Neurologic Control of Ventilation
I The following areas located throughout the body each play a specific role in the neurologic control of ventilation. Two general regulatory mechanisms exist: automatic or involuntary control and voluntary or conscious control.
A Located within the medulla oblongata is the respiratory control center, which receives afferent impulses from all other areas in the body (Figure 6-1).
B Afferent impulses are interpreted, and efferent impulses are initiated in the medulla oblongata.
C The medullary respiratory center maintains the normal rhythmic pattern of ventilation.
D The medullary respiratory center is located in the brain stem along with the pons and connects the midbrain and cerebellum with the spinal cord.
E Two fairly distinct areas in the medulla contain respiratory neurons (Figure 6-2).
1. The dorsal respiratory group is located in two elongated bundles of neurons along the lateral walls of the medulla referred to as the nucleus tractus solitarius (NTS).
a. Functions as initial processing centers of afferent impulses.
b. Origin of inspiratory efferent impulses, which travel to ventral respiratory group neurons and spinal cord.
c. The basic rhythm of respiration is generated by the dorsal respiratory group of neurons. Rhythmic ventilatory impulses are generated even when all peripheral nerves entering the medulla have been severed.
d. Inspiration is normally (except during stressed breathing) a ramp signal, increasing steadily in force for approximately 2 seconds.
e. Inspiration then ceases for approximately 3 seconds.
f. The ramp signal is controlled in two ways:
g. If the ramp signal ceases early the length of expiration is also decreased.
h. As a result, the dorsal respiratory group is the primary controller of the depth and rate of inspiration.
2. The ventral respiratory group is located approximately 5 mm anterior and lateral to each dorsal respiratory group. These neurons are located in the nucleus ambiguus anteriorly and the nucleus retroambiguus caudally.
F Areas from which afferent impulses are sent to the medulla oblongata:
A Two distinct centers in the pons contain afferent respiratory neurons.
1. The pneumotaxic center is located dorsally in the nucleus parabrachialis of the upper pons (see Figure 6-2).
a. Afferent impulses from the pneumotaxic center “fine tune” ventilatory rhythmicity by inhibiting length of inspiration.
b. Maximum stimulation in adults can limit inspiration to 0.5 seconds, increasing rate to ≥40 breaths/min.
c. A weak signal reduces respiratory rate to only 3 to 5 breaths/min.
d. If the pneumotaxic center is destroyed, apneustic breathing (long, sustained inspirations) occurs.
2. Apneustic center: Only weak evidence of its existence is available (see Figure 6-2).
b. Afferent impulses from the apneustic center cause a sustained inspiratory pattern with only short expiratory times; apneustic breathing.
c. How and when these neurons are activated is unclear; the vagus nerve must be impaired for this center to be active.
d. If the apneustic and pneumotaxic centers are destroyed, a rapid, irregular, gasping respiratory pattern develops.
A Axons from the higher brain centers descend into the spinal cord.
B These projecting axons influence phrenic intercostal and abdominal motoneuron transmission.
C Thus ventilatory skeletal muscle is stimulated.
D Skeletal muscle is composed of two types of contractile fibers:
1. Extrafusal fibers (main muscle): Contraction of these fibers is responsible for actual muscular contraction.
2. Fusimotor fibers (muscle spindle fibers): These fibers are organs of proprioception that determine the extent of muscle contraction necessary to perform a certain workload.
E Ventilatory reflexes, such as cough and hiccup, are mediated by the spinal cord.
F Ascending spinal pathways transmit sensations of pain, touch, temperature, and proprioception to higher brain centers.
1. Stimulation of nasal mucosa may cause exhalation.
2. Stimulated exhalation is frequently in the form of a sneeze.
3. Apnea and bradycardia also may result from nasal stimulation.
1. Stimulation may cause the sniff or aspiration reflex.
2. A rapid inspiration is initiated to move the irritant from the nasopharynx to the oropharynx.
3. Stimulation may also cause bronchodilation and hypertension.
A Afferent impulses via the vagus nerve originate from two areas:
a. Located in the aortic arch.
b. Stimulated by variation in blood pressure.
c. Afferent impulses from baroreceptors cause alteration of vascular tone to maintain normal blood pressure levels.
d. Ventilatory response is minimal.
a. Pulmonary stretch receptors (Hering-Breuer reflex)
(1) Pulmonary stretch receptors are located in the smooth muscle of conducting airways.
(2) Lung inflation and increased transpulmonary pressures stimulate these receptors.
(3) They are slowly adaptive to changes in inflating pressure but do not appear to be active in humans until tidal volume exceeds 1.5 L.
(4) Stimulation of these receptors may cause:
(5) Integration of information from these receptors assists in determining the rate and depth of breathing.
(1) Lung collapse stimulates an increased force and frequency of inspiratory effort.
(2) Mediated by the vagus nerve, but the specific receptors involved are unknown.
(1) Irritant receptors are located in the epithelium of the trachea, bronchi, larynx, nose, and pharynx.
A Innervates the peripheral chemoreceptor cells located in the carotid bodies.
B Conducts afferent impulses to the medullary respiratory center.
VIII Peripheral Chemoreceptors (Figure 6-3)
A Chemoreceptor cells can differentiate between concentrations or pressures of various substances.
B Two primary groups of peripheral chemoreceptor cells have been identified.
a. Located at the bifurcation of the common carotid artery.
3. Other less well-differentiated groups of cells are located in association with arteries in the thoracic and abdominal regions of the body.
C In general, a synergistic response from these receptors is noted in the presence of hypoxemia and acidosis.
1. Initial stimulation occurs at a Pao2 of 500 mm Hg and gradually increases as Pao2 decreases.
2. Maximum stimulation occurs when Pao2 is between 40 and 60 mm Hg.
3. A gradual decrease in stimulation is noted when Pao2 is <30 mm Hg.
4. Additional sources of stimulation:
5. These cells have a high blood flow for the size of tissue involved.
6. They are primarily affected by oxygen delivery in the form of dissolved oxygen. Any pathophysiologic situation in which oxygen delivery is inadequate for the metabolic needs of these cells results in stimulation.
E Effects of Paco2 and H++ concentrations
1. The cell membrane is permeable to H+ and Paco2.
2. These cells are directly affected only by H+ concentrations.
3. Paco2 changes cause a change in H+ concentration, which stimulates the receptor.
4. Thus, Paco2 has an indirect effect on these cells.
5. Stimulation is primarily a result of an increase in H+ concentration.
6. Decreases in H+ concentration have only a minimal effect.
7. Stimulation of these receptors by an increase in [H+] causes:
8. Stimulation of these receptors by a decrease in [H+] may cause:
9. The magnitude of the response of the peripheral chemoreceptors to [H+] changes is less than that of the central chemoreceptors.
IX Central Chemoreceptors (Figure 6-4)
A Poorly defined groups of cells located near the ventrolateral surface of each side of the medulla oblongata.
B These cells are in contact with cerebral spinal fluid (CSF) and arterial blood.
C Actual stimulation is caused by [H+] of CSF.
D The composition of the CSF differs somewhat from that of blood.
1. Electrolytes are similar in content to those in plasma.
E Diffusion across blood-brain barrier
1. The only readily diffusible substanc across the blood-brain barrier is carbon dioxide.
2. HCO3− and H+ also move across the membrane but extremely slowly. Active transport mechanisms and diffusion are believed to be involved in the movement of these two substances.
1. Changes in arterial Pco2 alter diffusion of carbon dioxide across the blood-brain barrier, causing a change in Pco2 of the CSF.
2. The altered Pco2 level effects a change in CSF [H+].
3. The altered [H+]either stimulates or inhibits ventilation.
4. Increased Pco2 (increased H+) stimulates ventilation, whereas decreased Pco2 (decreased H+) inhibits ventilation.
X Medullary Adjustments in Compensated Respiratory Acidosis
A Acute increases in Paco2 rapidly cause an increase in CSF Pco2. This occurs because the blood-CSF barrier is permeable to CO2.
B The increased Pco2 in the CSF causes the CSF pH to decrease, which stimulates the central chemoreceptors.
C If the body is unable to increase its level of ventilation, the elevated Paco2 and CSF Pco2 levels persist.
D As a result, the kidney begins to retain HCO3−.
E As the serum HCO3− level increases, active transport mechanisms and diffusion increase the CSF HCO3− level.
F The CSF pH eventually returns to normal as the CSF HCO3− level increases.
G When the CSF pH is returned to normal, the body responds to changes in Paco2 at the newly elevated level.
XI Medullary Adjustments in Compensated Respiratory Alkalosis
A Acute decreases in Paco2 rapidly cause a decrease in CSF Pco2.
B This causes the CSF pH to increase, which inhibits the central chemoreceptors.
C If the stimulus causing hyperventilation persists, the decreased Paco2 and CSF Pco2 levels also persist.
D As a result, the kidney will begin to excrete more HCO3−.
E As the serum HCO3− level decreases, active transport mechanisms and diffusion decrease the CSF HCO3− level.
F The CSF pH eventually returns to normal as the CSF HCO3− decreases.
G When the CSF pH is returned to normal, the body responds to changes in Paco2 at the new decreased level.
XII Medullary Adjustments in Compensated Metabolic Acidosis
A Because H+ does not readily cross the blood-brain barrier, decreases in plasma pH stimulate the peripheral chemoreceptors.
B This is interpreted as an increase in Paco2; thus, the peripheral chemoreceptors increase the level of ventilation, decreasing Paco2.
C The decreased Paco2 decreases the CSF Pco2, which increases the CSF pH, resulting in inhibition to ventilation via the central chemoreceptors.
D As a result, the peripheral chemoreceptors stimulate ventilation, whereas the central chemoreceptors inhibit ventilation.
E Because the effect on the peripheral chemoreceptors is the predominant stimulus, there is a stepwise readjustment (decrease) in CSF HCO3− levels. This allows normalization of CSF pH and a sustained increase in the drive to ventilate.
F The maximum response of the respiratory system to a metabolic acidosis does not occur until the CSF pH is normalized.
XIII Medullary Adjustments in Compensated Metabolic Alkalosis
A Because neither H+ nor HCO3− readily cross the blood-brain barrier and the peripheral chemoreceptors respond poorly to alkalosis, the respiratory system’s response to a metabolic alkalosis is poor unless the alkalosis is significant.
B A significant increase in plasma pH causes inhibition of the peripheral chemoreceptors, which inhibits ventilation, resulting in increased Paco2.
C The increased Paco2 increases the CSF Pco2, which stimulates ventilation via the central chemoreceptors.
D As a result, the peripheral chemoreceptors inhibit ventilation, whereas the central chemoreceptors stimulate ventilation.
E Because inhibition of the peripheral chemoreceptors is the predominant stimulus, there is a stepwise readjustment (increase) in the CSF HCO3−. This allows a normalization of the CSF pH and a sustained decrease in the drive to ventilate.
F However, it is rare that the Paco2 will increase above 50 mm Hg in an attempt to compensate unless the metabolic alkalosis is severe. Remember, if the Paco2 increases, the alveolar Po2 decreases, resulting in hypoxemia, which stimulates ventilation via the peripheral chemoreceptors.
XIV Voluntary Control of Ventilation
A Most voluntary control of ventilation is initiated via the cerebral cortex.
B The thalamus is involved in controlling breathing during emotional behavior:
A The drive to breathe is affected by the numerous factors presented earlier. However, many individuals have varying responses to individual stimuli.
XVI Abnormal Breathing Patterns (Figures 6-5 and 6-6)
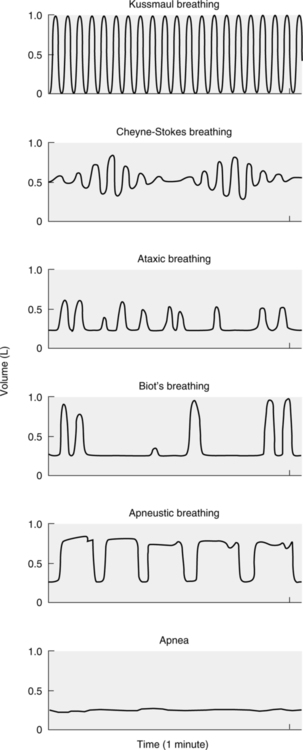
A Normal breathing in adults at a rate of 10 to 20 breaths/min is referred to as eupnea.
B Rates >20 breaths/min are referred to as tachypnea.
C Rates <10 breaths/min are termed bradypnea.
D Tidal volumes greater than normal for an individual are termed hyperpnea.
E Tidal volumes smaller than normal for an individual are termed hypopnea.
F Kussmaul breathing is the rapid and deep breathing associated with a severe diabetic acidosis.
G Cheyne-Stokes breathing is a pattern of breathing associated with increasing and then decreasing tidal volumes, followed by a period of apnea (the absence of breathing). This pattern is associated with brain injury or severe cardiovascular disease.
H Ataxic breathing is a pattern of breathing with irregular tidal volumes and rates seen during respiratory muscle fatigue and impending respiratory failure.
I Biot’s breathing is a highly irregular breathing pattern associated with periods of apnea; seen in individuals with severe brain stem injury unable to maintain control of breathing.
J Apneustic breathing is a pattern defined by long sustained inspirations and short expiratory times; seen when injury to the pons is present.