Chapter 37 Neurologic Dysfunction
Neurologic problems are among the most common, severe, and disabling complications that occur after cardiac surgery. The reported incidence varies from 0.4% to almost 80%, depending on how the deficit is defined.1 Roach found that perioperative adverse neurologic events occurred in 6.1% of adults after they had undergone coronary artery bypass graft (CABG) surgery. Half (3.1%) were classified as type 1 injury, including focal injury, stupor, and coma. The other half (3.0%), type 2 injury, had deterioration in intellectual function or memory or had seizures.2 Other investigators have found much higher rates of cognitive decline following cardiac surgery3 and, in a proportion of patients, this can be permanent. Stroke is the second most common cause of death following CABG surgery (after low cardiac output), and cardiac surgery is the most common cause of iatrogenic stroke. The direct economic cost of a stroke ranges from $90,000 to $280,000 over a patient’s life span.1. Various perioperative interventions may reduce the risk of brain injury; however, cardiac surgery is now being performed on people of increasing age and with multiple comorbidities, both of these factors increase the likelihood of neurologic complications.
CLINICAL PRESENTATIONS
Altered Consciousness
The term “altered consciousness” encompasses a range of disorders that extend from an acute confusional state or delirium through coma and brain death. Consciousness can be viewed as a combination of wakefulness and awareness of self and of the environment. Wakefulness requires intact functioning of the ascending reticular activating system (ARAS) and the cerebral cortices. The ARAS extends from the midpons through midbrain and thalamus to the cerebral cortices. Awareness requires multiple functions, such as attention, sensation, perception, memory, motivation, and cognition.4 Multiple descriptive terms (e.g., obtunded, somnolent) are used to describe different levels of altered consciousness. They should be avoided because they are not used consistently, either in the literature or in practice. It is much better to describe consciousness in terms of an objective measure such as the Glasgow Coma Scale (GCS; Table 37-1).5
Verbal Response | Motor Response | Eyes |
---|---|---|
5 = Appropriate | 6 = Obeys commands | 4 = Open spontaneously |
4 = Disorientated | 5 = Localizes to pain | 3 = Open to command |
3 = Unconnected words | 4 = Withdraws to pain | 2 = Open to pain |
2 = Only sounds | 3 = Abnormal flexion to pain | 1 = No response |
1 = Nothing (if extubated) | 2 = Abnormal extension to pain | |
1 = No response |
Total = V + M + E = 3-15
From Teasdale G, Jennett B: Assessment of coma and impaired consciousness: a practical scale. Lancet 2: 81-84, 1974.
Delirium or Acute Confusional State
The four key characteristics of delirium are (1) disorganized thinking, (2) impaired attention, (3) altered level of alertness, and (4) acute onset and a fluctuating course. Additional features are disordered sleep-wake cycles, perceptual disturbances (especially visual hallucinations), increased or decreased psychomotor activity, and memory impairment.4,6 Many authors use the term “delirium” specifically to describe a florid state that includes disorientation, fear, hallucinations, agitation and, commonly, increased sympathetic nervous activity. Others, including the Diagnostic and Statistical Manual of Mental Disorders, ed. 4 (DSM-IV), use “delirium” to refer to the four characteristics just noted. Because a fluctuating course and variable symptoms are common, it makes more sense to view delirium as a single syndrome that has a spectrum ranging from quiet, sleepy, and confused to agitated, hallucinating, and fearful.6 Delirium usually presents after the patient has been extubated, but it should also be suspected in patients who are combative and agitated as they emerge from sedation.
Depressed Consciousness and Coma
Consciousness is considered to be depressed when excessive sensory stimulation is required for arousal and wakefulness. However, when awake, patients may have normal movements and be able to open their eyes and talk. Depressed consciousness should be described in terms of the level of stimulation required to achieve arousal and whether thought processes appear to be preserved when awake. Comatose patients cannot be roused to consciousness; they are not awake or aware. They do not open their eyes, talk, or move, either spontaneously or on command. In response to painful stimuli, their eyes do not open, vocalization is limited or absent, and movements are reflexive. Coma is due to bilateral cortical dysfunction, lesions in the ARAS, or both. Lesions involving the brain-stem portion of the ARAS are commonly associated with oculomotor and breathing abnormalities.4
Altered consciousness (including delirium, depressed consciousness, and coma) is common after cardiac surgery. The reported incidence varies from 8.4% to 32%, according to whether studies were prospective and whether structured interviews were used.7 Patients who do experience altered consciousness have significantly worse outcomes than do those who do not. The average length of stay in the hospital is almost double (14 days versus 8 days), and mortality rates triple.7 The most common causes of altered consciousness following cardiac surgery are stroke, microembolic injury, metabolic encephalopathy, and hypoxic ischemic encephalopathy; they are discussed subsequently.
Weakness
The most common presentation of weakness early after cardiac surgery is hemiplegia due to stroke. Weakness may also be caused by spinal cord, nerve root, nerve plexus, or peripheral nerve lesions. Patients in intensive care units (ICUs), especially those who have multiple organ dysfunction syndrome (MODS), may also experience weakness caused by critical illness polyneuropathy or myopathy. Classically, weakness is divided into upper motor neuron (UMN) and lower motor neuron (LMN) patterns. UMN weakness results from disorders affecting the cerebral cortex, subcortical white matter, internal capsule, brain stem, or spinal cord. LMN weakness results from disorders affecting the cell bodies of the lower motor neurons in the brain stem nuclei or anterior horn of the spinal cord or the axons of the neurons as they pass to skeletal muscle. UMN lesions are associated with increased tone and reflexes, extensor plantar responses, clonus, and no muscle wasting or fasciculation. LMN lesions are associated with reduced tone, reduced or absent reflexes, flexor plantar responses, muscle wasting, and sometimes fasciculation. However, hypertonicity takes days to weeks to develop and acutely, UMN lesions commonly cause hypotonia. Wasting also takes weeks to develop and fasciculation is a feature mainly of anterior horn cell disease. If they occur, extensor plantar responses and increased deep tendon reflexes typically develop within a few hours and a few days, respectively, of a stroke, and therefore may be clinically useful in the acute setting.
Seizures
The most common types of seizures after cardiac surgery are generalized tonic/clonic seizures and simple motor seizures. Isolated seizures—that is, in the absence of signs of focal brain injury—are uncommon, occurring in only 0.4% of patients after cardiac surgery.2 Simple motor or sensory seizures strongly suggest a structural brain lesion, whereas generalized seizures may occur with or without a structural lesion.
TARGETED NEUROLOGIC EXAMINATION
The goal of the targeted neurologic examination is to determine the likely location of the lesion, and therefore the probable cause, in order to determine further investigations and treatment. A key aim is to differentiate patients who have a structural cause of coma (e.g., due to stroke) from those who have diffuse cortical dysfunction (e.g., due to metabolic encephalopathy). Lateralizing signs suggest a structural lesion, whereas involuntary movements (seizures, myoclonus, asterixis, or a “flapping tremor” on attempted sustained wrist extension), especially if generalized, suggest a diffuse process.4 The targeted examination has three principal components: (1) determination of the level of consciousness; (2) examination of the cranial nerves; and (3) limited assessment of the motor system.
Level of Consciousness
Various levels of consciousness may be distinguished.4 In patients with delirium, assessment of the presence of disordered thinking, memory, and attention may be performed by using a standardized score such as the Confusion Assessment Method for the ICU (CAM-ICU).8 Depressed consciousness should be described in terms of the patient’s spontaneous activity and responses to graded stimulation. The patient should be watched undisturbed for several minutes for body position, motor activity, eye opening, and verbalization (if not intubated). Purposeful movements (e.g., reaching for the endotracheal tube) and comfort positioning (e.g., crossing legs, shifting position) suggest that cortical integration is intact. Response to graded stimulation should be assessed by using the GCS (see Table 37-1). The GCS was initially designed for the assessment of patients with traumatic brain injury, but it has become widely used for evaluating consciousness level in almost all acutely ill patients. It has good interobserver reliability and is a powerful predictor of outcome in a wide range of disorders.9,10 The individual components as well as the total score should always be recorded (e.g., E1, V1, M3, total 5). The motor component is the most powerful predictor of outcome, and it is essential that this be performed and recorded correctly. The patient should be asked to follow commands (e.g., open your eyes, lift up your arm). If unable to respond, painful stimulation (e.g., sternal rub, supraorbital compression) is applied. If the patient makes any sort of flexion response, pain should be applied in the cranial nerve distribution (e.g., supraorbital pressure, squeezing ear lobes) to clearly distinguish flexor posturing, withdrawal, and localization. If asymmetry exists, the best response should be recorded. In ventilated patients, the GCS score should be revised to a maximum of 10, with the annotation that the patient is intubated.
Cranial Nerve Examination
It is usually possible to assess at least some components of the functioning of cranial nerves II through X.4 A full discussion of the significance of all of the possible abnormalities may be found in standard texts on coma.6,11 The core brain stem reflexes to assess are:
Motor Examination of the Limbs
In an awake and cooperative patient, power should be assessed at all major limb joints and may be graded on a 6-point scale (Table 37-2). Although it may not determine the anatomic site of a lesion, assessment of power is helpful in evaluating the progression or deterioration in a patient’s condition. In the upper limb, deep tendon reflexes should be tested at the elbow (biceps and triceps) and wrist (supinator); in the lower limb, they should be tested at the knee and ankle. Reflexes may be recorded on a 5-point scale (see Table 37-2). Increased reflexes are suggestive of a UMN lesion. Reduced or even absent reflexes are consistent with an LMN lesion or a myopathy, but they are a relatively nonspecific finding in ICU patients. The plantar reflex is elicited by stroking the lateral undersurface of the foot; a normal response is flexion of the big toe. An extensor (Babinski) response is seen with UMN lesions, coma, and following generalized seizures. Asymmetries of motor response, tone, power, or reflexes suggest lateralization and make a structural cause of altered consciousness more likely.
Power | |
0 | Complete paralysis |
1 | Flicker of contraction |
2 | Movement possible when gravity excluded |
3 | Movement possible against gravity but not if further resistance added |
4 | Movement possible against gravity and some resistance |
5 | Normal power |
Deep Tendon Reflexes | |
0 | Absent |
+ | Reduced |
++ | Normal |
+++ | Exaggerated |
++++ | Exaggerated with clonus |
Sensory Examination
Accurate examination of sensation is difficult and time consuming and requires an awake and cooperative patient. It is most often performed in patients receiving epidural analgesia (see Chapter 12) but is also important if a spinal cord or peripheral nerve injury is suspected. If an awake patient has a motor deficit or complains of numbness and tingling, a careful sensory examination should be performed. Sensation is carried primarily in two spinal cord tracts: the spinothalamic pathway and the posterior columns. Pain and temperature fibers are carried in the spinothalamic tract. These fibers decussate (cross over) in the spinal cord a few segments above their nerve roots and ascend in the contralateral spinothalamic tract. Vibration and proprioception fibers are carried ipsilaterally in the posterior columns and decussate in the brain stem. Light touch fibers are carried in both pathways and, therefore, this sensory modality is the least discriminatory. Pain and temperature fibers can be tested by pin prick or ice; vibration sense can be tested by placing a 128 Hz tuning fork over a distal bony prominence and having the patient report the moment the tuning fork is deadened by the examiner; light touch may be tested by lightly dabbing (not stroking because this stimulates hairs) cotton wool over the skin.
Sensory deficits usually conform to one or more patterns: (1) dermatome distribution (see Fig. 12-3) due to a lesion involving a nerve root, nerve plexus, spinal cord, or regional nerve block (e.g., epidural); (2) peripheral nerve distribution; (3) glove or stocking distribution secondary to a distal symmetric peripheral neuropathy; (4) a hemisensory distribution due to a lesion involving the spinal cord, brain stem, thalamus, or sensory cortex.
CAUSES OF NEUROLOGIC INJURY FOLLOWING CARDIAC SURGERY
Stroke
Almost all (99%) strokes that occur after adult cardiac surgery are ischemic as opposed to hemorrhagic.12 The majority are due to macroemboli originating from the heart, aorta, or proximal arteries. In a review of 388 patients with stroke after isolated CABG surgery, 62% of cases were embolic; 10% were due to multiple causes; 9% were watershed (due to hypoperfusion, usually caused by a combination of extracranial arterial stenoses and systemic hypotension); 3% were lacunar (due to deep white matter ischemia in brain supplied by penetrating arteries); and 1% were thrombotic. The causes were unknown in 14%.12 When assessed prospectively, stroke is detected within the first 24 to 48 hours after surgery in at least two thirds of patients.7 Later-onset strokes are more common in patients with atrial fibrillation and in those undergoing valve surgery or placement of a ventricular assist device. The likelihood of death following cardiac surgery is three to six times higher in patients who have had strokes than those who have not. The length of stay in the ICU and the hospital is prolonged and more than half require in-patient rehabilitation.7
Clinical Presentation
Stroke can cause altered consciousness, focal neurologic signs, or both. Focal signs include weakness (typically a hemiplegia), visual field defects, and speech defects. Altered consciousness may occur when (1) there is a large hemispheric stroke; (2) there are multifocal infarctions; or (3) there is a posterior circulation stroke. Lesions in the prefrontal lobe, nondominant parietal lobe, or either occipital lobe may also cause altered consciousness and few or no localizing signs. Between 25% and 65% of strokes after cardiac surgery are multiple.13
Approximately one quarter of embolic strokes affect the posterior circulation. The posterior circulation supplies the brainstem, cerebellum, thalami, and occipital lobes. Emboli may affect any of the branches of the vertebrobasilar system. The most common site of obstruction is at the top of the basilar artery. Here an embolus can cause ischemia bilaterally to the thalami, midbrain, and occipital lobes. Clues to a posterior circulation stroke include altered consciousness, cranial nerve palsies, autonomic dysfunction, visual dysfunction, extensor posturing, and abnormal breathing.13
Risk Factors and Prevention
A number of investigators have developed preoperative predictive models to determine the risk for stroke. These models usually include the common risk factors for vascular disease, such as advanced age, previous stroke or transient ischemic attack, hypertension, diabetes, carotid artery stenosis, and peripheral vascular disease.1,7 In addition, they may include factors related to the cardiac procedure, such as the presence of unstable angina, valvular or combined cardiac operations, and aortic arch surgery.
The single most significant risk factor for stroke is atherosclerosis of the ascending aorta. Surgical manipulation of the aorta during cannulation, clamping, placement of proximal anastomoses, and the shear effect of flow from the aortic cannula may lead to embolism of atheromatous material. The presence of extensive (particularly mobile) atheroma of the ascending aorta is a strong predictor of postoperative stroke (and cognitive deficit and encephalopathy).14–16 For instance, in one study of CABG surgery patients, the presence of mobile atheroma in the aortic arch was associated with a 33% stroke rate, compared with 2.7% in those with nonmobile plaque.14 In another study of CABG surgery patients, individuals who had intimal thickness of greater than or equal to 2 mm in the ascending aorta or aortic arch had more intraoperative emboli, more new ischemic brain lesions, and more postoperative confusion than patients who had intimal thickness of less than 2 mm.16
Aortic atheroma can be detected by a number of methods. Intraoperative epiaortic scanning is superior to transesophageal echocardiography (TEE) and direct palpation. Intraoperative palpation by the surgeon identifies approximately one third of lesions seen with epiaortic scanning.17 A protruding or mobile atheroma, which carries the greatest risk for stroke, is even harder to detect by palpation.18 TEE reliably identifies the presence of atheroma in the descending aorta but may miss atheroma in the ascending aorta or aortic arch. However, because it is unusual to have extensive atheroma in the ascending aorta without atheroma also being present in the descending aorta, TEE is a useful screening tool. If atheroma is not identified by TEE, it is very unlikely that there is significant plaque in the ascending aorta, even if this region is not visualized directly. If TEE examination is positive, significant plaque in the ascending aorta is present in about one third of patients.19 CT scanning detects most severely diseased aortas but underestimates mild to moderate disease compared to TEE.20
Epiaortic scanning is used routinely in some centers and selectively in others.21 Although there are clear data that atheroma is an important cause of stroke and that epiaortic scanning can identify atheroma, there are only limited data that show that altering surgical or cardiopulmonary bypass (CPB) technique once atheroma has been identified improves outcome.22 Strategies that may be useful in preventing stroke include employing an off-pump technique for coronary artery bypass graft (CABG) surgery, using sites other than the proximal aorta for arterial cannulation for CPB (e.g., axillary artery), using an on-pump technique that avoids aortic cross-clamping, and using modified aortic cannulas.23
Preexisting cerebrovascular disease is common in patients having cardiac surgery. CT or magnetic resonance (MR) imaging evidence of cerebral ischemic damage that is present before surgery significantly increases the risk for both stroke and cognitive dysfunction after surgery. The largest study, of 421 patients before CABG surgery, showed that half had brain abnormalities on MR scanning; 30% had small brain infarctions, and 20% had multiple brain infarctions.24 Cerebral infarctions on MR scanning are common in asymptomatic elderly patients. Cerebral white matter abnormalities, which are thought to reflect white matter damage resulting from small vessel cerebral arterial disease, are found in nearly one third of patients who have histories of hypertension, diabetes, peripheral vascular disease, and myocardial infarction. This is a rate that is similar to that of the patient population having cardiac surgery. The presence of these white matter lesions preoperatively is significantly associated with the risk for postoperative stroke in patients undergoing aortic valve replacement.25–27
Carotid artery stenosis also significantly increases the risk for stroke. If carotid stenoses are less than 50%, the risk for stroke is 2%; if they are 50% to 80%, the risk is 10%; and if they are greater than 80%, the risk is 11% to 18.8%.1 Preoperative carotid artery screening using Doppler ultrasound scanning is indicated in patients older than 65 years, smokers, and patients who have a history of peripheral vascular disease, left main coronary artery stenosis, previous stroke, or transient ischemic attack.1 The management of patients requiring cardiac surgery who have concomitant symptomatic or high-grade (>80%) asymptomatic carotid stenosis is discussed in Chapter 9.
New-onset atrial fibrillation occurs in 30% of patients undergoing CABG surgery and is associated with a two- to threefold increase in the risk for perioperative stroke.1 Atrial fibrillation is likely to be an important factor in strokes occurring beyond the first 2 to 3 days after surgery. Strategies to prevent and treat atrial fibrillation and the appropriate use of anticoagulation may all reduce the risk of stroke; they are discussed in Chapter 21.
In addition to the strategies listed, which are intended to reduce systemic embolization, a number of techniques related to the conduct of CPB and anesthesia (such as the use of an α-stat pH strategy, avoiding hyperthermia, tight glycemic control, delivery of adequate CPB pressure and flows, and avoiding marked anemia) may also reduce adverse neurologic outcomes (including, but not limited to, stroke) following cardiac surgery.23,28
Imaging
The presence of focal neurologic signs or persistently altered consciousness is an indication to image the brain with either CT or MR scanning (Figs. 37-1, 37-2, 37-3, and 37-4). Scans typically either confirm the presence of an ischemic stroke or strokes or show no acute changes, which suggests metabolic encephalopathy or microembolic injury as the cause of the neurologic deficit.
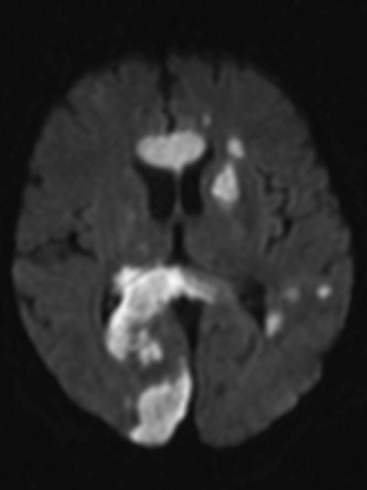
Figure 37.4 Diffusion-weighted magnetic resonance scan showing multiple brain infarcts due to macroemboli.
Brain imaging rarely alters treatment acutely, so it need not be undertaken as an emergency procedure. A practical approach is to perform a scan once the patient is stable from a cardiac and respiratory perspective, usually on postoperative day 1 or 2. An exception to this is the patient who has undergone cardiac surgery within a few weeks of a cerebral ischemic event (usually for endocarditis; see Chapter 35), in which case an urgent scan (as soon as a new neurologic event is suspected) to rule out hemorrhage within a liquefying infarct, is indicated.
CT scans are usually more readily available, easier to perform, and cheaper than MR scans, but they are much less sensitive in detecting strokes. CT scanning detects approximately 60% of strokes in the anterior circulation within 6 hours of the stroke’s occurring if reported by experts in stroke imaging, but it detects only 46% of strokes if scans are reviewed by residents (see Fig. 37-2).29 Strokes usually become more obviously apparent on CT scans 4 to 7 days after the event (see Fig. 37-1). A newer modality of MR scanning, diffusion-weighted imaging (DWI), detects acute changes in the diffusion of water molecules in ischemic tissue and is very sensitive to early detection of stroke (see Fig. 37-3). Using this modality, 91% of strokes can be detected within 6 hours of their occurrence, and there is much better interobserver agreement and less dependence on the experience of the reporter.29 Visualization of the brain stem is also better with MR scanning. To a certain extent, the choice between CT and MR scanning depends on the relative availability of the two imaging modalities in individual institutions. However, if an early CT scan is negative but neurologic signs persist, either the scan should be repeated 4 to 7 days postoperatively, or an early diffusion-weighted MR scan should be performed. For patients with unexpected coma or apparent “locked-in syndrome” (see subsequent material), an MR scan should be obtained because of its improved diagnostic accuracy, particularly in revealing brainstem infarctions.
The results of brain imaging may help to guide the timing of anticoagulation. Patients with “large” infarctions are at increased risk for cerebral hemorrhage if given anticoagulants, particularly within the first 2 weeks after the event. There are few data to guide therapy, but as a general rule, if the indication for anticoagulation is modest (e.g., atrial fibrillation, bioprosthetic valve), anticoagulation should probably be withheld for the first 2 weeks in the presence of a large hemispheric infarction. If the indication for anticoagulation is strong (e.g., a mechanical mitral valve), warfarin should probably be commenced within the first few days after surgery, but with very careful monitoring, aiming for an international normalised ratio (INR) at the lower end of the therapeutic range (see Chapter 10).
It is very likely that our understanding of stroke after cardiac surgery will develop significantly as more sophisticated MR studies (especially DWI) are undertaken. To date, most studies employing DWI have been small. However, they suggest that: (1) as many as two thirds of strokes are silent; (2) strokes may occur in approximately 40% of patients undergoing aortic valve replacement, and they may be much more common in connection with this operation than with either mitral valve or CABG surgery; (3) multiple (macro) cerebral emboli are common in patients with altered consciousness; (4) between 50% and 70% of patients with neurologic dysfunction after cardiac surgery and acute infarcts that are revealed on MR imaging have normal CT scans.25,27,30,31
Microembolic Injury
By convention, microemboli are defined as those with particle sizes less than 200 μm; they block arterioles. Microemboli in the brain have been documented during and after cardiac surgery by retinal fluorescein angiography, transcranial Doppler of the middle cerebral artery, and at autopsy. They are implicated in contributing to the development of postoperative delirium and altered consciousness, generalized seizures, and cognitive deterioration.23 Microemboli are either gaseous or particulate. Air may be introduced into the CPB circuit by incomplete deairing or may be entrained via the venous cannula or perfusion interventions or during surgery in which the left heart is opened. Lipid emboli have been found in the small cerebral vessels of adults who have died after cardiac surgery and in dogs after CPB.32 Post mortem human brain studies show the presence of diffuse small-capillary arteriolar dilatations—known as SCADs—which are thought to be caused by microemboli. In animal experiments, lipid emboli have been found to originate from the reinfusion of blood suctioned from the pericardial space (so-called pump-sucker return).33 Reinfusion of this blood in humans is also associated with neurologic dysfunction after CPB. In addition to avoiding pump-sucker return, the reduction of microemboli may also be achieved by the inclusion of a filter in the arterial line,28 complete deairing of the venous line before commencing CPB,34 and the use of membrane oxygenators as opposed to bubble oxygenators.
Brain injury due to microemboli is not associated with acute changes on CT and conventional MR (including DWI) scans. Functional MR scanning, which measures the oxygenation status of the brain (and therefore metabolic activity) during cognitive tasks, can be used to assess functional deficits caused by microemboli,35 but it is not a practical brain imaging technique for routine clinical use.
Drug and Alcohol Withdrawal
Withdrawal syndromes are an important cause of delirium and a rare cause of seizures following cardiac surgery. The most common substances patients are likely to be withdrawing from are alcohol and sedative-hypnotic drugs. Major and minor withdrawal syndromes are recognized. Symptoms of minor withdrawal include tremors, diaphoresis, hallucinations, seizures, disorientation, fever, tachycardia, and hypertension. Without treatment, minor withdrawal develops in to major withdrawal, the signs of which include severe delirium with increased psychomotor and autonomic activity, hallucinations, disorientation, tremors, marked tachycardia, and blood pressure lability. Withdrawal from benzodiazepines and opioids is discussed in Chapter 4. Alcohol withdrawal can be managed by means of a long-acting benzodiazepine such as lorazepam, administered orally or intravenously. However, in an acute crisis, intravenous midazolam or phenobarbitone are more rapidly effective.
Hypoxic Ischemic Encephalopathy
Hypoxic ischemic encephalopathy is a term used to refer to brain injury that occurs after profound hypoxemia or ischemia. It is most commonly used to describe brain injury after cardiac arrest. However, there is clearly a continuum from complete cessation of cerebral blood flow through lesser degrees of compromise to the watershed patterns of infarction seen with hypoperfusion injury. Although hypoxic ischemic encephalopathy is seen following cardiac surgery, much of the literature relating to prognosis comes from patients who have suffered cardiac arrest in the community, usually secondary to myocardial infarction.
Resuscitation of patients after out-of-hospital cardiac arrest has a very low success rate (<10%), and even in-hospital resuscitation results in less than 20% of patients surviving to hospital discharge.36,37 A significant period of absent or severely compromised cerebral blood flow leads to coma after return of spontaneous circulation. If it occurs, recovery of consciousness usually takes place within 3 days; beyond this time recovery is very unlikely.
Certain features are associated with a uniformly poor outcome following cardiac arrest36,37:
If an invariably poor prognosis is expected, comfort care only should be given in consultation with the family. If the likelihood of a poor outcome is less certain, decisions regarding ongoing treatment should take into account the following factors: (1) the clinical signs; (2) the circumstances of the arrest (witnessed or unwitnessed, time to return of spontaneous circulation, initial rhythm); (3) the patient’s pre-event comorbidities and quality of life; (4) an assessment of the anticipated treatment required; (5) the probability of a good outcome, (6) the patient’s wishes (if known). Survivors of cardiac arrest are often left with severe cognitive disability and become fully dependent. Even moderate disability can result in substantial caregiver burden, as well as the loss of cognitive, emotional, social, and psychological well-being, along with unemployability and social isolation.38–40 Health professionals and the lay public hold similar views concerning severe neurologic disability, usually considering it worse than death.41,42 Further discussion of end-of-life care is provided in Chapter 39.
General management of coma after cardiac arrest is the same as that for other forms of encephalopathy. In addition, moderate induced hypothermia has been shown to improve outcome, according to the results in two randomized trials of adults remaining comatose after outof-hospital ventricular fibrillation cardiac arrest.43,44 The recommendations of the International Liaison Committee on Resuscitation, the American Heart Association, and the European Resuscitation Council are that unconscious patients suffering out-of-hospital, ventricular fibrillation cardiac arrest should be cooled to 32° to 34°C as soon as possible for 12 to 24 hours. It is also suggested that induced hypothermia should be considered in unconscious patients suffering either outof-hospital cardiac arrest due to nonshockable rhythms (asystole, pulseless electrical activity) or in-hospital cardiac arrest. Cooling can be achieved either externally (e.g., using cold-water cooling blankets) or internally (e.g., using infusions of 4°C crystalloid solutions or intravascular cooling catheters). Patients require sedation (with short-acting agents such as propofol to enable rapid neurologic assessment once cooling is stopped) and neuromuscular blockade to prevent shivering. Rewarming should be slow and controlled (no faster than 0.25° to 0.5°C per hour), and hyperthermia should then be avoided.
Postoperative Cognitive Dysfunction
Postoperative cognitive dysfunction may be defined as “impaired verbal or visual memory and language comprehension, impaired abstraction and spatial orientation, and decreased attention and psychomotor processing speed.”45 Patients may demonstrate intellectual blunting, impaired memory and learning, poor concentration, inattention, and difficulty initiating and planning actions, and they are often easily distractible. There may be associated psychiatric or behavioral abnormalities, such as depression, emotional instability, anxiety, hyperactivity, delirium, or even psychosis.
Cognitive deterioration is common following cardiac surgery, although the reported incidence varies depending on how the condition is defined and on the population being studied. In one systematic review of patients undergoing CABG surgery, the incidence (defined as a decline in the performance of at least one standard deviation in at least two tests from a core battery of tests) varied between 14% and 37%, with a mean value of 22.5%.46 Some investigators have found that cognitive changes are relatively minor and that patients return to their baseline functioning over several weeks.47,48 However, in one large, well-conducted trial, cognitive dysfunction following CABG surgery was found to persist and, indeed, worsen over the longer term. Cognitive decline was defined as a decline in performance of at least one standard deviation in at least one of four cognitive domains: (1) verbal memory; (2) abstraction and visuospatial orientation; (3) attention, psychomotor processing speed, and concentration and; (4) visual memory. It was identified in 53% of patients at hospital discharge, in 36% of patients at 6 weeks, in 24% at 6 months, and in 42% at 5 years.3 Some of the differences observed among studies is almost certainly methodologic, for instance, using four different criteria for cognitive decline. Mahanna and colleagues found the incidence varied between 1% and 34% on the same data set.49
Risk factors for postoperative cognitive decline following cardiac surgery include advanced age, noncoronary atherosclerosis (carotid, peripheral vascular), low education level, preexisting neurologic deficit, diabetes, adverse intraoperative and postoperative events, and valve as opposed to CABG surgery.50–52 The presence of multiple small cerebral infarctions on MR scanning in elderly asymptomatic patients significantly increases the risk for developing postoperative cognitive dysfunction.24 Such lesions are also associated with an increased likelihood of developing cognitive impairment and dementia among elderly patients who have not undergone surgery.53
A number of causative factors have been proposed to explain postoperative cognitive decline: cerebral microemboli, cerebral hypoperfusion, the systemic inflammatory response to surgery and CPB, the effects of anesthesia, genetic susceptibility, and depression.54 It is likely that the observed clinical effect represents a complex interplay among these factors. Limited data support the idea that postoperative cognitive function is improved by using a 120-μm aorticline filter during CPB to reduce particulate emboli55 and by using a slow rate of rewarming during CPB.56 In one randomized study, off-pump CABG surgery was associated with a transient benefit compared with on-pump CABG surgery, but no difference in cognitive function was observed at 12 months.57 Various other strategies, such as the use of biocompatible CPB circuits and leukodepleted blood products, may also be beneficial. However, data are limited. Cognitive impairment is a well-recognized complication of cardiac surgery, but it is also seen following noncardiac surgery,48,58 nonsurgical critical illness,59 and percutaneous coronary intervention.60
Spinal Cord Injury
Patients undergoing open or endoluminal surgery involving the descending thoracic aorta are at risk for developing anterior spinal artery syndrome (see Chapter 11). Although the posterior spinal arteries have rich collateral circulation, the anterior spinal artery has a much less efficient collateral circulation, and so the tissue it supplies is more vulnerable to ischemia. The anterior spinal artery supplies the anterior two thirds of the spinal cord. It is formed by branches from each vertebral artery that join to form a single vessel lying in the median fissure of the spinal cord. It is joined by 7 to 10 unpaired radicular branches, usually from the left side. These include cervical, intercostal, and sacral arteries. The largest radicular artery is the artery of Adamkiewicz, which usually arises at the T9 through L2 level, is on the left side in 70% of the population, and supplies the low thoracic and lumbar cord. Occlusion (by an endoluminal stent) or disruption (during open aortic surgery) of the artery of Adamkiewicz can lead to spinal cord ischemia in the distribution of the anterior spinal artery.
Patients with anterior spinal artery syndrome present with paraplegia or quadriplegia. Initially, the affected limbs are flaccid and reflexes are absent. However, over a period of days increased tone, increased deep tendon reflexes, and extensor plantar responses develop. Because the anterior spinal artery supplies the spinothalamic tracts, there is loss of pain and temperature sensation with a discrete sensory level. However, vibration and position sense are maintained because the posterior spinal arteries supply the dorsal columns. Urinary retention occurs if the patient is not catheterized. Strategies to prevent spinal cord injury in these patients, such as cerebrospinal fluid drainage and left-heart bypass, are discussed in Chapter 11.
Neuropathy and Myopathy
Peripheral Nerve Injuries
Peripheral nerve injuries61 are relatively common following cardiac surgery. In one series of 421 patients undergoing CABG surgery, 55 patients (13%) developed 63 new peripheral lesions. By far the most common (26/63) were lesions involving the ulnar nerve or ulnar components of the brachial plexus (C8, T1 nerve roots).62 In a smaller study of 53 patients undergoing cardiac surgery, 20 (37.5%) developed postoperative motor and sensory neuropathies of the upper limb, all in the distribution of the ulnar nerve.63 A likely mechanism of these injuries is traction on the brachial plexus due to sternal retraction, particularly during dissection of the internal mammary artery. Excessive (>90 degree) abduction of the arms may also contribute. Brachial plexus lesions usually recover spontaneously over weeks to months, but in a minority of patients the deficit is permanent. Diagnosis is usually by clinical examination. Nerve conduction studies or somatosensory evoked potentials may also be used to confirm nerve injury, but these tests are highly sensitive and may not correlate with symptoms.
Other frequently encountered lesions include those to the phrenic, recurrent laryngeal, saphenous, and peroneal nerves.62 Phrenic nerve dysfunction can be identified in as much as 70% of cardiac surgery patients; however, complete paresis of a hemidiaphragm occurs in less than 10% of patients.64,65 Phrenic nerve injury is discussed in Chapter 27. A number of mechanisms of injury to the recurrent laryngeal nerves have been proposed. These include traumatic endotracheal intubation, overinflation of the endotracheal tube cuff, trauma during central venous catheterization, sternal retraction, cardiac retraction during open-heart procedures, hypothermic injury caused by ice slush, and direct trauma during surgery involving the great vessels.66 The short course (around the subclavian artery) of the right recurrent laryngeal nerve means it is more prone to traction injury during cardiac surgery than the left nerve; the longer intrathoracic course (around the aortic arch) of the left recurrent laryngeal nerve means it is more prone to direct trauma during surgery involving the aortic arch. Unilateral injury may result in hoarseness; bilateral injury (rare) can lead to inspiratory stridor and respiratory insufficiency. Postoperative hoarseness typically resolves after a few days. In the absence of nerve injury, however, hoarseness may be caused by direct trauma to the larynx. Injury to the recurrent laryngeal nerves may occur in association with phrenic nerve injury.
Critical Illness Polyneuropathy and Myopathy
ICU patients, particularly those with MODS, are at risk for developing critical-illness polyneuropathy (CIP) and myopathy (CIM).67,68 CIP is a functional sensorimotor polyneuropathy. CIM ranges in severity from functional impairment to muscle atrophy and necrosis. The two conditions usually coexist. Risk factors for developing CIP/CIM include prolonged critical illness (particularly that associated with sepsis and acute respiratory distress syndrome), female sex, and the use of corticosteroids and neuromuscular blocking drugs. The first sign of CIP/CIM is usually difficulty in being weaned from the ventilator in a patient with MODS. CIP/CIM may present within a few days of developing a critical illness, but more commonly it develops after more than a week. Patients have a flaccid quadriparesis with reduced reflexes. The limbs and trunk are typically involved, with relative sparing of the nerves and muscles of the face. Sensation is usually abnormal (due to CIP) in a “glove-and-stocking” distribution, but effective sensory examination is usually impossible. The condition ranges in severity from minor limb weakness to profound paralysis in which the patient may be able to move only his or her eyes and facial muscles. Severe cases of CIP/CIM can resemble “locked-in syndrome,” in which the patient is fully conscious but able to communicate only by eye blinks or vertical eye movements. (Locked-in syndrome is typically caused by a pontine or midbrain infarction.) The differential diagnosis of CIP/CIM includes persistent neuromuscular blockade, stroke, and spinal cord injury. Conditions such as myasthenia gravis, myasthenic syndrome, and Guillain-Barré syndrome can also resemble CIP/CIM but are very uncommon following cardiac surgery.
Treatment of CIP/CIM is supportive: appropriate treatment of the underlying critical illness; prolonged mechanical ventilation via a tracheotomy tube (see Chapter 29); good nutritional support (see Chapter 34); and intensive physical therapy. The majority of patients who survive the underlying critical illness slowly recover from CIP/CIM, although in severe cases it may take several weeks.
TREATMENT OF NEUROLOGIC DYSFUNCTION
The primary aim in treating patients with neurologic dysfunction is preventing secondary brain injury. Secondary brain injury occurs because coma and brain stem dysfunction may lead to hypoxemia or hypotension, exacerbating the primary brain injury. Any patient with a GCS less than or equal to 8 should be intubated and ventilated to ensure that the airway is protected and ventilation is adequate. Hyperventilation can contribute to cerebral ischemia (due to cerebral vasoconstriction; see Chapter 1) and has no role in treating brain injury.
Hypoosmolarity, usually manifested as hyponatremia, can exacerbate cerebral edema in patients with acute brain injury, so it should be avoided. Only isotonic intravenous fluids should be used. In cardiac surgery patients, hyponatremia is almost always due to water excess rather than sodium depletion (see Chapter 32). Maintenance fluids should be restricted to no more than a half to two thirds of usual requirements and should be restricted further in the presence of hyponatremia. A good choice for resuscitation fluid is 0.9% sodium chloride. Hyperglycemia is associated with worse neurologic outcome in many settings, including after cardiac surgery, stroke, and cardiac arrest; therefore, blood glucose should be controlled by intravenous insulin, as described in Chapter 36. Fever is common after brain injury and is also associated with worse neurologic outcome. Normothermia, using acetaminophen and active cooling when necessary, should be maintained in patients with acute brain injuries for the first 72 hours.
Nonpharmacologic measures for preventing and treating delirium include frequent orientation (to time, place, and person), avoiding sensory deprivation (early return of glasses and hearing aids), establishing day-night routines and adequate sleep, early mobilization, and the provision of cognitively stimulating activities. Drug treatment of delirium is required if patients become combative and aggressive and place themselves and staff at risk. Antipsychotic drugs are the agents of choice (e.g., haloperidol in small intravenous boluses of 2.5 mg; see Chapter 4). The aim is to use the minimum dose necessary to control agitation and make the patient safe. Because these drugs alter consciousness, they also contribute to the encephalopathy.
Seizure management involves treating any precipitating causes, stopping the seizure, and preventing further seizures. Blood glucose levels should be checked rapidly and dextrose administered if hypoglycemia is present. Other metabolic derangements (e.g., acute hyponatremia, uremia) should be sought and treated appropriately (see Chapters 32 and Chapter 33). Seizures lasting longer than 3 to 5 minutes should be treated as an emergency because they may lead to cardiorespiratory instability, hyperthermia, and metabolic derangements (e.g., metabolic acidosis, hyperkalemia). These effects, in association with the seizure activity itself, can lead to further brain injury. Status epilepticus refers to seizures that are either prolonged or repetitive, with impaired consciousness in the interictal period. The required duration varies but is usually 15 to 30 minutes. Refractory status epilepticus (very rare following cardiac surgery) occurs when seizures continue despite the administration of two anticonvulsants.
An algorithm for treating seizures is shown in Table 37-3. The decision about whether to administer subsequent seizure prophylaxis is determined by the clinical situation. If there are signs of focal pathology or if status epilepticus occurs, prophylaxis is usually indicated. If, however, there is a short seizure (<3 to 5 minutes) that stops spontaneously or after a single dose of a benzodiazepine and there are no focal signs, then it is appropriate to wait and observe for further seizures. Patients should begin to awaken within 15 to 20 minutes after the termination of a seizure. If this does not occur, the patient should be assumed to have nonconvulsive status epilepticus. Careful observation may show subtle clonic movements if seizures are still occurring. A common error in the treatment of status epilepticus (and also a cause of nonconvulsive status epilepticus) is inadequate dosing of effective drugs, especially phenytoin.
Step 1: | Lorazepam 0.05 mg/kg IV |
Alternatives: Diazepam 0.075 mg/kg IV Midazolam 0.05 mg/kg IV |
|
This dose may be repeated once. | |
Step 2: | Phenytoin* 20 mg/kg at a rate of <50 mg/min or Fosphenytoin* 20 mg/kg phenytoin equivalents at a rate of 150 mg/min |
Step 3:*** | Phenobarbital** 20 mg/kg IV |
Step 4: | Midazolam infusion or Thiopentone infusion or Propofol infusion |
If seizures continue after Step 1, proceed to steps 2-4.
* Additional doses of 5 to 10 mg/kg may be given, depending on blood levels.
** Step 3 may be missed in many patients in the ICU, especially if the seizures have been prolonged (>60 to 90 min) or if there are severe systemic complications (e.g., extreme hyperthermia).
1 Eagle KA, Guyton RA, Davidoff R, et al. ACC/AHA 2004 guideline update for coronary artery bypass graft surgery: a report of the American College of Cardiology/American Heart Association Task Force on Practice Guidelines (Committee to Update the 1999 Guidelines for Coronary Artery Bypass Graft Surgery). Circulation. 2004;110:e340-e437.
2 Roach GW, Kanchuger M, Mangano CM, et al. Adverse cerebral outcomes after coronary bypass surgery. Multicenter Study of Perioperative Ischemia Research Group and the Ischemia Research and Education Foundation Investigators. N Engl J Med. 1996;335:857-863.
3 Newman MF, Kirchner JL, Phillips-Bute B, et al. Longitudinal assessment of neurocognitive function after coronary-artery bypass surgery. N Engl J Med. 2001;344:395-402.
4 Stevens RD, Bhardwaj A. Approach to the comatose patient. Crit Care Med. 2006;34:31-41.
5 Teasdale G, Jennett B. Assessment of coma and impaired consciousness: a practical scale. Lancet. 1974;2:81-84.
6 Young GB, Ropper AH, Bolton CF. Coma and Impaired Consciousness: A Clinical. In: Perspective. New York: McGraw-Hill; 1998.
7 McKhann GM, Grega MA, Borowicz LMJr, et al. Stroke and encephalopathy after cardiac surgery: an update. Stroke. 2006;37:562-571.
8 Ely EW, Inouye SK, Bernard GR, et al. Delirium in mechanically ventilated patients: validity and reliability of the confusion assessment method for the intensive care unit (CAM-ICU). JAMA. 2001;286:2703-2710.
9 Bastos PG, Sun X, Wagner DP, et al. Glasgow Coma Scale score in the evaluation of outcome in the intensive care unit: findings from the Acute Physiology and Chronic Health Evaluation III study. Crit Care Med. 1993;21:1459-1465.
10 Booth CM, Boone RH, Tomlinson G, et al. Is this patient dead, vegetative, or severely neurologically impaired? Assessing outcome for comatose survivors of cardiac arrest. JAMA. 2004;291:870-879.
11 Plum F, Posner JB. The Diagnosis of Stupor and Coma. Oxford, U.K.: Oxford University Press, 1982.
12 Likosky DS, Marrin CA, Caplan LR, et al. Determination of etiologic mechanisms of strokes secondary to coronary artery bypass graft surgery. Stroke. 2003;34:2830-2834.
13 Llinas R, Barbut D, Caplan LR. Neurologic complications of cardiac surgery. Prog Cardiovasc Dis. 2000;43:101-112.
14 Barbut D, Lo YW, Hartman GS, et al. Aortic atheroma is related to outcome but not numbers of emboli during coronary bypass. Ann Thorac Surg. 1997;64:454-459.
15 Goto T, Baba T, Matsuyama K, et al. Aortic atherosclerosis and postoperative neurologic dysfunction in elderly coronary surgical patients. Ann Thorac Surg. 2003;75:1912-1918.
16 Djaiani G, Fedorko L, Borger M, et al. Mild to moderate atheromatous disease of the thoracic aorta and new ischemic brain lesions after conventional coronary artery bypass graft surgery. Stroke. 2004;35:e356-e358.
17 Ohteki H, Itoh T, Natsuaki M, et al. Intraoperative ultrasonic imaging of the ascending aorta in ischemic heart disease. Ann Thorac Surg. 1990;50:539-542.
18 Katz ES, Tunick PA, Rusinek H, et al. Protruding aortic atheromas predict stroke in elderly patients undergoing cardiopulmonary bypass: experience with intraoperative transesophageal echocardiography. J Am Coll Cardiol. 1992;20:70-77.
19 Konstadt SN, Reich DL, Kahn R, et al. Transesophageal echocardiography can be used to screen for ascending aortic atherosclerosis. Anesth Analg. 1995;81:225-228.
20 Bergman P, van der Linden J, Forsberg K, et al. Preoperative computed tomography or intraoperative epiaortic ultrasound for the diagnosis of atherosclerosis of the ascending aorta ? Heart Surg Forum. 2004;7:E245-E249.
21 Schachner T, Nagele G, Kacani A, et al. Factors associated with presence of ascending aortic atherosclerosis in CABG patients. Ann Thorac Surg. 2004;78:2028-2032.
22 Gold JP, Torres KE, Maldarelli W, et al. Improving outcomes in coronary surgery: the impact of echo-directed aortic cannulation and perioperative hemodynamic management in 500 patients. Ann Thorac Surg. 2004;78:1579-1585.
23 Hogue CWJr, Palin CA, Arrowsmith JE. Cardiopulmonary bypass management and neurologic outcomes: an evidence-based appraisal of current practices. Anesth Analges. 2006;103:21-37.
24 Goto T, Baba T, Honma K, et al. Magnetic resonance imaging findings and postoperative neurologic dysfunction in elderly patients undergoing coronary artery bypass grafting. Ann Thorac Surg. 2001;72:137-142.
25 Floyd TF, Shah PN, Price CC, et al. Clinically silent cerebral ischemic events after cardiac surgery: their incidence, regional vascular occurrence, and procedural dependence. Ann Thorac Surg. 2006;81:2160-2166.
26 Sablotzki A, Dehne MG, Friedrich I, et al. Different expression of cytokines in survivors and non-survivors from MODS following cardiovascular surgery. Eur J Med Res. 2003;8:71-76.
27 Stolz E, Gerriets T, Kluge A, et al. Diffusion-weighted magnetic resonance imaging and neurobiochemical markers after aortic valve replacement: implications for future neuroprotective trials ? Stroke. 2004;35:888-892.
28 Shann KG, Likosky DS, Murkin JM, et al. An evidence-based review of the practice of cardiopulmonary bypass in adults: a focus on neurologic injury, glycemic control, hemodilution, and the inflammatory response. J Thorac Cardiovasc Surg. 2006;132:283-290.
29 Fiebach JB, Schellinger PD, Jansen O, et al. CT and diffusion-weighted MR imaging in randomized order: diffusion-weighted imaging results in higher accuracy and lower interrater variability in the diagnosis of hyperacute ischemic stroke. Stroke. 2002;33:2206-2210.
30 Perez-Vela JL, Ramos-Gonzalez A, Lopez-Almodovar LF, et al. Neurologic complications in the immediate postoperative period after cardiac surgery: role of brain magnetic resonance imaging. Rev Esp Cardiol. 2005;58:1014-1021.
31 Wityk RJ, Goldsborough MA, Hillis A, et al. Diffusion- and perfusion-weighted brain magnetic resonance imaging in patients with neurologic complications after cardiac surgery. Arch Neurol. 2001;58:571-576.
32 Moody DM, Brown WR, Challa VR, et al. Brain microemboli associated with cardiopulmonary bypass: a histologic and magnetic resonance imaging study. Ann Thorac Surg. 1995;59:1304-1307.
33 Brooker RF, Brown WR, Moody DM, et al. Cardiotomy suction: a major source of brain lipid emboli during cardiopulmonary bypass. Ann Thorac Surg. 1998;65:1651-1655.
34 Willcox TW, Mitchell SJ, Gorman DF. Venous air in the bypass circuit: a source of arterial line emboli exacerbated by vacuum-assisted drainage. Ann Thorac Surg. 1999;68:1285-1289.
35 Abu-Omar Y, Cifelli A, Matthews PM, et al. The role of microembolisation in cerebral injury as defined by functional magnetic resonance imaging. Eur J Cardiothorac Surg. 2004;26:586-591.
36 Wijdicks EF, Hijdra A, Young GB, et al. Practice parameter: prediction of outcome in comatose survivors after cardiopulmonary resuscitation (an evidence-based review): report of the Quality Standards Subcommittee of the American Academy of Neurology. Neurology. 2006;67:203-210.
37 Zandbergen EG, de Haan RJ, Stoutenbeek CP, et al. Systematic review of early prediction of poor outcome in anoxic-ischaemic coma. Lancet. 1998;352:1808-1812.
38 Hoofien D, Gilboa A, Vakil E, et al. Traumatic brain injury (TBI) 10-20 years later: a comprehensive outcome study of psychiatric symptomatology, cognitive abilities and psychosocial functioning. Brain Inj. 2001;15:189-209.
39 Lederer W, Lichtenberger C, Pechlaner C, et al. Long-term survival and neurologic outcome of patients who received recombinant tissue plasminogen activator during out-of-hospital cardiac arrest. Resuscitation. 2004;61:123-129.
40 Satz P, Zaucha K, Forney DL, et al. Neuropsychological, psychosocial and vocational correlates of the Glasgow Outcome Scale at 6 months post-injury: a study of moderate to severe traumatic brain injury patients. Brain Inj. 1998;12:555-567.
41 Hanger HC, Fogarty B, Wilkinson TJ, et al. Stroke patients ’ views on stroke outcomes: death versus disability. Clin Rehabil. 2000;14:417-424.
42 Hauswald M, Tanberg D. Out-of-hospital resuscitation preferences of emergency health care workers. Am J Emerg Med. 1993;11:221-224.
43 Hypothermia after cardiac arrest study group. Mild therapeutic hypothermia to improve the neurologic outcome after cardiac arrest. N Engl J Med. 2002;346:549-556.
44 Bernard SA, Gray TW, Buist MD, et al. Treatment of comatose survivors of out-of-hospital cardiac arrest with induced hypothermia. N Engl J Med. 2002;346:557-563.
45 Kronzon I, Tunick PA. Aortic atherosclerotic disease and stroke. Circulation. 2006;114:63-75.
46 van Dijk D, Keizer AM, Diephuis JC, et al. Neurocognitive dysfunction after coronary artery bypass surgery: a systematic review. J Thorac Cardiovasc Surg. 2000;120:632-639.
47 Mullges W, Berg D, Schmidtke A, et al. Early natural course of transient encephalopathy after coronary artery bypass grafting. Crit Care Med. 2000;28:1808-1811.
48 Selnes OA, Grega MA, Borowicz LMJr, et al. Cognitive changes with coronary artery disease: a prospective study of coronary artery bypass graft patients and nonsurgical controls. Ann Thorac Surg. 2003;75:1377-1384. discussion 1384-1386
49 Mahanna EP, Blumenthal JA, White WD, et al. Defining neuropsychological dysfunction after coronary artery bypass grafting. Ann Thorac Surg. 1996;61:1342-1347.
50 Ho PM, Arciniegas DB, Grigsby J, et al. Predictors of cognitive decline following coronary artery bypass graft surgery. Ann Thorac Surg. 2004;77:597-603.
51 Arrowsmith JE, Grocott HP, Newman MF. Neurologic risk assessment, monitoring and outcome in cardiac surgery. J Cardiothorac Vasc Anesth. 1999;13:736-743.
52 Herrmann M, Ebert AD, Tober D, et al. A contrastive analysis of release patterns of biochemical markers of brain damage after coronary artery bypass grafting and valve replacement and their association with the neurobehavioral outcome after cardiac surgery. Eur J Cardiothorac Surg. 1999;16:513-518.
53 Vermeer SE, Prins ND, den Heijer T, et al. Silent brain infarcts and the risk of dementia and cognitive decline. N Engl J Med. 2003;348:1215-1222.
54 Selnes OA, McKhann GM. Neurocognitive complications after coronary artery bypass surgery. Ann Neurol. 2005;57:615-621.
55 Schmitz C, Weinreich S, White J, et al. Can particulate extraction from the ascending aorta reduce neurologic injury in cardiac surgery ? J Thorac Cardiovasc Surg. 2003;126:1829-1838.
56 Grigore AM, Grocott HP, Mathew JP, et al. The rewarming rate and increased peak temperature alter neurocognitive outcome after cardiac surgery. Anesth Analg. 2002;94:4-10.
57 van Dijk D, Jansen EW, Hijman R, et al. Cognitive outcome after off-pump and on-pump coronary artery bypass graft surgery: a randomized trial. JAMA. 2002;287:1405-1412.
58 Dijkstra JB, Houx PJ, Jolles J. Cognition after major surgery in the elderly: test performance and complaints. Br J Anaesth. 1999;82:867-874.
59 Hopkins RO, Weaver LK, Collingridge D, et al. Two-year cognitive, emotional, and quality-of-life outcomes in acute respiratory distress syndrome. Am J Respir Crit Care Med. 2005;171:340-347.
60 Rosengart TK, Sweet J, Finnin EB, et al. Neurocognitive functioning in patients undergoing coronary artery bypass graft surgery or percutaneous coronary intervention: evidence of impairment before intervention compared with normal controls. Ann Thorac Surg. 2005;80:1327-1334. discussion 1334-1335
61 Sharma AD, Parmley CL, Sreeram G, et al. Peripheral nerve injuries during cardiac surgery: risk factors, diagnosis, prognosis, and prevention. Anesth Analg. 2000;91:1358-1369.
62 Lederman RJ, Breuer AC, Hanson MR, et al. Peripheral nervous system complications of coronary artery bypass graft surgery. Ann Neurol. 1982;12:297-301.
63 Seyfer AE, Grammer NY, Bogumill GP, et al. Upper extremity neuropathies after cardiac surgery. J Hand Surg. 1985;10:16-19.
64 Wilcox P, Baile EM, Hards J, et al. Phrenic nerve function and its relationship to atelectasis after coronary artery bypass surgery. Chest. 1988;93:693-698.
65 Markand ON, Moorthy SS, Mahomed Y, et al. Postoperative phrenic nerve palsy in patients with open-heart surgery. Ann Thorac Surg. 1985;39:68-73.
66 Hamdan AL, Moukarbel RV, Farhat F, et al. Vocal cord paralysis after open-heart surgery. Eur J Cardiothorac Surg. 2002;21:671-674.
67 Latronico N, Shehu I, Seghelini E. Neuromuscular sequelae of critical illness. Curr Opin Crit Care. 2005;11:381-390.
68 Maramattom BV, Wijdicks EF. Acute neuromuscular weakness in the intensive care unit. Crit Care Med. 2006;34:2835-2841.