studies have found improved lower extremity function; other have not.33 However, intrauterine repair is also associated with risks, including increased perinatal mortality, and most all infants are preterm.2,171 A large trial randomizing infants to prenatal (before 26 weeks) or postnatal repair with follow-up to 30 months was recently completed.2 Intrauterine surgery significantly reduced the need for shunting after birth and improved outcomes at 30 months, but was associated with an increased risk of preterm delivery and uterine dehiscence at delivery.2
Disorders of ventral induction are thought to arise no later than 5 to 6 weeks’ gestation. Disorders of forebrain development often have concurrent facial anomalies and include holoprosencephaly (failure of the brain to divide into 2 cerebral hemispheres) and holotelencephaly (see Table 15-6).
Fetal neurodevelopment
Neuronal proliferation
Neuronal proliferation (neuronogenesis) is a period of massive production of neurons and their precursors. The maximal rate of neuronal proliferation occurs between 12 and 18 weeks’ gestation.249 During proliferation the walls of the neural tube thicken, forming layers. The ependyma is the interior lining of the neural tube that later becomes the lining of the ventricles and the central canal of the spinal cord. In the subependymal layer the neurons and glial cells of the CNS are formed in the ventricular and subventricular zones of the germinal layer (germinal matrix).249 Neuronal proliferation begins at 6 weeks in the ventricular zone and at 7 weeks in the subventricular zone; both zones reach their peak proliferation by 12 weeks.39 In conjunction with the proliferation of neurons, glial cells also increase in number; however, with the exception of radial glial cells, the peak in glial cell numbers occurs roughly from 5 months’ gestational age through the first year of life.249 Radial glia have two major functions. First they serve as guides during neuron migration (see next section) and secondly they are neuronal progenitors in the ventricular zone.249 Thus neurons arise from both neuronal and radial glial cell progenitors. Although most of the neuronal complement is formed early in fetal life, a few areas, notably the cerebral subventricular zone and the external granular layer of the cerebellum, continue to acquire neurons during the early months after birth (see Development of the Cerebellum).249 This pattern of neuronal formation produces a particular vulnerability in the preterm infant. In late gestation the ventricular and subventricular zones disappear almost completely (a subventricular zone remnant persists to adulthood) and proliferation shifts to intermediary and subplate zones for continued production of glial cells.39
Alterations in neuronal proliferation can lead to increases or decreases in number and size of cells in the brain and associated structures. Micrencephaly arises from a decrease in size (micrencephaly vera) or number (radial microbrain) of neuronal-glial stem cell units.246,249 Micrencephaly vera may be due to unknown causes; have a familial basis; or be associated with teratogens such as alcohol, cocaine, radiation, and maternal phenylketonuria.249 Excessive proliferation (macrencephaly) may also be due to unknown causes, have a familial basis, or occur with growth disturbances (e.g., achondroplasia or Beckwith-Wiedemann syndrome) and chromosomal disorders (fragile X and Klinefelter syndromes). Macrencephaly is also seen with neurocutaneous syndromes (such as multiple hemangiomas and neurofibromatosis) that involve excessive proliferation of cells within the central nervous system and of mesodermal structures.249
Migration
Once formed, neurons travel from the germinal layer in the subependymal region below the lateral ventricles to the areas of the nervous system where they will further differentiate, forming the gray matter, and take on unique and individual functions.249 The bulk of migration occurs from 10 to 20 weeks’ gestation (usually completed by 22 weeks; Figure 15-7).53,167,249 Two types of neuron migration are seen in the cerebrum and cerebellum: radial and tangential. Radially migration accounts for much of the cortex and involves radial glia, which appear by 10 weeks’ gestation (Figure 15-8).115,167,249 The nuclei of the radial glia are in the germinal matrix. The basal process of each cell is attached to the ventricle and the apical process is attached to the pia matter.115 These processes extending from the radial glia guide the neuron to its respective site. Radial glia also act as progenitors for neuron and astrocyte generation.249 Migration is mediated by signaling proteins, surface molecules, and receptors on both the neurons and the radial glia.249 Cajal-Retzius cells in the external cortical layer secrete reelin, an extracellular matrix glycoprotein. Reelin is thought to exert a stop signal for the migrating neurons.167,249 Radial glial–assisted migration also occurs in the cerebellum. Cortical and cerebellar neurons migrate tangentially without radial glia guidance.167
The neocortex develops in six zones, which are, from innermost to outermost, the ventricular zone, subventricular zone, intermediate zone, subplate, cortical plate, and marginal zone. Neurons forming in the ventricular and subventricular zones migrate through the cortical plate to form more and more superficial layers. The initial neurons migrate toward the cerebral walls to form the preplate. The next neurons migrate to areas deep within the cortex, whereas later neurons migrate further to the surface of the cortex. As a result, neurons formed early come to lie in deeper layers of cortex and subcortex; those formed later end at more superficial layers.249 Thus neocortex formation follows an “inside-first-outside-last” pattern, except for the early neurons of the subplate.198,249 The subplate and marginal zone are transient structures that develop in parallel with the cortical plate, and then regress after the cortex is formed.167 Most cortical neurons have reached their sites by 20 to 24 weeks’ gestation.249
The subplate zone and subplate neurons are critical structures in neocortex development. The roles of the subplate are listed in Table 15-7. The subplate is the first place where synapses form within the cortex and receive input from thalamic and other regions.40 The subplate, located just below the developing cortex, serves as a “waiting room” or temporary site for thalamocortical and corticocortical projections and helps guide axons to cortical and subcortical targets and in establishing functional connections between brain regions.48,53 For example, thalamic fibers enter the subplate area before their target neurons are in place and transiently form synaptic connections with subplate neurons.249 These are held in the subplate until the cortical plate is ready.
Table 15-7
Major Roles of Subplate Neurons
Serve as sites of synaptic contact for “waiting” thalamic, commissural, and association (corticocortical) afferents
Establish a functional link between waiting afferents and their cortical targets
Provide axonal guidance into cerebral cortex for the ascending afferents
Facilitate cerebral cortical organization and synaptic development
Provide “pioneering” axonal guidance for efferent projections from the cortex to subcortical targets, e.g., thalamus
From Volpe, J.J. (2009). The encephalopathy of prematurity—brain injury and impaired brain development inextricably intertwined. Semin Pediatr Neurol, 16, p. 172.
Subplate formation begins at 12 weeks, and reaches its maximum size at 27 to 30 weeks.49,53,252 Thalamic afferents develop at 12 to 16 weeks and reach the subplate by 20 to 24 weeks.118,252 Between 24 and 32 weeks axonal growth to the subplate and cortex increases and thalamocortical afferents move from the subplate to the cortex. Callosal and corticocortical axons enter the subplate from 24 to 32 weeks and enter the cortex from 32 to 26 weeks as the subplate begins to regress.249,252 Thus the subplate is “a significant reservoir of functional connectivity in the preterm infant.”53 The subplate is vulnerable to perinatal injury in these infants.
The subplate undergoes programmed cell death after birth, although subplate neurons remain as interstitial neurons.128,249 Interstitial neurons are embedded in superficial white matter. Alterations in interstitial neurons or in their role in cortical circuitry may play a role in some forms of schizophrenia.128
Disorders of migration alter gyral development and lead to hypoplasia or agenesis of the corpus callosum. Gyral development is most prominent during the last 3 months of gestation (see Figure 15-6,), with the most rapid increase between 26 and 28 weeks. The increased gyri result in a change in head shape (from oval to biparietal prominence). Gyral disorders arise secondary to inborn errors of metabolism, such as fatty acid oxidation defects, chromosomal anomalies, and exogenous insults, especially in preterm infants.172,246,249
Organization
Organization refers to the processes by which the nervous system takes on the capacity to operate as an integrated whole. This phase of neurodevelopment begins at approximately 6 months’ gestation (see Figure 15-7) and extends many years after birth, and probably continues into adulthood. Neuron growth and connections lead to development of sulci and gyri (see Figure 15-6), with a brain growth spurt seen from 26 to 30 weeks. With increasing organization, fetal and infant behaviors become more complex.6,249 Alterations in organization are seen in infants with Down, fragile X, and Angelman syndromes; periventricular leukomalacia (PVL); inflammation and infection; and hypothyroxinemia.199,200,249 Injury to the cortex and subcortical area white matter can lead to disorders such as cerebral palsy. Focal lesions in the immature brain can lead to reorganization of sensory, motor, and related tracts.40
During the period of organization, six processes occur.249 The first is differentiation and development of subplate neurons (see Migration). These neurons migrate to cortex early and guide ascending and descending projections to target neurons. Subplate neurons provide a connection site for axons ascending from the thalamus and other sites, until the neurons that these axons will eventually connect with have migrated from the germinal matrix. The subplate reaches its peak from 22 to 34 weeks.249
The second and third processes involve arrangement of cortical neurons in layers and arborization, wherein the dendrites and axons undergo extensive branching. This latter process is sometimes referred to as the “wiring of the brain” (Figure 15-9). The increase in cellular processes and in the size of the neuronal field is prerequisite for communication throughout the nervous system. This process is followed by the formation of connections or synapses between neurons, the fourth component of organization. The intracellular structures and enzymes that will produce neurotransmitters also develop at this time. Connections between cells are critical for integration across all areas of the nervous system. Throughout development, synapses continue to restructure; this process is believed to be the basis for memory and learning. Impulse conduction provides for functional validation of connections. Evidence suggests that use of conduction pathways may increase or alter connections.249 Characteristics of early neuronal activity include poor spontaneous neuronal activity, slow conduction velocity, slow synaptic potentials, and synaptic transmission uncertainty. Immaturity of neurotransmitters and hypersensitivity of NMDA receptors increases the risk of receptor overstimulation with hypoxic-ischemic events (see Hypoxic-Ischemic Encephalopathy).
Synaptogenesis is mediated by excitatory neurotransmitters such as glutamate.73,132 Glutamate acts on N-methyl-d-aspartate (NMDA) receptors to enhance neuronal proliferation, migration, and synaptic plasticity. During the brain growth spurt, NMDA receptors are hypersensitive. Blockage of these receptors in animal models by substances such as ethanol leads to apoptosis.73,115,132,211,249 Other substances, such as erythropoietin, may also be important in enhancing brain organization and for neuroprotection.130,160,211,258
The fifth organizational process entails reduction in the number of neurons and their connections through the death of up to half of the original neurons as well as regression of many dendrites and synapses.249 Neuronal survival has been likened to survival of the fittest, because neurons compete for resources such as nutrients, electrical impulses, and synapses. Neuronal death assists in elimination of errors within the nervous system. The appropriate number of neurons and their connections is retained while neurons that are improperly located or fail to achieve adequate connections are eliminated. Numbers of neurons are maximal in the fetus and survival is dependent on neurons or groups of neurons getting appropriate numbers of connections. From 8 months’ gestation to adulthood, numbers of neurons and synapses decrease. For example, there are an estimated 620,000/m3 neurons in the visual cortex at 7 months’ gestation versus 100,000/m3 at term and 40,000/m3 in the adult.150
Cell death and selective elimination of neuronal processes is important in adjusting the size of individual neurons to their anticipated need and in brain plasticity in infants. In the developing brain, neuronal processes targeted for elimination can be saved if they are needed, for example, because of damage to other processes in order to preserve functional ability. Excitatory neurotransmitters, such as glutamate, mediate neural development and organization by acting on NMDA receptors.23
The final organizational process is differentiation of glia from the general precursor cells into specific types and glia proliferation. The increase in glia begins at approximately 30 weeks’ gestation, continues into the second year, and then plateaus. There are three main types of glia: myelin-building (oligodendrocytes and Schwann cells), guiding (radial glia [for neuron migration], and Schwann cells), and “clean-up” (astroglia and microglia). The astroglia and microglia remove waste and dead tissue and occupy space left by neurons that have died, among other functions. Astrocytes also provide support for neurons. Astrocytes undergo rapid proliferation from 24-32 weeks (peak 26 weeks).226,249 These glia are found primarily in deep cortical layers and white matter. They assist with axonal guidance, growth, brain structural development, and functioning of the blood-brain barrier.226 Astrocytes can release transmitters (such as glutamate) to send signals to neighboring neurons. Each astrocyte may interact with several neurons and hundreds to thousands of synapses to integrate information.226,249 Microglia are the brain macrophages and immune cells. They also have a role in brain development with increased numbers seen in the third trimester. Activated microglia with white matter injury can lead to cellular injury initiated by ischemia and inflammation (mediated by reactive oxygen species, cytokines, and glutamate).226,249 Oligodendrocytes produce myelin in the CNS. During the premyelinating period (before 32 weeks’ gestation), oligodendrocytes are especially vulnerable to hypoxic-ischemic injury. Damage to these glial cells is a prominent feature in brain injury in preterm infants (see White Matter Injury).253 Schwann cells produce myelin for the peripheral nerves. Schwann cells also act as guiding glia following peripheral nerve injury to guide regenerating axons to their targets.249
Myelinization
Myelinization refers to the laying down of myelin, the lipoprotein insulating the covering of nerve fibers. Myelinization increases the speed of transmission along axons by 100-fold.150 This process occurs in both the central and peripheral nerve systems. Glia migrate to locations along the developing nerve fibers. Myelin is formed as the glia (either Schwann cells in the peripheral nervous system or oligodendroglia in the CNS) wrap around the nerve fiber. The glia’s cellular membrane, once wrapped around the fiber, fuses to become myelin.
Myelinization begins during the second trimester and continues into adulthood.249 The process of myelinization occurs at differing times in areas throughout the nervous system. For example, myelinization of somatosensory and auditory areas is nearly complete by term, whereas prefrontal lobe myelinization is not completed until after 20 years.150 Myelinization of the forebrain is most rapid after birth. Myelinization first begins in the peripheral nervous system, with motor fibers becoming myelinated before sensory fibers. In the CNS, myelinization of sensory areas precedes that of motor areas, with the primary sensory areas becoming myelinated relatively early in development. Incomplete myelinization does not prevent function. However, because incomplete myelinization affects nerve conduction, it does alter the speed of impulse conduction. The order of myelinization parallels overall nervous system functional development and generally precedes mature function.173 Areas of the brain that support higher-level functions, such as cognition and learning, myelinate later in life.249 Some brain areas continue to lay down myelin well into adulthood. Because myelin is a lipoprotein, dietary adequacy of fats and protein is important for normal myelinization. Disorders of myelinization are seen with amino and organic acidopathies, hypothyroidism, undernutrition, and perinatal insults such as PVL.249
Cerebellar development
The cerebellum is one of later brain structures to mature. The cerebellum undergoes a rapid growth spurt from 24 to 40 weeks with a fivefold increase in volume reflecting underlying histogenic and cytogenic changes; the cerebellar cortex increases more than 30-fold during this period.36,146 Prior to 20 weeks two proliferation zones are established that give rise to the major cerebellar structures. Between 20 and 40 weeks increased external surface foliation is seen with development of the external granular layer, Purkinje cell (neuron) differentiation, and migration.36,146 The lateral hemispheres of the cerebellum are involved in cognitive and motor function; the middle layers in regulation of emotion, social behavior, and affect.148 The cerebellum is important in cognition and acts as a node in distribution of neural networks with interconnections with thalamus, parietal, and prefrontal cortex.184 Cerebellar damage (see Cerebellar Injury in Preterm Infants) can alter language development, behavioral function and cognitive function.36,146,148,184,249
Development of specific systems
Autonomic nervous system.
The autonomic nervous system has both central and peripheral components. Peripheral portions develop from neural crest cells. Ganglia are formed from neural crest cells that migrate beyond the neural tube. Sympathetic ganglia are derived from neural crest cells that collect along either side of the developing spinal cord at about 5 weeks’ gestational age.173 The parasympathetic ganglia and plexi also form by migration.173 Once they reach their respective sites, neurons in the autonomic ganglia continue to differentiate. Nerve fibers, originating in the cord, grow out and make connections with the ganglia. The autonomic nervous system regulates many endocrine functions through nerve impulses. The embryonic origins of some autonomic end organs demonstrate a structural basis for these regulatory actions. The pituitary, a primary source of autonomic regulation, is derived from two forms of tissues. The adenohypophysis is formed from ectoderm and the neurohypophysis is formed from neuroectoderm. Similarly, the adrenal gland is derived from two types of tissues. The adrenal cortex is derived from mesoderm, whereas the medulla, which secretes catecholamines, is derived from neuroectoderm (specifically neural crest cells) and is controlled by the sympathetic nervous system.
Peripheral nervous system.
The peripheral nervous system is derived from the neural crest. Through migration and specialization, cells of the neural crest develop into cranial, spinal, and visceral nerves and ganglia. Neural crest cells are precursors of the adrenal medulla chromaffin cells that secrete epinephrine.173 Support cells of the peripheral nervous system also derive from the neural crest. Nerve fibers that eventually innervate skeletal motor fibers emerge from the spinal basal plate, combine into bundles to form the ventral root, and migrate to the developing motor fibers.173 Sensory fibers form the dorsal root in a similar manner.
Functional development
Sensory abilities
Generally the somatic sensory and special sensory systems develop in the following chronologic order: touch, proprioception, vestibular, chemoreception (smell and taste), hearing, and vision.92 As noted in the section on Myelinization, in the peripheral areas, motor fibers myelinate before sensory fibers, whereas in the central nervous system sensory fibers myelinate before motor fibers. Thus in terms of rate of impulse transmission, differences in myelinization are one basis for limited integration of sensory and motor actions in the fetus.
The early fetus has been shown to respond to touch around the mouth at around 2 months of gestational age; hands become touch sensitive by 10 to 11 weeks.245 Touch in utero entails contact with amniotic fluid that is approximately at body temperature and contact with body parts or the wall of the uterus. Maternal movement and buoyant amniotic fluid provide rich vestibular stimulation for the fetus in utero.
Nociceptors appear at 6 to 8 weeks’ gestation, initially around the mouth; by 11 weeks in the face, palms, and soles; by 15 weeks in the trunk, arms, and legs; and by 20 to 22 weeks are abundant throughout the fetus (Table 15-8).49,118,152,167,245 The fetus can process pain at the subcortical level before cortical structures are in place.245 Sensory fibers to dorsal interneuron areas may develop as early as 6 weeks with development and differentiation of dorsal horn afferents beginning at 12 to 13 weeks.1,152 Afferent synapses in the dorsal spinal cord and peripheral receptors start as early as 8 weeks’ gestation.152 Connections to the thalamus begin at 14 weeks and are complete by 20 weeks; thalamocortical connections are seen by 13 weeks and are more developed by 26 to 30 weeks.49,152,1 These connections are myelinated by 29 weeks.245 By at least 24 weeks noxious stimuli cause a response in the primary sensory cortex. Completion of these connections stimulates development of axons and synaptogenesis with selective elimination of excess connections and cell populations.49
Table 15-8
Anatomic and Functional Development of the Different Parts of the Pain System
PART OF THE SYSTEM | DEVELOPMENTAL EVENT | TIMING (WEEKS) |
Nociceptors | Nociceptors appear (starting around the mouth and later developing over the entire body). | 7-20 |
Peripheral afferents | Synapses appear to the spinal cord. | 10-30 |
Spinal cord | Stimulation results in motor movements. | 7.5 |
Spinothalamic connections are established. | 20 | |
Myelinization of pain pathways. | 22 | |
Descending tracts develop. | Postnatally | |
Thalamocortical tracts | First axons appear to the cortical plate. | 20-22 |
Functional synapse formation of the thalamocortical occurs. | 26-34 | |
Cerebral cortex | Cortical neurons migrate (cortex develops). | 8-20 |
First EEG burst may be detected. | 20 | |
Symmetric and synchronic EEG activity appears. | 26 | |
Sleep and wakefulness patterns in EEG become distinguishable. | 30 | |
Evoked potential becomes detectable. | 29 |
From Vanhatalo, S. & van Nieuwenhuizen, O. (2000). Fetal pain? Brain Dev, 22, 146.
Proprioception, which is involved with perception of joint and body movement and the position of the body, is interrelated with tactile and vestibular receptors. Vestibular sensation is a form of proprioceptive sensation involved in balance and postural control. Vestibular sensation, mediated by receptors in the ear, detects changes in direction and rate of head movement. Vestibular receptors mature by 14 to 15 weeks.136
Smell is mediated by three groups of receptors that bind fragrant molecules: (1) main ciliated neuroreceptors (seen by 11 weeks), (2) trigeminal nerve endings (respond by 7 to 10 weeks), and (3) vomeronasal (appear by 5 to 6 weeks and are maximal by 20 weeks).145,256 Olfactory marker protein is present by 28 weeks.43 Odor molecules in amniotic fluid stimulate the fetal smell receptors by the third trimester.256 The fetus may detect aromatic substances and flavors from the maternal diet in amniotic fluid. This stimulation may have a role in programming later dietary preferences.16,43,118,133,164 Responsiveness to odors is observed in preterm infants beginning at approximately 28 weeks and is readily documented by 32 weeks’ gestation in most infants.43
Taste occurs by activation of the taste buds and stimulation of the trigeminal nerve. Taste buds appear by 7 to 8 weeks and are morphologically mature by 13 to 15 weeks.43 Taste receptors are present by 16 weeks, reaching adult numbers by term.136 The fetus ingests amniotic fluid (see Chapter 3). Although variations occur in the composition of the amniotic fluid, it is unknown to what extent the fetus experiences taste. However, injection of a sweet substance increases fetal swallowing, whereas a bitter substance decreases swallowing.43 Preterm infants respond to sweet taste by as early as 24 weeks and consistently by 28 to 32 weeks.43
The structures of the auditory system, including the inner ear and cochlea, are mature enough to support hearing by approximately 20 weeks’ gestation; fetal hearing begins at 24 to 25 weeks.93 At this point the fluid spaces in the cochlea are still poorly developed, the basal membrane is thick, hair cell innervation and arrangement are immature, and sensitivity to sound is poor. Sound sensitivity matures rapidly from 24 to 35 weeks.255 The tympanic membrane is similar to that in the adult by 28 weeks.255 The cochlea matures slowly during the third trimester, undergoing “tuning” during this period. Tuning of the cochlea so that areas are frequency-specific involves mechanical maturation of the basilar membrane and outer hair cells.255 Tuning begins in the base (high-frequency sounds) and proceeds toward the apex (low-frequency sounds). Changes in the sound environment, as occurs with preterm birth, may alter this process.
Auditory evoked potentials can be recorded as early as 25 to 26 weeks’ gestation, but are not reliable until after 28 weeks.91,93,255 The fetus in the third trimester can discriminate between maternal live voice and tape-recorded voice and familiar versus novel sounds.85 Responses to sound are observed in preterm infants at approximately 25 to 28 weeks’ gestational age.91 The preterm infant can be observed to orient to sound, with evidence of arousal and attention. The hearing threshold decreases with gestational age. For example, in infants of 28 to 34 weeks’ gestational age, the hearing threshold is approximately 40 decibels, whereas in term infants the hearing threshold is approximately 20 decibels.
Intrauterine recordings demonstrate that sounds in the external environment are audible to the fetus. High-frequency sounds are attenuated, whereas low-frequency sounds are not. External sound is reduced by about one-half its strength by the time it reaches the fetus.133 For example, a 90-dB sound in a midrange frequency would be decreased to 45 dB by the time it reached the fetus.85 The intrauterine auditory environment includes sounds within the mother such as breathing, movement of blood through the umbilical cord, and intestinal peristalsis and differs significantly from the sound environment of the preterm infant.83 Hearing in preterm infants of different gestational ages is summarized in Table 15-9.
Table 15-9
Development of Hearing in Preterm and Term Infants
GESTATIONAL AGE | ANATOMIC AND FUNCTIONAL DEVELOPMENT |
Preterm infants <28 weeks |
Compiled from references 22, 31, 80, 92, 93, 136, 191.
Formation of the eyes begins during embryonic development. The fovea can be identified by 14 weeks’ gestation but maturation of the foveal region is not complete until late childhood.169 Rod differentiation and retinal vascularization begin by 25 weeks’ gestation and myelinization of the optic nerve begins at 24 weeks.80,91 The choroid vasculature (vessels that underlie the retina and pigment epithelium) are mature by 21 weeks. Retinal vessels that supply the inner retina develop in parallel with the retina. These vessels begin to develop from the optic disk at the base of the hyaloid artery and extend peripherally to the ora serrata.169 Growth is particularly rapid from 24 to 28 weeks and, then continues at a slower pace. Zone III, the most peripheral retina, has minimal vessel development in preterm infants at 29 weeks; by 36 weeks about half of the vessels have developed, and almost all are developed by 45 to 49 weeks.169
The neurons forming the visual cortex are in place at 26 weeks. Between 28 and 34 weeks’ gestation, visual neuronal connections and processes undergo rapid development.91 Visual evoked potentials can be recorded between 25 and 30 weeks.91 Visual attention begins at about 30 to 32 weeks’ gestation, although it is fleeting at this age. Development of vision in preterm infants at different gestational ages is summarized in Table 15-10.
Table 15-10
Development of Vision in Preterm and Term Infants
Adapted from Glass, P. (1999). The vulnerable neonate and the neonatal intensive care environment. In G.B. Avery, M.A. Fletcher, & M.G. MacDonald (Eds.), Neonatology: Pathophysiology and management of the newborn (5th ed.). Philadelphia: Lippincott Williams & Wilkins.
Motor abilities
The development of motor activity in the fetus is a function of both neural and muscular maturation. Muscle cells develop from mesoderm. Innervation during development is critical for muscle fiber development.249 Muscle cells, as well as neurons, undergo migration and differentiation during development. Mature myocytes are present at approximately 38 weeks; muscle cells increase in size postnatally.249
The pattern of fetal motor development includes differences in both emergence of muscle tone and the amount of movement over time.91 Development of muscle tone follows a caudocephalad and distal-proximal pattern; that is, lower extremities precede upper extremities and extremities precede axial or truncal muscle tone. Motor development is associated with increased flexor tone, with lower extremities demonstrating flexion before upper extremities. In the lower extremities, passive flexor tone is noticed at 29 weeks’ gestation and active flexor tone becomes apparent at 31 weeks. Tone in the upper extremities develops later, with flexor tone demonstrated in the upper extremities by 34 weeks.10 Active tone develops before passive tone; that is, muscle tone is seen during movement or action before resting muscle tone. Both tone and flexion increase with gestational age. Motor movement also shows increasing coordination with gestational age, with less tremor, smoother movement, and more coordination.91 More complex motor movements are observed after 24 weeks’ gestation.91 Muscle tone is used as one criterion in scoring gestational age.
Muscle tone is limited before 28 weeks’ gestation. By 32 weeks, flexor tone can be observed in the lower extremities; it is observable in the upper extremities by 36 weeks. The term infant demonstrates flexion.249 Movement changes with development. At 28 to 32 weeks’ gestation, movement is slow and may appear uncoordinated, with flailing- or writhing-type movements.249 By 32 weeks’ gestation, flexion movements are somewhat more coordinated.249 Neonates of this age can turn their head, but head control is lacking. With continued development there is increasing strength: alternating movements may be seen in the lower extremities, and head control improves.249 Fetal movements are episodic and cyclical with cycles increasing in duration and regularity from midgestation to term.186
During pregnancy the pattern of fetal movements is one of increasing frequency of movements, followed by a reduction in movement close to term. Spontaneous movements begin at 7 to 8 weeks’ gestation with slow flexion-extension of the vertebral column and passive displacement of the extremities, followed by discrete limb movements at 9 weeks. The earliest reflex is tilting of the head with perioral touch (7.5 weeks); legs demonstrate reflexive movement by 14 weeks.245 The development of movement involves twitching-type movement before 10 weeks, followed by independent limb movement (10 to 12 weeks); hand to face movement (12 to 13 weeks); limb, head, and torso movement (12 to 16 weeks); sucking on fingers (15 weeks); and increasingly complex hand, face, and respiratory movements after 24 weeks.91,249
The developing motor activity of the fetus reflects integration of nervous system activity as well as general fetal well-being. Changes in fetal movement may reflect placental insufficiency, hypoxemia, or other evidence of fetal distress. Daily fetal movement monitoring has been used as a screening tool for fetal well-being during the third trimester (see Chapter 6). In addition to changes in tone and frequency of movement during pregnancy, fetal movements provide evidence of fetal sleep-wake or state patterns.
Fetal state patterns
Although the constancy of the uterine environment may suggest similar consistency in fetal behavior, this assumption is untrue. Fetal behaviors exhibit regular patterns of occurrence, particularly sleep-wake behaviors or behavioral states in the third trimester. In the adult, sleep-wake behaviors demonstrate a diurnal pattern of activity and sleep. Sleep in the fetus and infant does not follow this pattern. Sleep changes with maturation of the central nervous system. Because sleep is qualitatively and quantitatively different in the young compared with adults, fetal and infant sleep-wake patterns are more commonly described as states. In the infant, state is determined by characteristics of heart and respiratory regularity, eyes open or closed, motor activity, and presence or absence of rapid eye movements (REM). Although not all of these parameters can be observed in the fetus, fetal state can be determined by regularity of heart rate, presence of eye movements, and fetal movement.56
In the fetus as well as the infant, motor activity and irregular heart rate occur during REM sleep. Motor movement also occurs during awake periods. During quiet sleep, regular heart rate and minimal motor movement are noted. Alteration of quiet and active periods in fetal movement have been reported as early as 21 weeks’ gestation; however, there is little evidence of regular, rhythmic pattern and limited coordination among state parameters at this time.234 Fetal movement is largely continuous in early pregnancy, but with increasing maturation, quiet periods emerge. For example, at 24 to 26 weeks’ gestation there is almost continual motor activity of the fetal extremities; by 28 weeks distinct periods of quiescence and activity alternate; by 32 weeks’ gestation, alteration of activity and quiet periods becomes more regular and rhythmic.58 Between 28 and 32 weeks (and possibly as early as 27 weeks), heart rate cycles in conjunction with activity/nonactivity cycles are noted.186 By 32 weeks’ gestation, patterns of fetal heart rate, eye movement, and gross body movements also begin to show coordination.248 This periodic function is credited with being a prenatal version of the alteration of REM (fetal state 2F) and quiet sleep (fetal state 1F), representing an underlying rest-activity cycle.196 Duration of state 2F is longer than state 1F. An agitated state (4F), seen infrequently and primarily near term, is associated with decreased time in state 2F.56 The fetal state cycle is approximately 40 minutes long.234 With continuing development, the length of quiet periods and the integration among state behaviors increase.185 These changes continue after birth.