14 Nervous system
The neurological history
The essentials of history taking have been covered elsewhere, but there are some points particularly salient to neurological conditions. The repertoire of neurological symptoms is actually quite limited, though perhaps larger than that of other specialties. Box 14.1 lists the usual ones, and each should be specifically enquired about in taking a neurological history.
Box 14.1 Neurological symptoms
Cognitive symptoms, especially memory impairment
Alteration of perception, including déjà vu
‘Positive’ visual symptoms, including components of migraine auras, unformed and formed hallucinations
More than double vision (polyopia, palinopsia)
Oscillopsia (a visual sensation of stationary objects swaying back and forth)
Abnormal movements of muscles (including cramp, fasciculations)
Abnormal movements of parts of the body (including tremor, dystonia, myoclonus)
Impairment of control of limbs
The three most common causes of attacks of reduced consciousness or awareness that lead to neurological consultations are: neurocardiogenic syncope, epilepsy and psychogenic non-epileptic attacks. It is far more informative to hear a description from a witness than to request potentially misleading and inappropriate investigations. In the setting of the emergency department, obtaining the witness’s account may involve contact by telephone; this is time well invested. Table 14.1 summarizes some points which help to distinguish syncope from seizures.
Table 14.1 Points which help to distinguish syncope from seizures involving loss of consciousness. Minor injury, incontinence and sleepiness after the event do not distinguish well
The symptoms of focal seizure activity which precede a secondarily generalized attack may or may not be remembered after the attack is over
Vocalization at onset of generalized convulsion (GC)
Postictal amnesia – paramedical staff present when patient ‘comes round’, or patient ‘comes round’ in hospital (although he walked to the ambulance)
The most frequent symptom leading to neurological referral is headache. It is also a common reason for attending an emergency department. The vast majority of headaches are not caused by life-threatening disorders such as aneurysmal subarachnoid haemorrhage, meningitis or brain tumour, but are caused by common primary headache syndromes, particularly migraine. Table 14.2 outlines some of the features which may help to distinguish headaches of different sorts.
Table 14.2 Headaches: points to consider in the history
Aspect of history | Feature | Diagnosis |
---|---|---|
Region/location | Focal, retro-orbital | Cluster headache |
Migraine | ||
Retro-orbital lesion | ||
Focal, frontal | Sinus pathology | |
Unilateral | Migraine | |
Chronic paroxysmal hemicrania (CPH) | ||
Hemicrania continua | ||
Generalized | Migraine | |
Tension-type headache | ||
Temporal aspects | >50% of days | Chronic daily headache (chronic migraine; tension-type headache) |
Attacks of hours/days | Migraine | |
Attacks of up to 1 hour | Cluster headache | |
Many attacks, lasting minutes | CPH | |
Attacks of seconds | Trigeminal neuralgia | |
Worse on waking | Raised intracranial pressure | |
Sleep apnoea | ||
New, acute/subacute onset | Meningitis | |
Abscess | ||
Encephalitis | ||
Explosive onset, severe | Subarachnoid haemorrhage (SAH) | |
Character and severity | Tight band around head, bland, featureless, not very severe | Tension-type headache |
Throbbing, moderately severe | Migraine | |
Extremely severe, constant | Cluster headache | |
Severe, stabbing, lancinating | Trigeminal neuralgia | |
Provoking/relieving factors | Provoked by alcohol | Migraine |
Cluster headache | ||
Occur at night, start in sleep | Migraine | |
Cluster headache | ||
Hypnic headache | ||
Relieved by sleep | Migraine | |
Triggered by touching or moving the face | Trigeminal neuralgia | |
Caused by cough | Chiari malformation | |
Idiopathic cough headache | ||
Exertion | Exacerbates migraine | |
Benign exertional headache | ||
Orgasm | Benign coital headache (SAH has to be excluded in a severe single attack) | |
Associated symptoms | Nausea, vomiting, photophobia, phonophobia | Migraine |
Migraine aura (visual, sensory, etc.) | Migraine | |
Neck stiffness, photophobia, vomiting, symptoms of fever | Meningitis | |
Tear production ipsilateral to a unilateral headache | Migraine | |
Cluster headache | ||
SUNCT* | ||
Ipsilateral conjunctival injection | Cluster headache | |
SUNCT* | ||
Visual obscurations | Raised intracranial pressure with papilloedema | |
Persistent focal neurological symptoms | Intracranial lesion | |
General health | Indications of systemic neoplasia | Metastasis |
Polymyalgia and weight loss | Giant cell arteritis |
* SUNCT, short-lasting, unilateral, neuralgiform headache attacks with conjunctival injection and tearing (a rare disorder).
Most organic neurological disorders which give rise to sensory symptoms involve structural or functional damage to nerves somewhere, whether it be in peripheral nerves, nerve roots, spinal cord or brain. Hypersensitivity (hyperaesthesia) to all modalities of sensation is therefore improbable or impossible. Patients who appear to have very sensitive skin as a result of a lesion (e.g. herpes zoster radiculitis) have combinations of paraesthesia, hypoaesthesia, dysaesthesia, allodynia, hyperalgesia and hyperpathia. Some of these terms are not without their ambiguities. Table 14.3 provides a definition for each.
Table 14.3 Nomenclature of cutaneous sensory symptoms
Hypoaesthesia | Reduced cutaneous sensation of any modality |
Paraesthesia | Spontaneous abnormal sensation including, tingling, pins and needles and pain |
Neuralgia | Pain in the distribution of a nerve or nerve root |
Dysaesthesia | An abnormal perception of a sensory stimulus, e.g. touch causes tingling or pain |
Allodynia | Pain caused by a stimulus that does not normally cause pain |
Hyperalgesia | An abnormally intense perception of a mildly painful stimulus |
Hyperpathia | Perseveration, augmentation and, on occasion, spread of pain |
The pain threshold is normal or sometimes high | |
In this, the threshold for perceiving pain may be raised and there may be delay in perceiving a painful stimulus, but once perceived, the pain is severe and prolonged and may spread | |
Hyperaesthesia | An ambiguous term, best avoided |
Cranial nerve examination
The optic (II) nerves
The optic nerve runs from the back of the globe of the eye to the apex of the orbit and into the skull through the optic canal to the optic chiasm, where it is joined by the optic nerve from the other eye. Directly above the optic chiasm is the hypothalamus. Directly below is the pituitary gland. The pituitary stalk runs from the hypothalamus to the pituitary gland just behind the optic chiasm, between the optic tracts. Sensory afferents from all points of the retina run in the nerve-fibre layer on the inner surface of the retina to enter the optic nerve at the optic disc. Fibres from the temporal retina (nasal visual half-field) are placed laterally while those from the nasal retina (temporal visual half-field) are medial. Fibres from the upper half of the retina run in the upper half of the optic nerve. At the optic chiasm, fibres from the nasal half of the retina (temporal visual half-field) cross (decussate) to the contralateral optic tract, while the fibres from the temporal half of the retina do not cross but proceed posteriorly into the ipsilateral optic tract (Fig. 14.1). Starting within the chiasm and continuing further posteriorly within the optic tract, fibres which convey matching information from each eye (i.e. homonymous fibres, representing equivalent parts in the temporal retina for one eye, nasal retina for the other eye) become aligned with each other. Thus, each optic nerve conveys information from its respective eye, but every part of the sensory visual system behind the optic chiasm on each side deals with vision for the contralateral binocular visual half-field.
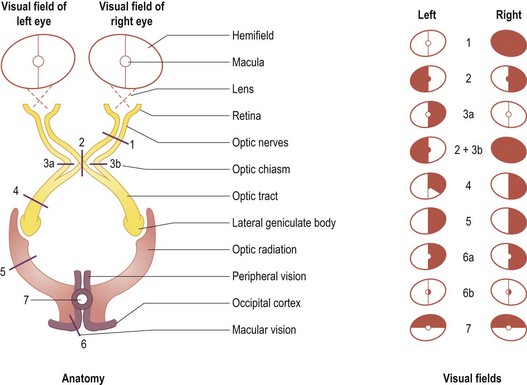
2: Optic chiasm lesion (bitemporal hemianopia).
3a or 3b: Uniocular nasal hemianopia (rare).
3a plus 3b: Binasal hemianopia (very rare).
4: Optic tract lesion (incongruous homonymous hemianopia).
5: Visual radiation (homonymous quadrantanopia or hemianopia).
6a: Occipital cortex lesion sparing the occipital pole (homonymous hemianopia with macular sparing).
6b: Occipital pole lesion (homonymous paracentral hemiscotoma).
7: A bilateral occipital cortex lesion (homonymous altitudinal hemianopia).
The pupils
Other common pupillary abnormalities of neurological relevance
Simple (physiological) anisocoria (inequality of pupil size): this is common. The pupillary inequality is not marked and the reactions to light and accommodation are normal.
Tonic pupil: this is seen in Holmes Adie syndrome, a relatively benign polyneuropathy comprising a lesion of the ciliary ganglion and a degree of loss of tendon reflexes. An acute Adie pupil is enlarged, does not react to light and there is a slow constriction to accommodation. Redilatation of the pupil after accommodation is delayed such that, temporarily, the normal pupil may be larger than the affected one.
Argyll Robertson pupils: these are small, irregular, unequal pupils which do not react to light but do to accommodation. They were seen in advanced syphilis. Without the irregularity but with the other features, diabetic small vessel disease is currently the most common cause.
The pupils in coma: this is important but covered elsewhere (Ch. 8).
The oculomotor (III), trochlear (IV) and abducens (VI) nerves – eye movements
The nucleus for the third cranial nerve is in the midbrain (Fig. 14.2) and emerges ventrally (anteriorly), medial to the cerebral peduncle, passing forward through the cavernous sinus to the superior orbital fissure. In the orbit, the superior ramus supplies superior rectus and levator palpebrae superioris. The inferior ramus supplies inferior rectus, inferior oblique and medial rectus and parasympathetic fibres from the inferior ramus pass to the ciliary ganglion and thence to the ciliary muscle and the pupil sphincter.
The sixth nerve nucleus is beneath the floor of the fourth ventricle in the pons (Fig. 14.3). Nerve fibres run forward (ventrally) through the pons emerging at its lower border, then up the skull base and forward through the cavernous sinus to the superior orbital fissure and into the orbit to supply the lateral rectus muscle. The nerve is long, thin and very susceptible to dysfunction, most notably in the setting of raised intracranial pressure of any aetiology, which may give rise to either unilateral or bilateral sixth nerve lesions. This is referred to as a ‘false localizing sign’, since a focal mass lesion causing raised intracranial pressure may be remote from the sixth nerves and their nuclei or there may be no focal cause of the raised pressure at all (e.g. idiopathic intracranial hypertension).
Table 14.4 and Figure 14.4 outline the actions of each eye muscle.
Examination of eye movements
Diplopia testing
If a patient complains of double vision, first establish that it is true diplopia and not monocular diplopia. In patients who have obvious, easily visible paresis of movement of one or both eyes, the reason for diplopia is self-evident (Fig. 14.5).
Diplopia develops with even very subtle misalignment of the eyes, which cannot be seen on simple inspection. In this situation, if the ophthalmoparesis affects just one eye, it is possible to work out which eye muscles are underactive by diplopia testing. The true image is that generated by the eye with normal movements. The false image is that generated by the eye with the paretic muscle or muscles. For example, if a patient develops double vision on looking to the right, with horizontal separation of the images, the false image will be the one further out to the right. This is true whether it is the right eye which does not abduct adequately (right lateral rectus weakness) or if it is the left eye which does not adduct adequately (left medial rectus weakness). If this does not seem immediately clear, consider the extreme case: one eye moves, the other does not. An image (an examiner’s finger or a white pinhead) moves to the patient’s right. The image remains in the middle of the field of the eye which moves, but moves progressively to the right of the field of the eye which does not. The same rule applies in all directions of gaze. Diplopia is always maximal in the direction in which the weak muscle has its purest action (see Table 14.4).
The severity of the diplopia should be assessed in eight positions: looking to left and right, up and down, and obliquely up and down to the left and obliquely up and down to the right. To work out which muscle is underactive, where the diplopia is maximal, cover each eye in turn and get the patient to tell you which of the two images disappears. Inconsistent answers are common, however, and the assistance of an ophthalmologist or optometrist is frequently desirable. The features of lesions of the third, fourth and sixth cranial nerves are summarized in Table 14.5.
Table 14.5 The effects of lesions of the oculomotor (III), trochlear (IV) and abducens (VI) nerves
Affected nerve | Signs | Comment |
---|---|---|
Oculomotor | Paresis of adduction (medial rectus) | The eye becomes abducted because of unopposed action of lateral rectus, and slightly depressed because of action of superior oblique |
Paresis of elevation (superior rectus and inferior oblique) | The pure depressor action of superior oblique cannot be tested because the eye cannot be adducted | |
Intorsion of the eye on attempted down gaze indicates intact trochlear nerve and superior oblique function | ||
Paresis of depression (inferior rectus) | ||
Ptosis due to paresis of levator palpebrae superioris | With complete ptosis, there is of course no diplopia | |
Dilated, unreactive pupil | This feature is not present in pupil-sparing lesions (microvascular lesions of nucleus or nerve) | |
Trochlear | Paresis of superior oblique | Extorsion of the eye due to unopposed action of inferior oblique leads to diplopia such that a vertical line looks V-shaped |
The patient compensates with a head tilt to the side opposite the lesion, intact intorsion on that side tending to correct the diplopia | ||
Abducens | Paresis of lateral rectus | Horizontal diplopia |
In assessing patients who have double vision, it is best first to establish which muscles appear to be weak, and then try to decide what the nature of the problem is likely to be, taking into consideration all the physical signs. Thus, impairment of eye movements in one eye in combination with proptosis of that eye may occur because of mechanical restriction of eye movements by an intraorbital lesion. Weakness of muscles in both eyes with different patterns of involvement of the muscles in the two eyes is likely to be due to a disorder of the muscles themselves (orbital myositis, thyroid eye disease) or due to ocular or generalized myasthenia. The pupils will not be involved. Bilateral, asymmetrical combinations of cranial nerve lesions are relatively uncommon (neoplastic infiltration, cranial polyneuritis). Bilateral sixth cranial nerve lesions are common, usually but not exclusively as a feature of raised intracranial pressure. Multiple oculomotor neuropathies in one eye direct attention to the superior orbital fissure and the cavernous sinus (see Box 14.2).
Box 14.2 Cranial nerve involvement in lesions of the cavernous sinus and superior orbital fissure
1 Lesion of the cavernous sinus (e.g. internal carotid aneurysm; internal carotid artery dissection; meningioma):
2 Lesion of the superior orbital fissure (e.g. Tolosa Hunt syndrome):
Horizontal gaze paresis; internuclear ophthalmoparesis
Neural control of voluntary lateral gaze to the right starts in the left cerebral hemisphere, such that a large left cerebral hemisphere lesion may be associated with failure of right gaze and a tendency for the eyes to deviate to the left (the side of the lesion). Output runs to the right paramedian pontine reticular formation (PPRF); hence,a right-sided pontine lesion may involve a right gaze paresis. Output from the right PPRF goes to the right sixth nerve nucleus, resulting in right eye abduction, and across, via the left medial longitudinal fasciculus (MLF), to the left third nerve nucleus, resulting in simultaneous left eye adduction. Attempted right gaze in the setting of a left MLF lesion results in abduction of the right eye, but failure of adduction of the left eye – an internuclear ophthalmoparesis (INO) (see Fig. 14.6). Bilateral MLF lesions give rise to bilateral INO, in which case, with lateral gaze in either direction, only the abducting eye moves normally. Commonly, nystagmus is seen in the abducting eye. The pathway for adducting both eyes for near vision is separate, and sometimes in bilateral INO preservation of adduction of the eyes for near vision can be demonstrated, proving that the problem is not bilateral medial rectus weakness.
Non-paralytic strabismus
Neurologists need to be able to recognize non-paralytic strabismus. Decompensation of a longstanding squint may be a cause of acquired diplopia, but this is an ophthalmological problem and it is covered in Chapter 19.
The trigeminal (V) nerve
Sensory component of the trigeminal nerve
The primary sensory neurones are in the trigeminal ganglion, just behind the cavernous sinus at the apex of the petrous bone. Central projections run in the trigeminal nerve into the pons. Figure 14.7 shows the cutaneous distribution of the three divisions of the trigeminal nerve: ophthalmic (V1), maxillary (V2) and mandibular (V3). These nerves also mediate general sensation inside the mouth and nose and proprioception. The ophthalmic nerve passes through the cavernous sinus and superior orbital fissure. The maxillary nerve also passes through the cavernous sinus, but leaves the inside of the skull through the foramen rotundum. The mandibular nerve passes through the foramen ovale.