Chapter 35 Neuro-ophthalmology
Ocular Motor System
The human fovea is a highly sensitive part of the retina capable of resolving angles of less than 20 arc seconds. The ocular motor system places images of objects of regard on the fovea and maintains fixation (foveation) if the object or head moves. Each eye has six extraocular muscles (Table 35.1) yoked in pairs (Table 35.2) that move the eyes conjugately (versions) to maintain alignment of the visual axes (Fig. 35.1). The actions of the medial and lateral recti are essentially confined to the horizontal plane. The actions of the superior and inferior recti are solely vertical when the eye is abducted 23 degrees. The oblique muscles, the main cyclotortors, also act as pure vertical movers when the eye is adducted 51 degrees (Fig. 35.2). For practical purposes, the vertical actions may be tested at 30 degrees of adduction and abduction. According to the Hering law of dual innervation, yoked muscles receive equal and simultaneous innervation while their antagonists are inhibited (the Sherrington law of reciprocal inhibition), thereby allowing the eyes to move conjugately and with great precision. The pulling actions of the extraocular muscles evolved to move the eyes in the planes of the semicircular canals, which are not strictly horizontal or vertical. These pulling actions are influenced by both the conventional insertions of the global layer of each extraocular muscle directly into the eyeball and by the insertion of the orbital layer into the fibromuscular connective tissue sheath that envelopes each rectus muscle (Fig. 35.3). This arrangement forms a pulley system that is actively innervated (Demer, 2002), stabilizes rotation of the globes in three-dimensional space during complex eye movements (e.g., when a horizontal muscle contracts during upgaze), and prevents excessive retraction of the globe within the orbit during extraocular muscle contraction.
Ipsilateral | Contralateral |
---|---|
Medial rectus | Lateral rectus |
Superior rectus | Inferior oblique |
Inferior rectus | Superior oblique |
Images of the same object must fall on corresponding points of each retina to maintain binocular single vision (fusion) and stereopsis (Fig. 35.4). If the visual axes are not aligned, the object is seen by noncorresponding (disparate) points of each retina, and diplopia results (Fig. 35.5). In patients with paralytic strabismus, the image from the nonfixating paretic eye is the false image and is displaced in the direction of action of the weak muscle. Thus, a patient with esotropia has uncrossed diplopia (see Fig. 35.5, A), and a patient with exotropia has crossed diplopia (see Fig. 35.5, B). After a variable period, the patient person learns to ignore or suppress the false image. If suppression occurs before visual maturity (approximately 6 years of age) and persists, central connections in the afferent visual system fail to develop fully, leading to permanent visual impairment in that eye (developmental amblyopia). Amblyopia is more likely to develop with esotropia than with exotropia, because exotropia is commonly intermittent. After visual maturity, suppression and amblyopia do not occur; instead, the patient learns to avoid diplopia by ignoring the false image.
Heterophorias and Heterotropias
Divergent eyes are designated exotropic and convergent eyes are esotropic. Vertical misalignment of the visual axes is less common: When the nonfixating eye is higher, the patient is said to have a hypertropia, and when it is lower, a hypotonia (Donahue, 2007), irrespective of which eye is abnormal; for example, with a right hypertropia, the right eye is higher. Asymptomatic hypertropia on lateral gaze often is a congenital condition or “physiological hyperdeviation.”
Comitant Strabismus
New-onset strabismus at school age (after age 6 years) is unusual and warrants evaluation for a neurological disorder. Comitant strabismus occurs early in life; the magnitude of misalignment (deviation) is similar in all directions of gaze, and each eye has a full range of movement (i.e., full ductions). Probably, it occurs because of failure of central mechanisms in the brain that keep the eyes aligned. Infantile (congenital) esotropia may be associated with maldevelopment of the afferent visual system, including the visual cortex, and presents within the first 6 months of life; those with comitant esotropia of more than 40 prism diopters (20 degrees) do not “grow out of it” and require surgical correction (Donahue, 2007). Evidence using cortical motion visual evoked potentials indicates that early correction of strabismus (before 11 months of age) improves visual cortical development (Gerth et al., 2008). Cases of comitant esotropia that manifest between the ages of 7 months and 7 years (average years) are caused by hyperopia (farsightedness) resulting in accommodative esotropia: children with excessive farsightedness must accommodate to have clear vision; the constant accommodation causes excessive convergence and leads to persistent esotropia. Accommodative esotropia responds well to spectacle correction alone. Evidence indicates that high-level stereopsis is restored in these children (unlike those with uncorrected infantile esotropia) if treatment is initiated within 3 months of the onset of constant esotropia (Fawcett et al., 2005).
Occasionally, children with Chiari malformations or posterior fossa tumors present with isolated esotropia before the appearance of other symptoms or signs. Features that suggest a structural cause for the esotropia include presentation after age 6, complaints such as diplopia or headache, incomitance in horizontal gaze, esotropia greater at distance than near, and neurological findings such as abduction deficits, ataxia, optic disc edema, pathological nystagmus, and saccadic pursuit. Adults in whom isolated esotropia develops, particularly when they become presbyopic in their early 40s, should have a cycloplegic refraction to detect latent hyperopia. Other causes of adult-onset esotropia include Chiari malformations and acute thalamic hemorrhage (Box 35.1).
Box 35.1 Causes of Esotropia
Congenital esotropia (also acquired, cyclic)
Abducens palsy (unilateral or bilateral)
Tonic convergence spasm (part of dorsal midbrain syndrome)
Pseudo–sixth cranial nerve palsy of Fisher
Posterior internuclear ophthalmoplegia of Lutz (pseudo-sixth)
Cyclical oculomotor palsy (spastic phase)
Nystagmus blockage syndrome (in congenital and latent nystagmus)
Abducens palsy with contracture of antagonist (ipsilateral medial rectus) during recovery
Medial rectus entrapment (blowout fracture)
Thyroid myopathy (rare at presentation)
Orbital disorders (orbital varix, infiltrative lesions)
Stiff person syndrome (associated with abduction deficits, hypometric saccades) (Economides and Horton, 2005)
Noncomitant (Incomitant) Strabismus
Noncomitant strabismus occurs when the degree of misalignment of the visual axes varies with the direction of gaze as a result of weakness or restriction of one or more extraocular muscles. When a patient with a noncomitant strabismus fixates on an object with the nonparetic eye, the angle of misalignment is referred to as the primary deviation. When the patient fixates with the paretic eye, the angle of misalignment is referred to as the secondary deviation. Secondary deviation is always greater than primary deviation in noncomitant strabismus because of the Hering law of dual innervation; it may mislead the examiner to believe that the eye with the greater deviation is the weak one (Fig. 35.6).
Diplopia
Theoretically, the onset of double vision should be abrupt. However, in practice the history of onset may be vague for various reasons. The patient may interpret subtle diplopia as blurring unless one eye is covered, or the onset may be uncertain because the diplopia is intermittent initially, of small amplitude, or compensated for by head position, as may be the case in disorders such as congenital superior oblique palsy, ocular myasthenia, and thyroid eye disease. Guidelines for evaluation for diplopia are presented in Box 35.2.
Box 35.2 Rules for Evaluation for Diplopia
1. Head tilt: When the weak extraocular muscle is unable to move the eye, the head moves the eye. Therefore, the head tilts or turns (or both) in the direction of action of the weak muscle (see Fig. 35.9).
2. Image from the nonfixating eye is the false image and is displaced in the direction opposite the deviation; when the patient fixates with the nonparetic eye, the false image is displaced in the direction of action of the paretic muscle (see Fig. 35.5).
3. False image is the most peripheral image and is displaced in the direction of action of the weak muscle, except when the patient fixes with the paretic eye. When the lateral rectus is paralyzed, the eyes are esotropic (crossed), but the images are uncrossed (see Fig. 35.5A). Diplopia is worse at a distance and on looking to the side of the weak muscle. When the medial rectus is paralyzed, the eyes are exotropic (wall-eyed), but the images are crossed (see Fig. 35.5B). Diplopia is worse at near and on looking to the opposite side.
4. Images are most widely separated when an attempt is made to look in the direction of the paretic muscle.
5. Secondary deviation (angle of ocular misalignment when paretic eye is fixating) is always greater than primary deviation (when normal eye is fixating; see Fig. 35.6). Patients who fixate with the paretic eye may appear to have intracranial disease.
6. Comitance: With a comitant strabismus, the angle of ocular misalignment is relatively constant in all directions of gaze. With a noncomitant (paralytic) strabismus, the angle of misalignment varies with the direction of gaze.
Most adult patients with acquired heterotropia complain of frank double vision, but if the images are close together, the patient may not be aware of frank diplopia but merely perceive blurring, overlapping images (ghosting), or strain. Occasionally, visual confusion occurs because each fovea fixates a different object simultaneously, causing the perception of two objects in the same place at the same time (Fig. 35.7).
Anxious or histrionic patients may misinterpret physiological diplopia, a normal phenomenon, as a pathological symptom. Physiological diplopia occurs when a subject fixates an object in the foreground and then becomes aware of another object farther away but in the direction of gaze. The nonfixated object is seen by noncorresponding parts of each retina and is perceived by the mind’s (cyclopean) eye as double (Fig. 35.8). Conversely, when the subject fixates a distant object, a near object may appear double.
Isolated vertical diplopia (Box 35.3) most commonly is caused by superior oblique muscle palsy. If the palsy is acquired, one image is virtually always tilted—an infrequent finding when the palsy is congenital. If recently acquired diplopia is worse in downgaze, the weak muscle is a depressor. If the diplopia is worse in upgaze, it is an elevator. If one image is tilted, the weak muscle is more likely an oblique rather than a vertically acting rectus.
Box 35.3 Causes of Vertical Diplopia
Less Common Causes
Orbital inflammation (myositis, idiopathic orbital inflammatory syndrome [previously designated “pseudotumor”])
Orbital infiltration (lymphoma, metastases, amyloid)
Entrapment of the inferior rectus (blowout fracture)
Superior division third nerve palsy
Atypical third nerve (partial nuclear lesion)
Aberrant third nerve reinnervation
Brown syndrome (congenital, acquired)
Congenital extraocular muscle fibrosis or muscle absence
Double elevator palsy (monocular elevator deficiency); controversial in origin
Other Causes
If double vision persists when one eye is covered, the patient has monocular diplopia, which may be bilateral. The most common cause of monocular diplopia is an optical aberration (refractive error) and warrants appropriate correction (Box 35.4). Less commonly, monocular diplopia is psychogenic, but occasionally it can be attributed to dysfunction of the retina or cerebral cortex. The pinhole test quickly settles the matter. The patient is asked to look through a pinhole; if the cause is refractive, the diplopia abates because optical distortion is eliminated as the light rays entering the eye through the pinhole are aligned along the visual axis and thus not deflected. Oscillopsia (see later discussion) may be misinterpreted as diplopia.
Box 35.4 Causes of Monocular Diplopia
After surgery for long-standing tropia (eccentric fixation)
Corneal disease (e.g., astigmatism, dry eye, keratoconus)
Corrected long-standing tropia (eccentric fixation)
Equipment failure (defective contact lens, ill-fitting bifocals in patients with dementia)
Foreign body in aqueous or vitreous media
Iris abnormalities (polycoria, trauma)
Lens: multirefractile (combined cortical and nuclear) cataracts, subluxation
Monocular oscillopsia (nystagmus, superior oblique myokymia, eyelid twitching)
Occipital cortex: migraine, epilepsy, stroke, tumor, trauma (palinopsia, polyopia)
Occasionally, disorders that displace the fovea, such as a subretinal neovascular membrane, can cause binocular diplopia by disrupting the alignment of the photoreceptors (foveal displacement syndrome). The diplopia probably results from rivalry between central and peripheral fusional mechanisms (Brazis and Lee, 1998). Central disruption of fusion (see later) and horror fusionis (in patients with asymmetrical retinal disease) causes intractible diplopia.
Clinical Assessment
History
Box 35.5 shows the procedure for assessing patients with diplopia. The following points should be clarified if the patient has not volunteered the information: Is the diplopia relieved by covering either eye? (If not, it is monocular diplopia; see Box 35.4.) Is it worse in the morning or in the evening? Is it affected by fatigue? Are the images separated horizontally, vertically, or obliquely? If obliquely, is the horizontal or vertical component more obvious? Is the distance between images constant despite the direction of gaze, or does it vary? Is the diplopia worse for near vision or for distance? Is one image tilted? Do the eyelids droop? Is the diplopia influenced by head posture? Has this condition remained stable, improved, or deteriorated? Are there any general health problems? Are there associated symptoms such as headache, dizziness, vertigo, or weakness? What medications are taken? Is there a family history of ocular, neurological, autoimmune, or endocrine disease? Has the patient had a “lazy” eye, worn a patch, or had strabismus surgery?
Box 35.5 Assessment of the Patient with Diplopia
Eye Examination
Visual acuity (each eye separately, and binocularly if primary position nystagmus present)
Versions (pursuit, saccades, and muscle overaction)
Convergence (does miosis occur?)
Ocular alignment (muscle balance) in the “forced primary position”
Lids (examine palpebral fissures, levator function, fatigue)
Vestibulo-ocular reflexes (doll’s eye reflex)
Head Posture
Because the weak extraocular muscle cannot move the eye fully, patients compensate by tilting or turning the head in the direction of action of the weak muscle (Fig. 35.9). For example, with right lateral rectus palsy, the head is slightly turned to the right; then on attempted right gaze, the patient turns the head farther to the right (see Fig. 35.9, A). With a right superior oblique palsy, the head tilts forward and to the left (see Fig. 35.9, B). The rule is as follows: The head turns or tilts in the direction of action of the weak muscle.
Versions (Pursuit, Saccades, and Ocular Muscle Overaction)
Pursuit movements are tested by asking the patient to fixate and follow (track) a moving target in all directions (Fig. 35.10, A). This test determines the range of eye movement and provides an opportunity to observe for gaze-evoked nystagmus. If spontaneous primary-position nystagmus is present, the effects of the direction of gaze and convergence on the nystagmus may be determined. Pursuit movements should be smooth and full. Cogwheel (saccadic) pursuit is a nonspecific finding and is normal in infants. When present in only one direction, however, it suggests a defect of the ipsilateral pursuit system.
Saccades (fast eye movements) are tested by asking the patient to look rapidly from one target to another (e.g., from the examiner’s nose to a pen) while observing for a delay in initiating the movement (latency) as well as the movement’s speed, accuracy, and conjugacy. An internuclear ophthalmoplegia is best detected by this method (Video 35.1, available at www.expertconsult.com). If a specific muscle (particularly an oblique) underacts or overacts, this can be observed in eccentric gaze before testing ductions in each eye separately, as shown in Fig. 35.10, B. Assessment of disorders of conjugate (supranuclear) gaze is discussed later.
Ductions
Ductions are tested monocularly by having the patient cover one eye and checking the range of movements of the other eye (see Fig. 35.10, B). If ductions are not full, the physician should check for restrictive limitation by moving the eye forcibly (see Forced Ductions, later).
Ocular Alignment and Muscle Balance
With the red glass test, the patient views a penlight while a red filter or glass is placed, by convention, over the right eye. This allows easier identification of each image; the right eye views a red light and the left a white light. The addition of a green filter over the left eye, using red-green glasses, further simplifies the test for younger or less reliable patients. The target light is shown to the patient in the nine diagnostic positions of gaze (see Fig. 35.10, A). As the light moves into the field of action of a paretic muscle, the images separate. The patient is asked to signify where the images are most widely separated and to describe their relative positions. Interpretation of the results is summarized in Fig. 35.11.
The Maddox rod test uses the same principle as for the red glass test, but the images are completely dissociated by changing the point of light seen through the rod, which is a series of half cylinders, to a straight line perpendicular to the cylinders (Fig. 35.12). This dissociation of images (a point of light and a line) breaks fusion, allowing detection of heterophorias as well as heterotropias. Cyclotorsion may be detected by asking if the image of the line is tilted (see Fig. 35.15, B). The Maddox rod can be positioned to produce a horizontal, vertical, or oblique line.
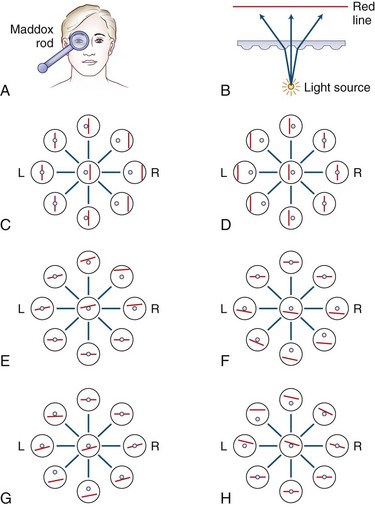
Fig. 35.12 The Maddox rod test. (Unlike in Fig. 35.11, the images are displayed as the patient perceives them.) A, By convention, the right eye is covered by the Maddox rod, which may be adjusted so the patient sees a red line, at right angles to the cylinders, in the horizontal or vertical plane, as desired (red image seen by the right eye; light source seen by the left eye). B, The Maddox rod is composed of a series of cylinders that diffract a point of light to form a line. C, Right lateral rectus palsy. D, Right medial rectus palsy. E, Right superior rectus palsy. F, Right inferior rectus palsy. G, Right superior oblique palsy. H, Right inferior oblique palsy.
The Hirschberg test, an objective method of determining ocular deviation in young or uncooperative patients, is performed by observing the point of reflection of a penlight held approximately 30 cm from the patient’s eyes (Fig. 35.13); 1 mm of decentration is equal to 7 degrees of ocular deviation. One degree is equal to approximately 2 prism diopters. One prism diopter is the power required to deviate (diffract) a ray of light by 1 cm at a distance of 1 m (Fig. 35.14).
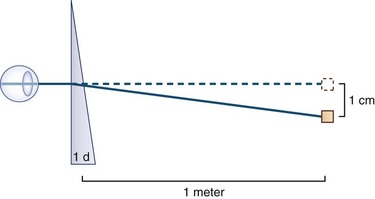
Fig. 35.14 A prism with the power of 1 prism diopter (D) can diffract a ray of light 1 cm at 1 meter.
The cover-uncover test is determined for both distance (6 m) and near (33 cm) vision. The patient is asked to fixate an object held at the appropriate distance. The left eye is covered while the patient maintains fixation on the object. If the right eye is fixating, it remains on target, but if the left eye alone is fixating, the right eye moves onto the object. If the uncovered right eye moves in (adducts), the patient has a right exotropia; if it moves out (abducts), the patient has an esotropia; if it moves down, a right hypertropia; if it moves up, a right hypotropia. The physician should always observe the uncovered eye. The test should be repeated by covering the other eye. If the patient has a tropia, the physician must determine whether it is comitant or noncomitant by checking the degree of deviation in the nine diagnostic cardinal positions of gaze (see Fig. 35.10, A). With a lateral rectus palsy, the esotropia increases on looking to the side of the weak muscle and disappears on looking to the opposite side (see Fig. 35.11, A). Similarly, with a medial rectus weakness, the patient has an exotropia that increases on looking in the direction of action of that muscle (see Fig. 35.11, B). Prisms are used mainly by ophthalmologists to measure the degree of ocular deviation (see Fig. 35.14). If the diplopia is due to breakdown of a long-standing (congenital) deviation, prism measurement can detect supranormal fusional amplitudes (large fusional reserve). If no manifest deviation of the visual axes is found using the cover-uncover test, the patient is orthotropic. Then the physician may perform the cross-cover test.
Three-Step Test for Vertical Diplopia
Eight muscles are involved in vertical eye movements: four elevators and four depressors. The three-step test endeavors to determine whether one particular paretic muscle is responsible for vertical diplopia (Fig. 35.15). Using the cover-uncover test, which is objective, or one of the subjective tests such as the red glass test, the physician can perform the three-step test for vertical diplopia. When using one of the subjective tests, it is important to remember that the hypertropic eye views the lower image.
Step 1 determines which eye is higher (hypertropic) in primary position. The patient’s head may have to be repositioned (controlled primary position) because of a compensatory tilt. If the right eye is higher, the weak muscle is either one of the two depressors of the right eye (inferior rectus or superior oblique) or one of the two elevators of the left eye (superior rectus or inferior oblique).
Step 2 determines whether the hypertropia increases on left or right gaze. If it increases on left gaze, the weak muscle is either the depressor in the right eye, which acts best in adduction (i.e., the superior oblique), or the elevator in the left eye, which acts best in abduction (i.e., the superior rectus), and vice versa.
Step 3 determines whether the hypertropia changes when the head tilts to the left or the right. If it increases on head tilt left, the weak muscle must be an intortor of the left eye (superior rectus). If it increases on head tilt right, the weak muscle must be an intortor of the right eye (superior oblique).
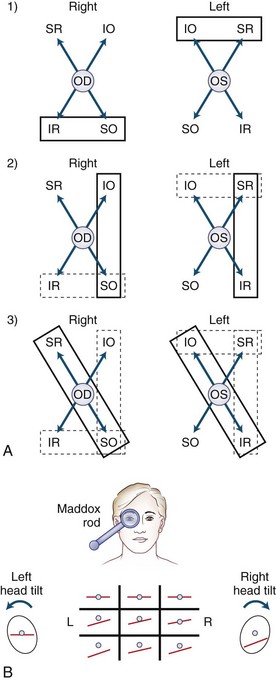
Fig. 35.15 Example of the three-step test in a patient with an acute right superior oblique palsy. A, In a patient with hypertropia, one of eight muscles may be responsible for vertical ocular deviation. Identifying the higher eye eliminates four muscles. Step 1: With a right hypertropia, the weak muscle is either one of the two depressors of the right eye (IR or SO) or one of the two elevators of the left eye (IO or SR) (enclosed by solid line). Step 2: If the deviation (or displacement of images) is greater on left gaze, one of the muscles acting in left gaze (enclosed by solid line) must be responsible, in this case either the depressor in the right eye (SO) or the elevator in the left eye (SR). Step 3: If the deviation is greater on right head tilt, the incyclotortors of the right eye (SR and SO) or the excyclotortors of the left eye (IR and IO) (enclosed) must be responsible, in this case, the right SO—that is, the muscle enclosed three times. If the deviation is greater on left head tilt, the left SR would be responsible. IO, Inferior oblique; IR, inferior rectus; SO, superior oblique; SR, superior rectus. B, The Maddox rod test (displayed as in Fig. 35.12, as the subject perceives the images) in a patient with a right SO palsy shows vertical separation of the images that is worse in the direction of action of the weak muscle and demonstrates subjective tilting of the image from the right eye. When the head is tilted toward the left shoulder, the separation disappears, but when the head is tilted to the right shoulder, to the side of the weak muscle, the separation is exacerbated (Bielschowsky’s third step).
Step 4 uses one of a number of techniques (e.g., double Maddox rod, visual field blind spots, indirect ophthalmoscopy, fundus photography) to determine whether ocular torsion is present. Establishing the degree and direction of ocular torsion, if any, can differentiate a skew deviation from a superior oblique palsy. Because the primary action of the superior oblique muscle is intorsion (see Table 35.1), an acute palsy typically results in approximately 5 degrees of extorsion of the affected eye due to unopposed action of the ipsilateral inferior oblique muscle. If either eye is intorted, a superior oblique palsy is not responsible, and the patient may have a skew deviation (Donahue et al., 1999).
Step 5 is helpful in the acute phase. If the deviation is greater on downgaze, the weak muscle is a depressor; if it is worse on upgaze, the weak muscle is likely to be an elevator. This fifth step is helpful only in the acute stage, because with time the deviation becomes more comitant.
The examiner should be aware of the pitfalls of the three-step test—namely, the conditions in which the rules break down. These include restrictive ocular myopathies (Box 35.6), long-standing strabismus, skew deviation, and disorders involving more than one muscle.
Box 35.6 Causes of Positive (Restrictive) Findings on Testing Forced Ductions
Acquired: superior oblique tendinitis, myositis, or injury
Carotid-cavernous or dural shunt fistula
Congenital: superior oblique tendon sheath syndrome
Extraocular muscle fibrosis (congenital, postoperative)
Orbital infiltration: myositis, lymphoma, metastasis, amyloidosis, cysticercosis, trichinosis
Forced Ductions
If the weak muscle does not fatigue, the physician should determine whether it is restricted by performing forced ductions (Video 35.2, available at www.expertconsult.com). The use of phenylephrine hydrochloride eye drops beforehand reduces the risk of subconjunctival hemorrhage. Although this test is in the realm of the ophthalmologist, it may be performed in the office using topical anesthesia and a cotton-tipped applicator, but great care must be taken to avoid injuring the cornea. The causes of restrictive myopathy are listed in Box 35.6; however, any cause of prolonged extraocular muscle paresis can result in contracture of its antagonist.
Signs Associated with Diplopia
When evaluating a patient with diplopia, the examiner should determine whether any of the signs outlined in Box 35.7 are present.
Box 35.7 Signs Associated with Diplopia
Extraocular muscle or lid fatigue suggests MG.
Cogan lid twitch sign suggests MG.
Weakness of other muscles (e.g., orbicularis oculi, other facial muscles, neck flexors, bulbar muscles) suggests oculopharyngeal dystrophy or MG (see Chapters 25, 78, and 79).
Narrowing of palpebral fissure and retraction of globe on adduction, associated with an abduction deficit, suggests Duane retraction syndrome (Gutowski, 2000).
Paradoxical elevation of upper lid on attempted adduction or downgaze, and pupil constriction on attempted adduction or downgaze, occurs with aberrant reinnervation of the third cranial nerve, which is virtually always a result of trauma or compression caused by tumor or aneurysm (see Chapter 70).
Miosis accompanying apparent bilateral sixth nerve palsy occurs with spasm of the near reflex.
Horner syndrome, ophthalmoplegia, and impaired sensation in the distribution of the first division of the trigeminal nerve occur with superior orbital fissure and anterior cavernous sinus lesions.
A third nerve palsy with pupillary involvement most often is due to a compressive lesion; with acute onset, a posterior communicating aneurysm usually is responsible.
Proptosis suggests an orbital lesion such as thyroid eye disease, inflammatory or infiltrative orbital disease (tumor, pseudotumor, or amyloidosis), or a carotid-cavernous sinus fistula, in which case it may be pulsatile.
Ocular bruits, often heard by both patient and doctor, occur with carotid-cavernous or dural shunt fistulas.
Entrapment (blowout fracture) is a sign of periorbital and ocular injury.
Ophthalmoplegia, ataxia, nystagmus, and confusion suggest Wernicke encephalopathy.
Pyramidal and spinothalamic signs with crossed hemiparesis suggest brainstem syndromes (Chapter 19).
Facial pain, hearing loss, and ipsilateral lateral rectus weakness indicate the Gradenigo syndrome.
INO, Internuclear ophthalmoplegia; MG, myasthenia gravis; RP, retinitis pigmentosa.
Edrophonium (Tensilon) Test
The edrophonium test is discussed in detail in Chapter 78, but a few points are emphasized here. The test must have an objective endpoint (e.g., ptosis, a tropia, limited ductions), and the physician must observe an objective change. When forced ductions are positive, indicating a restrictive myopathy, the edrophonium test will be negative and therefore is not indicated. Myasthenic ptosis may be reversed temporarily with application of an ice pack over the affected lid.
Acute Bilateral Ophthalmoplegia
The causes of acute bilateral ophthalmoplegia are outlined in Box 35.8.
Box 35.8 Causes of Acute Bilateral Ophthalmoplegia*
Basilar meningitis, hypertrophic cranial pachymeningitis, or neoplastic infiltration†
Carotid-cavernous or dural shunt fistula†
Cavernous sinus thrombosis (febrile, ill)†
HMG-CoA reductase inhibitors* (may be transient, may be associated with anti-AChR antibodies) (Negvesky et al., 2000; personal observation, 2002)
Intoxication (sedatives, tricyclics, organophosphates, anticonvulsants—consciousness impaired)
Leigh disease (subacute necrotizing encephalomyelitis)
Fisher syndrome (Miller Fisher syndrome) with or without ataxia (Lee et al., 2008)
Neuroleptic malignant syndrome (personal observation)
Paraneoplastic encephalomyelitis
Polyradiculopathy (associated with)
PERM, a variant of stiff person syndrome (Hutchinson et al., 2008)
Chronic Bilateral Ophthalmoplegia
The causes of chronic bilateral ophthalmoplegia are outlined in Box 35.9.
Box 35.9 Causes of Chronic Ophthalmoplegia
Related Disorders
Monocular elevator deficiency (double elevator palsy) is discussed later.
Triplopia, or triple vision, is rare and anatomically so unlikely that it generally is presumed to be psychogenic. However, in a review of findings in 13 patients who described triplopia, 11 had ocular motor abnormalities, including third nerve palsies in 5, sixth nerve palsies in 2, and internuclear ophthalmoplegia in 4 (Keane, 2006). Generally, the patients reported triplopia when looking in the direction of maximal nystagmus or ocular dissociation, probably as a result of oscillopsia. Psychogenic triplopia was uncommon but recognized by persistence in all directions of gaze and failure to resolve with the pinhole test.
Polyopia, the perception of multiple images, frequently is optical and can be determined by the pinhole test, discussed earlier under Diplopia. Polyopia also may be caused by cortical lesions (see Box 35.4).
Palinopsia (or palinopia), the pathological persistence or recurrence of visual images after the stimulus has been removed, may cause cerebral diplopia or polyopia. The images become more apparent and numerous when the target moves relative to the retina, because it provokes multiple persistent afterimages (Fig. 35.16). Sometimes patients describe the visual disturbance as trailing, vibrating, echoing, smearing, or ghosting of images. This visual perseveration occurs more frequently in patients with mild left homonymous visual field defects caused by right parieto-occipital lesions; it may be ictal and respond to anticonvulsant medication. Palinopsia can occur with migraine, metabolic disorders, carbon monoxide poisoning, and a variety of drugs including clomiphene, interleukin 2, lysergic acid diethylamide, mescaline, nefazodone, topiramate, trazodone, and 3,4-methylenedioxymethamphetamine (“Ecstasy”). Palinopsia can occur in patients with ocular or optic nerve disease and in apparently healthy people. Occasionally it may be associated with auditory perseveration (palinacusis) (Pomeranz and Lessell, 2000).
Nystagmus
Nystagmus, which interrupts steady fixation, is an involuntary biphasic rhythmic ocular oscillation in which one or both phases are slow (Fig. 35.17). The slow phase of jerk nystagmus is responsible for the initiation and generation of the nystagmus, whereas the fast (saccadic) phase is a corrective movement bringing the fovea back on target. Nystagmus often interferes with vision by blurring the object of regard (poor foveation), or making the environment appear to oscillate (oscillopsia), or both.
For clinical purposes, nystagmus may be divided into pendular and jerk forms. Either form may be horizontal or, less commonly, vertical. Jerk nystagmus is labeled conventionally by the direction of the fast phase and is divided into three types on the basis of the shape of the slow phase tracing on oculographic recordings (see Fig. 35.17).
Mechanisms
Nystagmus may result from dysfunction of the vestibular end-organ, vestibular nerve, brainstem, cerebellum, or cerebral centers for ocular pursuit. Pendular nystagmus (see Fig. 35.17, A) is central (brainstem or cerebellum) in origin, whereas jerk nystagmus may be either central or peripheral. Jerk nystagmus with a linear (constant velocity) slow phase (see Fig. 35.17, B) is caused by peripheral vestibular dysfunction resulting in an imbalance in vestibular input to the brainstem gaze centers. When the slow phase has a decreasing velocity exponential (see Fig. 35.17, C), the brainstem neural integrator (NI) is at fault and is said to be “leaky.” The integrator is unable to maintain a constant output to the gaze center to hold the eyes in an eccentric position, resulting in gaze-paretic nystagmus. An increasing velocity exponential slow phase (see Fig. 35.17, D) in the horizontal plane is central in origin and is the usual form of congenital nystagmus (now termed infantile nystagmus syndrome).
Clinical Evaluation
Congenital nystagmus usually is asymptomatic and rarely bothers the patient or causes oscillopsia. The physician should determine whether the nystagmus was present since birth or is acquired and whether there is a family history, a history of amblyopia or lazy eye, and what medications the patient takes. Symptoms such as headache, diplopia, impaired vision, oscillopsia, vertigo, or other neurological abnormalities must be taken into account. Examination should include assessment of visual acuity, confrontation visual fields, ocular motility, pupil reflexes, observing for ocular albinism, and ophthalmoscopy. Ophthalmoscopy may be used to detect subtle nystagmus not apparent to the naked eye. Clinical features that must be determined are listed in Box 35.10.
Box 35.10 Clinical Features to Look for in Patients with Nystagmus
Are there signs of ocular albinism?
Is there a spontaneous head tilt or turn?
Is the nystagmus present in primary position or only with eccentric gaze (gaze-evoked)?
Is the nystagmus binocular and conjugate, or is it dissociated?
Is the waveform pendular or jerk? If jerk, what is the direction of the fast phase?
Is there a latent component (i.e., an increase in nystagmus intensity when one eye is covered)?
Is there a torsional component?
Is there spontaneous alteration of direction, as with periodic alternating nystagmus? This entity must be distinguished from rebound nystagmus, for which recognition requires observation over time.
Is there a null zone (a direction of gaze in which the nystagmus is minimal or absent)?
Determine whether convergence damps the nystagmus or changes its direction.
Is the nystagmus altered (accentuated or suppressed) by head positioning or posture or by head shaking (as in spasmus nutans)?
What is the effect of optokinetic stimulation? In infantile nystagmus syndrome (INS), the response is paradoxical—that is, the fast phase is in the direction of the slow-moving target.
Are there associated rhythmic movements of other muscle groups (e.g., face, tongue, ears, neck, palate [as in oculopalatal myoclonus/tremor], limbs)?
Nystagmus Syndromes
Table 35.3 summarizes the localizing value of nystagmus syndromes and non-nystagmus ocular oscillations.
Table 35.3 Localizing Value of Nystagmus Syndromes and Non-Nystagmus Ocular Oscillations
Nystagmus Syndrome | Localization |
---|---|
Downbeat nystagmus | Bilateral cervicomedullary junction (flocculus) |
Floor of the fourth ventricle | |
PAN | Cervicomedullary junction (nodulus) |
Upbeat nystagmus | Bilateral pontomesencephalic junction |
Bilateral pontomedullary junction | |
Cerebellar vermis | |
Pendular nystagmus | Paramedian pons |
Deep cerebellar (fastigial) nuclei | |
SSN: | Mesodiencephalic junction, chiasm, disorders that disrupt central vision |
Hemi-jerk SSN | Unilateral mesodiencephalic lesions: upper poles of the eyes jerk toward side of the lesion, and vertical component is always disjunctive (eyes oscillate in opposite directions, with the intorting eye rising and the extorting eye falling) |
Lateral medullary lesions: upper poles of the eyes jerk away from the side of lesion; but the vertical component may be either conjugate, usually upward, or disjunctive | |
Alternating hemi-SSN with direction | Middle cerebellar peduncle of vertical pursuit |
Rebound nystagmus | Cerebellum |
Brun nystagmus | Cerebellopontine angle, AICA territory stroke |
Torsional nystagmus, jerk | Central vestibular system |
Torsional nystagmus, pendular | Medulla |
Atypical INS: | |
Asymmetrical horizontal | Ocular albinism |
Vertical (pendular, downbeat, or upbeat) | Retina: congenital cone dysfunction, congenital stationary night blindness |
Non-nystagmus Ocular Oscillations | Localization |
Convergence-retraction “nystagmus” | Dorsal midbrain |
Opsoclonus | Cerebellar fastigial nuclei or brainstem |
Ocular flutter | Deep cerebellum nuclei or brainstem |
Ocular dysmetria | Cerebellum (dorsal vermis and fastigial nuclei) |
Ocular myoclonus (oculopalatal) | Guillain-Mollaret triangle (central tegmental tract in the pons) |
Ocular bobbing | See Table 35.4 |
Square-wave jerks | See Table 35.7 |
Square-wave pulses | Cerebellar outflow tracts (may be associated with rubral tremor) |
AICA, Anterior inferior cerebellar artery; INS, infantile nystagmus syndrome; PAN, periodic alternating nystagmus; SSN, seesaw nystagmus.
Congenital Forms of Nystagmus
The three distinct nystagmus syndromes seen in infancy were renamed by the Classification of Eye Movement Abnormalities and Strabismus (CEMAS) Working Group (2003), sponsored by the National Eye Institute. The first of these syndromes, previously known as congenital nystagmus, is now called infantile nystagmus syndrome (INS); the second, fusion maldevelopment nystagmus syndrome (FMNS), includes the latent form and manifest latent nystagmus; and the third, spasmus nutans syndrome (SNS), remains virtually unchanged from past classifications.
Infantile nystagmus syndrome usually is present from birth but may not be noticed for the first few weeks, or occasionally even years, of life. It may be accompanied by severe visual impairment but is not the result of poor vision. Disorders that, through genetic association, are responsible for poor vision in patients with INS include those designated by the mnemonic of A’s—achiasma (Jansonius et al., 2001), achromatopsia, albinism (both ocular and oculocutaneous forms), amaurotic idiocy of Leber (Leber’s congenital amaurosis), aniridia, aplasia (usually hypoplasia) of the fovea, and aplasia (usually hypoplasia) of the optic nerve—and also congenital cataracts and congenital stationary night blindness. Paradoxical pupil constriction in darkness, particularly in patients with poor vision, suggests an associated retinal or optic nerve disorder. High myopia (uncommon early in life) in infants with INS suggests congenital stationary night blindness, and high hyperopia suggests Leber congenital amaurosis; such retinal disorders can be confirmed by electroretinography. INS sometimes is associated with head titubation (head nodding). INS may be familial and is inherited in an autosomal recessive, X-linked dominant or recessive pattern. Genetic defects identified in some families include a dominant form of INS linked to chromosomal region 6p12 (Kerrison et al., 1998), an X-linked form of INS with incomplete penetrance among female carriers associated with a defect on the long arm of the X chromosome (Kerrison et al., 2001), a deletion in the OA1 gene (ocular albinism) in a family with X-linked INS associated with macular hypoplasia and ocular albinism (Preising et al., 2001), and three mutations in the OA1 gene in families with hereditary nystagmus and ocular albinism (Faugere et al., 2003). Self and Lotery (2007) recently reviewed the molecular genetics of INS.
INS appears horizontal in most patients and may be either pendular or jerk in primary position. Pendular nystagmus often becomes jerk on lateral gaze. The horizontal oscillations may be accentuated during vertical tracking. Oculography with three-dimensional scleral search coils demonstrates that many patients with INS have a torsional component phase-locked with the horizontal component (Averbuch-Heller et al., 2002).
Patients with INS often have good vision unless an associated afferent defect is present (see earlier discussion). In INS, the nystagmus damps with convergence; latent superimposition (an increase in nystagmus amplitude occurring when one eye is covered) may be present. A null zone wherein the nystagmus intensity is minimal may be found; if this zone is to one side, the affected person turns the head to improve vision. The head often “oscillates” as well. Both features—damping of nystagmus with convergence and a null zone—can be used in therapy by changing the direction of gaze with prisms or extraocular muscle surgery to improve head posture and visual acuity. Oculographic recordings usually demonstrate either a sinusoidal (see Fig. 35.17, A) or a slow phase with an increasing exponential waveform (see Fig. 35.17, D). However, in the first few months of life, the waveform of INS may be more variable, evolving into the more classic pattern as the child gets older.
Patients with INS do not experience oscillopsia (an illusory oscillation of the environment) unless a head injury, decompensated strabismus, or retinal degeneration causes a decline in vision, ocular motor function, or both. Prisms or strabismus surgery may correct such late-onset oscillopsia (Hertle et al., 2001a). Approximately 30% of patients with INS have strabismus (Leigh and Zee, 2006).
Rarely in INS, the nystagmus may be in the vertical plane or circumductory, in which the eyes move conjugately in a circular or cycloid pattern. In patients with retinal disorders such as achromatopsia, congenital cone dysfunction, or congenital stationary night blindness, INS can have an asymmetrical horizontal (albinism) or vertical waveform that varies among pendular, downbeat, and upbeat (Shawkat et al., 2000). Occasionally INS may be unilateral, occur later in the teens or adult life, or become symptomatic if changes in the internal or external environment alter foveation stability and duration, causing oscillopsia. Less common patterns of INS such as periodic alternating, upbeat, downbeat, and seesaw nystagmus (SSN) are discussed later.
Fusion maldevelopment nystagmus syndrome, as noted earlier, includes both LN and manifest latent nystagmus (MLN). LN occurs with monocular fixation, that is, when one eye is covered. The slow phase is directed toward the covered eye. The amplitude of the oscillations increases on abduction of the fixating eye. With MLN, the oscillations are present with both eyes open, but only one eye is fixating, vision in the other is ignored or suppressed as a result of strabismus or amblyopia. The nystagmus waveform has a linear (decreasing velocity) slow phase (see Fig. 35.17), which differs from that of true INS. Some patients with LN can suppress it at will.
The pathogenesis of LN may be related to impaired development of binocular vision mechanisms. Under monocular viewing conditions, rhesus monkeys deprived of binocular vision early in life have poor nasal-to-temporal optokinetic responses. The pretectal nucleus of the optic tract (NOT) is necessary for generation of slow-phase eye movements in response to horizontal full-field visual motion. In normal monkeys, the NOT on each side is driven binocularly and responds well to visual stimuli presented to either eye. In monkeys with LN, each NOT is driven mainly by the contralateral eye. Thus in the altered monkeys, when only one eye is viewing, one optic tract nucleus is stimulated, causing an imbalance between each NOT. This imbalance is believed to be responsible for LN (Kaminski and Leigh, 1997). Of interest, under monocular viewing conditions, patients with congenital esotropia have poor temporal-to-nasal pursuit, and some have LN or MLN. Indeed, in esotropic patients, LN may be unmasked in dim light or by shining a bright light at the dominant eye, as when testing pupil reflexes.
Spasmus nutans syndrome is a transient, high-frequency, low-amplitude pendular nystagmus with onset between the ages of 6 and 12 months that lasts approximately 2 years but occasionally can be as long as 5 years. The direction of the oscillations may be horizontal, vertical, or torsional; the oscillations often are dysconjugate, asymmetrical, even monocular, and variable. SNS may be associated with torticollis and head titubation, and these three features constitute the spasmus nutans triad. The titubation has a lower frequency than that of the nystagmus and thus is not compensatory. Patients can improve vision by vigorously shaking the head, presumably to stimulate the vestibulo-ocular reflex and suppress or override the ocular oscillations. Some patients may have esotropia. Clinically, spasmus nutans is distinguished from INS and FMNS by its intermittency, high frequency, vertical component, and dysconjugacy (Leigh and Zee, 2006).
Although spasmus nutans is a benign and transient disorder, it must be distinguished from acquired nystagmus caused by structural lesions involving the anterior visual pathways in approximately 2% of patients. In the latter situation, a careful ophthalmological examination reveals clinical evidence such as impaired vision, a relative afferent pupillary defect, or optic atrophy. Also, retinal disorders may masquerade as spasmus nutans; paradoxical pupil constriction in darkness is suggestive, but an electroretinogram is confirmatory. Kim and associates (2003) reported spasmus nutans in a patient with congenital ocular motor apraxia and cerebellar vermian hypoplasia.
Pendular Nystagmus
Pendular nystagmus (see Fig. 35.17, A) has a sinusoidal waveform and usually is horizontal. It may be either congenital or acquired. Large-amplitude (“searching”) pendular nystagmus usually is associated with poor vision as a result of afferent disorders such as optic neuropathy, which can be unilateral, and retinal disorders (see INS). Acquired pendular nystagmus (APN) typically has horizontal, vertical, and torsional components, although one may be dominant. The oscillations of each eye may be so different that the nystagmus may appear monocular clinically (Leigh and Zee, 2006). The most common cause of APN is multiple sclerosis (MS), followed by brainstem vascular disease; MS patients frequently have optic neuropathy that usually is worse in the eye with the larger oscillations. Other disorders of myelin, including Cockayne syndrome, Pelizaeus-Merzbacher disease, peroxisomal disorders, disorders associated with toluene abuse, as well as spinocerebellar disease, hypoxic encephalopathy, and Whipple disease, can cause pendular nystagmus.
Pendular nystagmus probably results from disruption of normal feedback from cerebellar nuclei to the NI (Das et al., 2000). This is in keeping with the predominance of paramedian pontine lesions on magnetic resonance imaging (MRI) in patients with horizontal pendular nystagmus (Lopez et al., 1995, 1996) and with the predominance of medullary lesions in those with torsional pendular nystagmus. The rhythmic pendular oscillations may be the result of deafferentation of the inferior olive by lesions involving the central tegmental tracts, medial vestibular nuclei, or paramedian tracts, causing instability in the system. Disruption of prenuclear ocular motor pathways necessary for orthotropia (and conjugacy) may be a factor as well. A similar mechanism may be responsible for oculopalatal myoclonus (discussed later in this section).
Pendular vergence nystagmus, previously called convergent-divergent nystagmus, a rare variant of acquired pendular nystagmus, is dysconjugate and occurs in patients with MS, brainstem stroke, Chiari malformations, cerebral Whipple disease (see Oculomasticatory Myorhythmia), occasionally palated myoclonus, and progressive ataxia (Averbuch-Heller et al., 1995). The eyes oscillate, mainly horizontally, in opposite directions simultaneously, although they sometimes form circular, elliptical, or oblique trajectories, depending on the phase relationship of the horizontal, vertical, and torsional vectors responsible for the oscillations.
Cyclovergent nystagmus (i.e., dysconjugate torsional nystagmus in which the upper poles of the eyes oscillate in opposite directions) was detected by scleral search coil oculography in a patient with progressive ataxia and palatal myoclonus (Averbuch-Heller et al., 1995). On rare occasions, cyclovergent nystagmus may be observed clinically.
Vertical pendular nystagmus closely resembles the vertical ocular oscillation associated with palatal myoclonus (the oculopalatal syndrome) (Dell’Osso and Daroff, 1999a) and may be a form of the same disorder, which also results from lesions of the deep cerebellar nuclei and their connections.
Elliptical pendular nystagmus, with a larger vertical component and superimposed or interposed upbeat nystagmus, is characteristic of Pelizaeus-Merzbacher disease. This nystagmus can be difficult to discern with the naked eye. It is seen more easily with an ophthalmoscope, but oculography using scleral search coils may be necessary to detect it (Dell’Osso and Daroff, 1999b).
Oculomasticatory Myorhythmia
Oculomasticatory myorhythmia, described in patients with Whipple disease and, to date, pathognomonic of that disorder, consists of continuous rhythmic jaw contractions synchronous with dissociated pendular vergence oscillations. It may be associated with supranuclear vertical gaze palsy, altered mentation, somnolence, mild uveitis, or retinopathy (Knox et al., 1995). Myorhythmia of the face and arms is described in Hashimoto encephalopathy (Erickson et al., 2002).
Gaze-Paretic Nystagmus
Gaze-paretic nystagmus, the most common type of nystagmus, usually is symmetrical and evoked by eccentric gaze to either side but is absent in the primary position. Frequently it is present on eccentric vertical gaze, with upward-beating nystagmus on upgaze and downward-beating nystagmus on downgaze. It may be asymmetrical with asymmetrical central nervous system (CNS) disease or with disorders such as myasthenia. It has a jerk waveform, with the fast phase in the direction of gaze. Oculographic recordings show a decreasing exponential slow phase (see Fig. 35.17, C). Gaze-paretic nystagmus results from dysfunction of the NI and commonly is caused by alcohol or drug intoxication (by anticonvulsants and tranquilizers). When it is caused by structural disease, it tends to be asymmetrical.
Vestibular Nystagmus
Vestibular nystagmus results from damage to the labyrinth, vestibular nerve, vestibular nuclei, or their connections in the brainstem or cerebellum. Vestibular nystagmus may be divided into central and peripheral forms on the basis of the associated features outlined in Chapter 37. Peripheral vestibular nystagmus, caused by dysfunction of the vestibular end organ or nerve, has a linear slow phase (see Fig. 35.17, B), whereas with central lesions, the slow phase may be variable. Usually, peripheral vestibular nystagmus is associated with severe vegetative symptoms and signs including nausea, vomiting, perspiration, and diarrhea; it also may be associated with hearing loss and tinnitus. With central vestibular nystagmus, vegetative symptoms are less severe, but other neurological features may be present, such as headache, ataxia, dysconjugate gaze, and pyramidal tract signs (see Chapter 19).
Physiological Nystagmus
Physiological (endpoint) nystagmus is a jerk nystagmus observed on extreme lateral or upward gaze. If the bridge of the nose obstructs the view of the adducting eye, physiological nystagmus may be dysconjugate because the amplitude is greater in the abducting eye. A torsional component is sometimes seen. Physiological nystagmus is distinguished from pathological nystagmus by its symmetry on right and left gaze and by the absence of other neurological features. It is not present when the angle of gaze is less than 30 degrees from primary position. Oculographic recordings primarily demonstrate a linear slow phase (see Fig. 35.17, B) and may detect transient small-amplitude rebound nystagmus.
Dysconjugate Nystagmus
Dysconjugate (dissociated) nystagmus occurs when the ocular oscillations are out of phase (in different directions). It is seen with internuclear ophthalmoplegia, other brainstem lesions (see earlier discussion of pendular vergence nystagmus under Pendular Nystagmus), and spasmus nutans. Monocular nystagmus also is dysconjugate and may be associated with amblyopia and other forms of vision loss (Box 35.11).
Box 35.11 Causes of Monocular Nystagmus
Monocular Nystagmus
Monocular nystagmus may be pendular or jerk and also may be horizontal, vertical, or oblique. Oculographic recordings may reveal small-amplitude oscillations in the fellow eye. Monocular nystagmus may occur in patients with alternating hemiplegia of childhood (Egan, 2002), amblyopia, strabismus, monocular blindness, spasmus nutans, internuclear ophthalmoplegia, MS, or (rarely) seizures (Grant et al., 2002), and of course when the other eye is completely ophthalmoplegic or absent.
The Heimann-Bielschowsky phenomenon is a rare form of monocular vertical pendular oscillation, with a frequency of 1 to 5 Hz, that occurs in an amblyopic eye or after acquired monocular vision loss, such as with cataract. In the latter situation, it may be reversible after successful treatment of the underlying condition or with gabapentin (Rahman et al., 2006).
Superior oblique myokymia may be mistaken for a monocular torsional or vertical nystagmus (see Box 35.11).
Upbeat Nystagmus
Upbeat nystagmus is a spontaneous jerk nystagmus with the fast phase upward while the eyes are in primary position (Hirose et al., 1998). It is attributed to interruption of the anterior semicircular canal projections, which are responsible for the upward vestibulo-ocular reflex, causing downward drift of the eyes with corrective upward saccades. The amplitude and intensity of the nystagmus usually increase on upgaze. This finding strongly suggests bilateral paramedian lesions of the brainstem, usually at the pontomedullary or pontomesencephalic junction, the paramedian tract neurons in the lower medulla, or midline cerebellum (vermis). Rarely, upbeat nystagmus may be congenital, or it may result from Wernicke encephalopathy or intoxication with anticonvulsants, organophosphates, lithium, nicotine, or thallium (author’s personal observation). In infants, upbeat nystagmus may be a sign of anterior visual pathway disease, such as Leber congenital amaurosis (see Chapter 36), optic nerve hypoplasia, aniridia, or cataracts. Small-amplitude upbeat nystagmus may be seen in persons who are carriers of blue-cone monochromatism, whereas affected patients may have intermittent pendular oblique nystagmus. If the intensity of upbeat nystagmus diminishes in downgaze, base-up prisms over both eyes may improve the oscillopsia; gabapentin also may be helpful (Table 35.4). For an extensive list of causes of upbeat nystagmus, see Leigh and Zee’s textbook (2006).
Table 35.4 Treatment of Nystagmus and Non-Nystagmus Oscillations*
Nystagmus Syndrome | Treatment |
---|---|
Infantile nystagmus syndrome | Prisms |
Contact lenses | |
Extraocular muscle surgery | |
Kestenbaum-Anderson procedure | |
Tenotomy (experimental) | |
Acetazolamide (Thurtell et al., 2010) | |
Gabapentin (Shery et al., 2006) | |
Gene therapy (experimental) when the nystagmus is associated with retinal disorders (Leigh and Zee, 2006) | |
APN | Trihexyphenidyl, benztropine, clonazepam, gabapentin, isoniazid, memantine† (Leigh and Zee, 2006), valproate, diethylpropion hydrochloride, tenotomy followed by memantine (Tomsak et al., 2006) |
Convergence-evoked horizontal | Base-in prisms nystagmus |
Downbeat nystagmus | Base-out prisms (if nystagmus damps with convergence) |
Base-down prisms over both eyes if intensity of nystagmus diminishes in upgaze | |
Baclofen, clonazepam, gabapentin, scopolamine, 4-aminopyridine, 3,4-diaminopyridine | |
PAN: | |
Congenital | Dextroamphetamine, baclofen (occasionally), 5-HT |
Acquired | Baclofen, phenytoin, memantine |
Upbeat nystagmus | Base-up prisms over both eyes if intensity of nystagmus diminishes in downgaze |
Baclofen gabapentin, 4-aminopyridine, memantine (Thurtell et al., 2010), thiamine | |
Ocular myoclonus | Chronically patch one eye |
Baclofen, carbamazepine, cerulein, clonazepam, memantine, gabapentin, scopolamine, trihexyphenidyl, valproate | |
SSN | Baclofen, clonazepam, gabapentin or memantine (Huppert et al., 2011), base-out prisms |
Hemi-SSN | Memantine (Thurtell et al., 2010) |
Ictal nystagmus | AEDs |
Episodic nystagmus: | |
Episodic ataxia-1 | Acetazolamide |
Episodic ataxia-2 | Acetazolamide, 4-aminopyridine (Strupp et al., 2011) |
Oculomasticatory myorhythmia | Antibiotics for Whipple disease; consider gabapentin or memantine |
Torsional nystagmus | Memantine (Thurtell et al., 2010) |
Non-Nystagmus Ocular Oscillations | Treatment |
Opsoclonus | Treat underlying condition when possible, ACTH, vitamin B1, clonazepam, gabapentin, ondansetron, steroids; if paraneoplastic, protein A immunoabsorption |
Superior oblique myokymia | Carbamazepine, gabapentin, oxcarbazepine, other AEDs, topical beta-blockers, memantine, base-down prism over the affected eye, muscle/tendon surgery, microvascular decompression |
Ocular neuromyotonia | Carbamazepine |
Microflutter | Propranolol, verapamil |
Saccadic intrusions | Memantine (Serra et al., 2008) |
Square-wave jerks | Valproate (Traccis et al., 1997), amphetamines, barbiturates, diazepam, clonazepam, memantine (Leigh and Zee, 2006) |
Square-wave oscillations | Valproate (Traccis et al., 1997) |
ACTH, Adrenocorticotropic hormone; AEDs, antiepileptic drugs; 5-HT, 5-hydroxytryptamine; APN, acquired pendular nystagmus; PAN, periodic alternating nystagmus; SSN, seesaw nystagmus.
* Treat underlying cause when possible.
† Memantine is reported to exacerbate MS (Villoslada el al., 2009).
Downbeat Nystagmus
Downbeat nystagmus may be apparent only with changes in posture (positional downbeat nystagmus), particularly the head-hanging position. Downbeat nystagmus results from either damage to the commissural fibers between the vestibular nuclei in the floor of the fourth ventricle or bilateral damage to the flocculus that disinhibits the VOR in pitch; frequently it occurs with structural lesions at the craniocervical junction (Box 35.12). A thorough investigation for such should be made. MRI of the foramen magnum region (in the sagittal plane) is the investigation of choice. Olson and Jacobson suggested that in some cases of unexplained downbeat nystagmus, the cause is a radiographically occult infarction (2001); however, lesions that cause downbeat nystagmus are bilateral (Brandt and Dietrich, 1995). Causes of downbeat nystagmus are listed in Box 35.12.
Box 35.12 Causes of Downbeat Nystagmus
The treatment of downbeat nystagmus involves correction of the underlying cause when possible. When downbeat nystagmus damps on convergence, it may be treated successfully with base-out prisms, reducing the oscillopsia and improving the visual acuity. Baclofen, clonazepam, or 4-aminopyridine may also help (see Table 35.4); 4-aminopyridine is more effective in downbeat nystagmus associated with cerebellar atrophy rather than structural lesions (Huppert et al., 2011).
Both upbeat and downbeat nystagmus may be altered in amplitude and direction by a variety of maneuvers (e.g., convergence, head tilting, changes in posture) and by 3,4-diaminopyridine (Leigh and Zee, 2006).
Periodic Alternating Nystagmus
When PAN is congenital, it may be associated with albinism. In one series of patients with congenital PAN, none had pure vertical oscillations, even during the transition period (Gradstein et al., 1997). Although not all patients with acquired PAN have vertical nystagmus during the transition period, its presence may distinguish acquired from congenital PAN (personal observation); this finding does not obviate further evaluation when appropriate. Transient episodes of PAN were provoked by attacks of Ménière disease in a patient with a hypoplastic cerebellum and an enlarged cisterna magna (Chiu and Hain, 2002). Episodic PAN can be a manifestation of a seizure (see later discussion of ictal nystagmus). An atypical form of paroxysmal alternating skew deviation and nystagmus has followed partial destruction of the inferior uvula and adjacent pyramis during biopsy of a suspected brainstem glioma (Radtke et al., 2001).
Lesions of the cerebellar nodulus cause loss of γ-aminobutyric acid (GABA)-mediated inhibition from the Purkinje cells to the vestibular nuclei, impairing the velocity storage mechanism. It is likely that overcompensation in feedback loops causes cyclical firing between reciprocally connected inhibitory neurons and generates the unusual oscillations of acquired PAN. Affected patients have hyperactive vestibular responses and poor vestibular fixation suppression, attributed to involvement of the nodulus and uvula (Leigh and Zee, 2006).
Treatment of PAN should be directed at correcting the cause, such as a Chiari malformation, when possible. Baclofen, a GABA-b agonist, replaces the missing inhibition and usually is effective in the acquired form of the disease and occasionally in the congenital form. Dextroamphetamine resulted in clinical improvement in a patient with PAN who also had rod-cone dystrophy and strabismus (Hertle et al., 2001b).
Rebound Nystagmus
Rebound nystagmus is a horizontal gaze–evoked nystagmus in which the direction of the fast phase reverses with sustained lateral gaze or beats transiently in the opposite direction when the eyes return to primary position (Video 35.3, available at www.expertconsult.com). The latter is occasionally a physiological finding and caused by dysfunction of the cerebellum or the perihypoglossal nuclei in the medulla. Occasionally, rebound nystagmus may be torsional.
Convergence-Evoked Nystagmus
Convergence-evoked nystagmus is an unusual ocular oscillation, usually pendular, induced by voluntary convergence (see earlier discussion of pendular vergence nystagmus under Pendular Nystagmus). The movements may be conjugate or dissociated. This condition may be congenital or acquired, such as in patients with MS. A jerk form occurs with Chiari type I malformations. Convergence-evoked vertical nystagmus (upbeat more common than downbeat) also occurs. Convergence-evoked nystagmus should be distinguished from voluntary nystagmus and from convergence retraction nystagmus (see Saccadic Intrusions and Other Non-Nystagmus Ocular Oscillations, later).
Seesaw Nystagmus
Seesaw nystagmus is a spectacular ocular oscillation in which one eye rises and intorts as the other eye falls and extorts. The waveform appears pendular. The oscillations usually become faster and smaller on upgaze but slower and larger on downgaze; they may cease in darkness. Disordered control of the normal ocular counter-rolling reflex may be responsible. Bitemporal hemianopia, caused by acquired chiasmal defects or impaired central vision, plays a significant role in generating SSN. Disruption of retinal error signals necessary for VOR adaptation, which normally are conveyed to the inferior olive by the chiasmal crossing fibers, results in an unstable visuovestibular environment. Fixation and pursuit feedback accentuate this instability, causing synchronous oscillations of floccular Purkinje cells, which relay to the nodulus, resulting in SSN. This mechanism also may be the basis for the ocular oscillations of oculopalatal myoclonus. The observations of SSN and INS in achiasmatic humans and achiasmatic Belgian sheepdogs support this hypothesis (Dell’Osso and Daroff, 1998). Significantly, the onset of both SSN and oculopalatal myoclonus may be delayed after CNS lesions.
SSN occurs with lesions in the region of the mesodiencephalic junction, particularly the zona incerta and the interstitial nucleus of Cajal. Congenital SSN may be associated with a superimposed horizontal pendular nystagmus; some patients with congenital SSN may be achiasmatic or have septo-optic dysplasia. Acquired SSN may be associated with suprasellar tumors, Joubert syndrome, and Leigh disease, particularly the jerk form described later. Acquired pendular SSN may be accompanied by a bitemporal hemianopia from trauma or an expanding lesion in the third ventricular region, or by severe loss of central vision due to disorders such as choroiditis, cone-rod dystrophy, whole-brain radiation and intrathecal methotrexate, and vitreous hemorrhage (Dell’Osso and Daroff, 1998). Transient (latent) SSN may occur for a few seconds after a blink, perhaps because of loss of fixation, in patients with chiasmal region lesions. If SSN damps with convergence, base-out prisms may be helpful. Baclofen also may be beneficial in SSN.
Torsional (Rotary) Nystagmus
In torsional nystagmus (TN), the eye oscillates in a pure rotary or plane. TN may be present in primary position or with either head positioning or gaze deviation. Usually it results from lesions in the central vestibular pathways. Pure TN occurs only with central vestibular dysfunction, whereas mixed torsional-linear nystagmus may occur with peripheral vestibular disease. When the waveform of TN is pendular (i.e., torsional pendular nystagmus), the lesion usually is in the medulla (Lopez et al., 1995). Skew deviation frequently coexists with TN.
In patients with lesions of the middle cerebellar peduncle, TN with a jerk waveform—similar to jerk SSN—may be evoked by vertical pursuit eye movement and during fixation suppression of the vertical VOR. The direction of the fast phase changes with pursuit direction; it usually is toward the side of the lesion on downward pursuit and away from the side of the lesion on upward pursuit (FitzGibbon et al., 1996).
Ictal Nystagmus
Ictal nystagmus often accompanies adversive seizures and beats to the side opposite the focus. Ictal nystagmus may be associated with transient pupillary dilation of either the abducting or adducting eye (Masjuan et al., 1997). Pupillary oscillations synchronous with the nystagmus may occur rarely. Ictal nystagmus associated with unformed visual hallucinations, homonymous hemianopia, and unusual MRI findings occurs in patients with non-ketotic hyperglycemia (Lavin 2005). Nystagmus as the only motor manifestation of a seizure is rare; however, there are reports of isolated ictal nystagmus, such as occurs in patients with vivid ictal visual hallucinations. Monocular nystagmus associated with ipsilateral hemianopic visual hallucinations in a binocular patient can occur as the only manifestation of a partial seizure caused by a focal discharge in the contralateral medial occipital lobe (Grant et al., 2002). It is difficult to draw any conclusion clinically regarding the location of the seizure discharge in these patients, because seizure foci were found in occipital, parietal, temporal, and frontal areas. Usually the nystagmus is horizontal, but vertical nystagmus, mainly in comatose patients, was reported on occasion. In comatose patients, periodic eye movements should alert the physician to the possibility of status epilepticus; indeed, PAN associated with periodic alternating gaze deviation and periodic alternating head rotation may be a manifestation of a seizure (Moster and Schnayder, 1998). The monocular abducting nystagmus seen in alternating hemiplegia of childhood is likely ictal in origin.
Brun Nystagmus
Brun nystagmus occurs in patients with large cerebellopontine angle tumors. The nystagmus is bilateral but asymmetrical, with a jerk waveform. It is characterized by large-amplitude, low-frequency fast phases on gaze toward the side of the lesion but small-amplitude, high-frequency fast phases on gaze to the other side. The ipsilateral large-amplitude (coarse) nystagmus has an exponentially decreasing velocity slow phase attributed to compression of the brainstem NI, which includes the ipsilateral medial vestibular nucleus. The contralateral small-amplitude, high-frequency nystagmus has a linear slow phase attributed to ipsilateral vestibular dysfunction (see Fig. 35.17). Occasionally an anterior inferior cerebellar artery territory stroke can cause Brun nystagmus (personal observation).
Episodic Nystagmus (Periodic Nystagmus)
Episodic nystagmus is associated with disorders in which the patient has paroxysmal episodes of vertigo, ataxia, and nystagmus lasting up to 24 hours. The nystagmus may be torsional, vertical, or dissociated. The frequency of attacks varies, ranging from once a day to only a few times per year. Such periodic ataxias occur in patients with hereditary inborn errors of metabolism, in an autosomal dominant form without any detectable metabolic defect (channelopathy), in some forms of spinocerebellar degeneration, in basilar migraine, and in MS. Acetazolamide or 4-aminopyridine may alleviate or prevent attacks in the familial form (see Chapter 72).
Perverted Nystagmus
Perverted vestibular nystagmus occurs in a plane different from that of the applied vestibular stimulus and is caused by lesions involving central vestibular connections. For example, horizontal head shaking in a patient with MS caused transient downbeat nystagmus (Minagar et al., 2001).
Lid Nystagmus
Lid nystagmus, characterized by rhythmic jerking movements of the upper eyelids, occurs in the following situations: (1) synchronous with vertical ocular nystagmus, (2) synchronous with the fast phase of gaze-evoked horizontal nystagmus in some patients with the lateral medullary syndrome, (3) evoked by horizontal gaze in some patients with midbrain tumors that injure the M-group of neurons adjacent to the riMLF, and (4) during voluntary convergence (the Pick sign) in some patients with medullary or cerebellar disease (Dell’Osso and Daroff, 1999a; Leigh and Zee, 2006).
Treatment
Treatments used for the different types of nystagmus are summarized in Table 35.15. Underlying causes should be rectified where possible, and visual acuity should be corrected when necessary. With the exception of INS, in which prisms, surgery, and contact lenses are helpful, results of treatment of other forms of nystagmus are less effective; Occasionally, prisms are helpful in acquired nystagmus. Various pharmacological agents are used. For extensive reviews, see the works of Leigh and Zee (2006), Stahl et al. (2002), and Thurtell et al. (2010).