Neonatal and pediatric aerosolized drug therapy
After reading this chapter, the reader will be able to:
1. Define key terms that pertain to neonatal and pediatric drug therapy
2. Explain off-label use of aerosolized medications
3. List and describe the most important factors affecting neonatal and pediatric aerosol drug delivery
4. Describe the clinical response of neonatal and pediatric patients to aerosolized drugs
5. Describe special circumstances related to selection of delivery devices for neonatal and pediatric patients
6. Explain the most relevant factors to select the appropriate aerosol delivery device according to age group
7. Describe the implications of compliance and cooperation on the efficiency of aerosol delivery
8. Explain lung deposition of inhaled drugs in pediatric and neonatal intubated patients
Effective administration of aerosol therapy to neonates and pediatric patients is challenging. Inherent anatomic, physiologic, pathophysiologic, and behavioral factors make this age group a unique subpopulation.1–4 The lack of neonatal and pediatric dose labeling for many drugs given by inhaled aerosol complicates aerosol drug dosing. Results of studies on therapeutic aerosols and their lung deposition cannot simply be extrapolated from adult data. The differences between aerosol drug delivery as a form of topical administration and systemic administration of drugs are not appreciated in many instances, causing aerosol doses to be modified in neonates and children as if they were given systemically. Neonates and toddlers cannot perform an inhalation maneuver; they are usually nasal breathers and often get distressed during the administration of aerosol therapy.
Both the prescribing physician and the respiratory therapist need to be aware that a good understanding of the anatomic and physiologic characteristics associated with this age group has to be matched to adequate selection of the proper device. When an optimal device has been selected, factors such as crying, lack of cooperation, and presence of leaks around the aerosol masks dramatically decrease lung deposition. Identification of the determinants of efficient aerosol delivery and the specific challenges of aerosol delivery to infants and young children can facilitate a systematic approach to optimize aerosol delivery to this population. All considerations in this chapter relate to inhaled aerosols used for the treatment of pulmonary disorders and not for systemic treatment. The data cited are based largely on traditional aerosol delivery devices. New, highly efficient delivery systems may lead to different results in the future. Terms used for different age ranges are given in Box 17-1.
Off-label use of drugs in neonatal and pediatric patients
The term off-label is used to refer to drugs lacking U.S. Food and Drug Administration (FDA)–approved dosing information for a specific age group or condition.5 Off-label prescribing is a commonly used and accepted medical practice. Although these drugs do have FDA approval, the approval is for a different use. Aspirin has been a common pain reliever for more than 100 years; in 1988, physicians began prescribing its use in preventing heart attacks. Use of aspirin for the prophylaxis of heart attack is considered an off-label use. It has been estimated that nearly half of all prescriptions today are written for off-label uses. Infants, children, and pregnant women are the most common groups in which off-label use medications are prescribed. More than 70% of the entire Physicians’ Desk Reference (PDR) entries have either no existing dosing information for pediatric patients or specific statements that the safety and efficacy in children have not been determined. It has been reported in the United Kingdom that when a “suitable alternative” did not exist, physicians prescribed unlicensed or off-label medicine in 90% of neonates in neonatal intensive care units, 70% of children in pediatric intensive care units, and two-thirds of children on general medical and surgical pediatric wards.
Table 17-1 lists inhaled aerosol drugs and leukotriene modifiers that currently have an approved age labeling for pediatric use at the time of this edition. Drugs that are not listed in Table 17-1 do not have labeling for pediatric use. In March 2004, the U.S. Patent and Trademark Office (USPTO) issued a patent covering AccuNeb, the only FDA-approved (in 2001) lower concentration, unit-dose albuterol sulfate for the treatment of asthma in children 2 to 12 years old.
TABLE 17-1
Pediatric Drug Labeling for Inhaled Aerosols and Leukotriene Modifiers*
DRUG NAME | FORMULATION | AGE LABELING (FDA APPROVED) |
β-adrenergic Agents | ||
Albuterol | MDI | ≥4 years: 2 inhalations q4-6h |
SVN | 2-12 years: 1.25-2.5 mg tid-qid† | |
Racemic epinephrine | SVN | ≥4 years: 0.5 ml of 2.25% solution in 3.0 ml diluent; q3-4h |
Metaproterenol | SVN | ≥6 years: 0.1-0.2 ml of 5% solution tid-qid |
Salmeterol | DPI | ≥4 years: 1 inhalation (50 μg) bid |
Formoterol | DPI | ≥5 years: 1 inhalation (12-24 μg) bid |
Levalbuterol | MDI | ≥4 years: 2 inhalations (90 μg) q 4-6h |
SVN | 6-11 years: 0.31 mg tid; maximum 0.63 mg tid | |
Corticosteroids | ||
Beclomethasone | MDI | ≥5 years: 40-80 g twice daily |
Budesonide | DPI | ≥6 years: 180-360 μg bid |
SVN | 12 months–8 years: 0.5 mg total daily dose given once or twice daily in divided doses; maximum 1 mg total daily given once or 0.5 mg twice daily | |
Mometasone furoate | DPI | 4-11 years: 110 μg daily |
Flunisolide | MDI | 6-11 years: 1 puff daily, no more than 2 puffs daily |
Fluticasone | DPI | ≥4 years: 50 μg twice daily up to 100 μg twice daily |
MDI | 4-11 years: 88 μg bid | |
Fluticasone propionate/salmeterol | DPI | ≥4 years: 100 g fluticasone/50 g salmeterol, 1 inhalation twice daily, about 12 hours apart |
Mucolytic Agent | ||
Dornase alfa | SVN | Safety and efficacy in children <5 years have not been studied; usual dose 2.5 mg once daily |
Nonsteroidal Antiasthma Agent | ||
Cromolyn sodium | SVN | ≥2 years: 20 mg tid-qid |
Inhaled Antiinfectives | ||
Ribavirin | SPAG | Infants and young children: a 20 mg/ml solution nebulized for 12-18 hours/day for 3-7 days |
Tobramycin | SVN | ≥6 years: 300 mg bid, alternate 28 days on, 28 days off |
Leukotriene Modifiers | ||
Aztreonam | SVN | 75 mg TID, alternate 28 days on, 28 days off |
Montelukast | PO | 12-23 months: one packet of 4-mg oral granules daily in the evening |
2-5 years: one 4-mg chewable tab daily in evening or one packet of 4-mg oral granules daily in the evening | ||
6-14 years: one 5-mg chewable tab daily in evening | ||
Zafirlukast | PO | 5-11 years: one 10-mg tablet bid |
Zanamivir | DPI | ≥ 5 years: 2 inhalations bid for 5 days |
*Additional detail on dosing for adults can be found in previous chapters. Manufacturers’ information and other sources on drug administration and dosing should be consulted before use. Drug labeling is current at the time of this edition.
†Most frequent administration is not recommended. Patients 6 to 12 years old with more severe asthma (baseline FEV1 <60% predicted), patients with weight greater than 40 kg, or patients 11 to 12 years old may achieve a better initial response with higher dose.
In 1994, the Center for Drug Evaluation and Research of the FDA issued guidelines encouraging pediatric testing of drugs.6 A subsequent FDA ruling mandated that drugs submitted for FDA approval after December 2000 be evaluated in children. Waivers can be given if the drug has no meaningful application in children or is likely to be unsafe or ineffective in pediatric patients.7 There is a substantial cost associated with additional studies to document safety and efficacy in pediatric patients. The creation of the “pediatric rule” has provided the pharmaceutical companies that carry out studies with pediatric patients an economic incentive by allowing extended time of marketing before generic formulations of the product are marketed.8 The FDA cannot, by law, regulate how a drug is used medically or “interfere with the practice of medicine.”5
Factors affecting neonatal and pediatric aerosol drug delivery
The same mechanisms of aerosol penetration and deposition in the lung that were outlined in Chapter 3 apply to aerosol therapy in neonatal and pediatric patients. The airway environment differs in neonatal and pediatric subjects compared with adults. Table 17-2 outlines functional and structural features of the infant lung that may affect aerosol delivery and deposition.
TABLE 17-2
Comparison of Neonatal and Adult Respiratory Parameters
PARAMETER | NEONATE | ADULT |
Tracheal diameter | ∼4 mm | ∼20 mm |
Tracheal length | 5-6 cm | 10-12 cm |
Tidal volume | 6 mL/kg | 6 mL/kg |
Respiratory rate | 30-40/min | 12-14/min |
Minute ventilation | 200-300 mL/kg/min | 6 L/min |
Dead space | 0.75 mL/lb | 1.0 mL/lb |
Inspiratory flow rate | ≤100 mL/sec | ∼500 mL/sec |
From Wilkins RL, Stoller JK, Kacmarek R, editors: Egan’s fundamentals of care, ed 9, St Louis, 2009, Mosby.
Although it may seem obvious that aerosol therapy in infants and young children is quite different from that of adults, much more research is required to confirm the differences in aerosol drug delivery. Some in vitro studies have suggested that the increasing upper airway geometry in adults may explain the higher amount of aerosol deposition reaching the lower airway compared with neonatal and pediatric patients.9 The nose may filter out 75% of the dose received by mouth breathing. A smaller fraction of the nominal dose of an aerosol reaches the lower airways with the child-size oropharynx compared with an adult-size oropharynx.10 The smaller diameter of neonatal and pediatric lower airways, added to the effects of bronchoconstriction, inflammation, secretions, and the possible presence of an endotracheal tube, dramatically decreases aerosolized drug deposition in the lungs.11 Some of the variables associated with the reduction in lung dose in infants and young children are discussed subsequently.
Effect of age on aerosol lung dose
Systemic side effects rather than local side effects caused by treatment in the lung have been the basis for aerosol dose adjustment for age, which is usually based on body weight. However, the need for age adjustment of aerosol doses based on body weight has been clinically debated.12 Available data suggest that the actual dose of an inhaled aerosol drug could be less than 1% in neonates and infants13,14 and around 2.5% in young children15 compared with the traditional 10% to 15% cited for adults.16,17 Although a smaller percentage of the aerosol deposits in the lungs, small patients may receive considerably higher drug per kilogram of body weight than adults. However, the lower deposition may provide a comparable safety and efficacy profile to that of adults.18
Anhoj and associates19 found that a pressurized metered dose inhaler (pMDI) of budesonide with a 250-ml steel NebuChamber (Astrazenica, London, UK) having less than 2 ml of dead space delivered the same approximate dose to a range of ages from 2 to 41 years. A face mask was used for children 2 to 3 years old, and a mouthpiece was used for older subjects. Although the resulting plasma concentration of drug was the same in children and adults (Figure 17-1), the inhaled dose and the dose reaching the lung were not the same. Because the holding chamber eliminated oropharyngeal and stomach loss and there is high first-pass metabolism of budesonide, plasma drug levels reflect dose in the lung. If the same dose went to the lungs in adults as in 2-year-olds, higher plasma levels would be observed in younger subjects who have smaller circulating blood volumes. This report suggests that inhaled dose need not be adjusted for age to reduce systemic levels and possible toxicity.19 When a fixed dose of 2.5 mg by nebulizer was compared with a dose of 0.1 mg/kg body weight in children 4 to 12 years old with acute asthma, there was no difference between the two dosing protocols in either clinical improvement measured by flows, oxygen saturations, and clinical score or cardiovascular and tremor side effects. The fixed dose of 2.5 mg by nebulizer was efficacious and safe.20
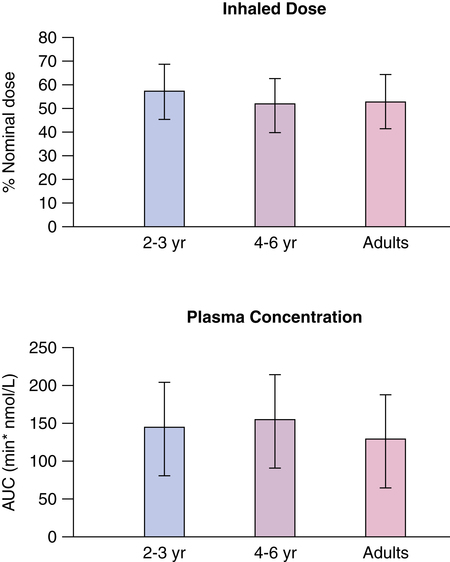
Effect of small tidal volumes, short respiratory cycles, and low flow rates
Effect on small volume nebulizer
If we assume a 6 L/min power gas, an adult with inspiratory flow of 500 mL/sec (30 L/min) and a tidal volume of 500 mL (500 mL/sec × 1 or 2 seconds) would completely inhale all of a nebulizer output. However, an infant with inspiratory flow of less than 100 mL/sec (less than 6 L/min) and a tidal volume less than 100 mL would not completely inhale all of the nebulizer output during the inspiratory phase (Figure 17-2).
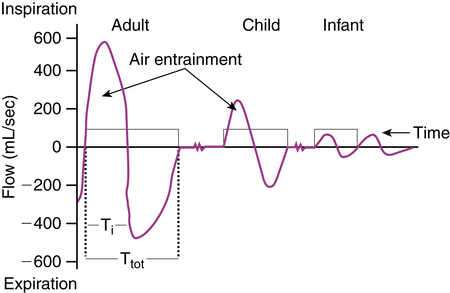
Collis and associates21 studied the fraction of nebulizer output inspired by infants 1 to 12 months old, children 3 to 16 years old, and adults 20 to 23 years old. Infants were sedated with chloral hydrate, and tidal breathing was recorded by face mask and pneumotachygraph. The fraction of nebulizer output inspired was lower in infants younger than age 6 months, then reached a plateau and remained constant in subjects older than 6 months. Wildhaber and colleagues22 also found that inhaled aerosol dose from an SVN attached to a mask increased with weight in infants 4 to 12 months old and was lower in smaller infants.23
Effect on reservoir dose
The same effect of low tidal volumes would theoretically reduce the amount of volume and drug mass inhaled from a reservoir chamber (Figure 17-3). The amount of reduction is proportional to the chamber volume for a given infant tidal volume. For a 50-mL tidal volume, approximately one-third of a 150-mL chamber and one-fifth (20%) of a 250-mL chamber would be inhaled. Even assuming no redistribution of aerosol in the chamber volume, gravitational settling would reduce available dose further within seconds of pMDI actuation into the chamber. The ideal volume for a spacer device is small enough to allow drug inhalation with few breaths for infants with low tidal volumes (less than 50 mL).
This theoretical prediction is supported by data from Everard and colleagues.23 Delivery (not lung deposition) of pMDI cromolyn sodium by reservoir and face mask with tidal volumes of 25 mL, 50 mL, and 150 mL was measured in vitro using various sizes of chambers. Smaller tidal volumes were associated with decreased inhaled drug mass. Higher aerosol concentrations in a smaller chamber enhanced drug delivery with tidal volumes less than 150 mL. Introduction of dead space between the chamber outlet and the filter collecting inspired drug reduced the dose deposited by 50% or more.24 The results are summarized in Table 17-3.
TABLE 17-3
Effect of Inspired Tidal Volumes on Mean (Range) Inhaled Aerosol Dose in Two Reservoir Devices
TIDAL VOLUME (mL) | |||
DEVICE | 25 | 50 | 150 |
AeroChamber (150 mL) | 0.33 mg (0.29-0.35 mg) | 1.15 mg (1.08-1.24 mg) | 1.41 mg (1.33-1.46 mg) |
Nebuhaler (750 mL) | 0.29 mg (0.26-0.32 mg) | 0.93 mg (0.91-0.97 mg) | 1.55 mg (1.48-1.61 mg) |
Data from Everard ML, Clark AR, Milner AD: Drug delivery from holding chambers with attached facemask, Arch Dis Child 67:580, 1992.
In contrast, an in vivo study by Wildhaber and associates22 that showed decreasing inhaled dose from a nebulizer in infants 6 to 7 kg also found that there was no effect of size (weight) on inhaled dose for two chamber devices used with a pMDI. The two reservoir devices were the Babyhaler (GlaxoSmithKline UK, Middlesex, UK) (350-mL chamber, 40 mL dead volume) and the NebuChamber (250-mL chamber, no dead volume). The plastic reservoir device (Babyhaler, 350 mL) had the electrostatic charge removed before testing. Turpeinen and colleagues24 compared the same reservoir devices and found that inhaled mass of budesonide did increase with increasing height, weight, and tidal volume with the Babyhaler but not with the NebuChamber. Such variation in study results indicates that testing conditions, type of drug, and choice of reservoir device can affect inhaled dose, although not necessarily the lung dose. Box 17-2 lists factors in reservoir devices that may affect the inhaled dose to neonates, infants, and small children.
Nebulized drug distribution
It might be obvious to the respiratory clinician that outcomes of aerosol therapy in young children are quite different from that in adults, and that much more research is required to confirm the differences in aerosol drug delivery. The most definitive data for answering the question of how much aerosol drug reaches the lungs of neonates and pediatric patients are actual measures of lung deposition. However, most radiolabeled substances used to estimate lung deposition may be harmful to children, limiting the scope of measurements.25 Some available data indicate that the lung dose of an aerosol drug does in fact decrease with age. Anhoj and associates19 found that blood levels of drug, reflecting the dose reaching the lungs, were constant in younger and older subjects, despite the smaller circulating volume of younger patients.
It has been hypothesized that the greater the infant’s distress, the lower the lung deposition and the higher the upper respiratory tract and gastrointestinal tract deposition. Evaluation of distribution of nebulized bronchodilators in wheezy infants has shown an average of 10% to 12% adherence to the patient’s face, 7.8% ± 4.9% deposition in the upper respiratory and gastrointestinal tracts, and 1.5% ± 0.7% deposited in the lungs (Figure 17-4).26,27
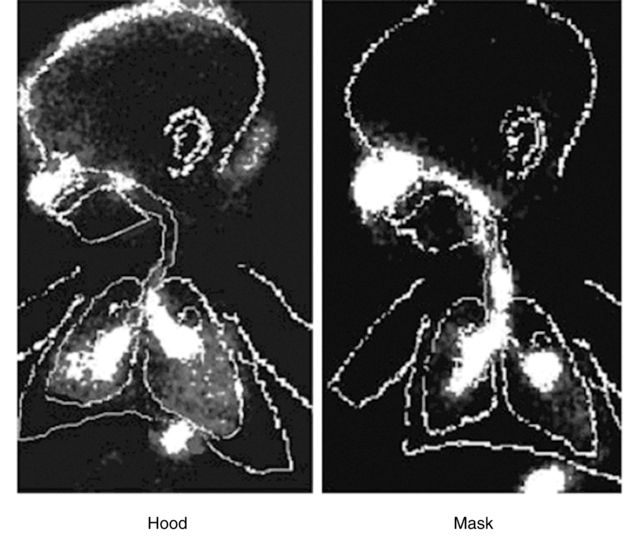
Figure 17-5 summarizes data on lung deposition with inhaled aerosols, compiled from four studies. All of the studies used a pMDI with a reservoir device and a face mask for delivery in subjects younger than 4 years. Values represent the percentage of total dose from the device. Fok and colleagues28 found that 0.67% (standard error of the mean [SEM] 0.17) of the total dose of albuterol reached the lungs of infants with a mean age of 86 days (range 25 to 187 days).
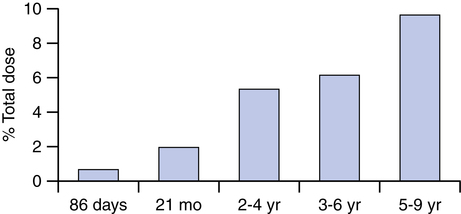
Tal and associates29 found 1.97% (standard deviation [SD] 1.4) as a lung dose of albuterol in patients with a mean age of 21 months (range 3 months to 5 years). Wildhaber and colleagues30 found 5.4% (SD 2.1) and 9.6% (SD 3.9) of albuterol reached the lungs of 2- to 4-year-olds and 5- to 9-year-olds. Agertoft and coworkers,31 using indirect measures of plasma levels, found 6.1% of a budesonide dose reached the lungs of 3- to 6-year-olds. Similar data have been reported for aerosol delivery with nebulizers (see Table 3-4).
If the amount of aerosolized albuterol reaching the lungs of infants and children in the study by Tal and colleagues29 is used, it can be shown that the self-limiting effect of younger ages on lung dose results in the same dose per amount of body weight in children as in adults (Table 17-4). The equivalence of dose per body weight illustrated in Table 17-4 and based on lung deposition data argues strongly against the need to adjust the nominal dose of an aerosol drug for age or body size with current aerosol delivery devices. Age and size have a self-limiting effect on lung dose, producing a natural titration of dose. This conclusion may need to be revised pending more efficient aerosol delivery devices.
TABLE 17-4
ADULT | CHILD | |
Weight | 80 kg | 10 kg |
Lung deposition | 20%* | 2% |
Nominal dose | 200 μg | 200 μg |
Dose to lung | 40 μg | 4 μg |
Per-kilogram dose | 0.5 μg/kg | 0.4 μg/kg |
*Percentage lung deposition measured by Tal and colleagues in two adult volunteers in the same study.
Data from Tal A, Golan H, Grauer N, et al: Deposition pattern of radiolabeled salbutamol inhaled from a metered-dose inhaler by means of a spacer with mask in young children with airway obstruction, J Pediatr 128:479, 1996.
Clinical response to aerosolized drugs in neonatal and pediatric patients
Commonly cited studies from the late 1970s concluded that response to inhaled bronchodilators was lacking in infants and children younger than 18 months of age.32,33 These studies found no change in respiratory resistance with phenylephrine, epinephrine, or salbutamol (albuterol) in infants and children 7 to 18 months of age using a forced oscillation technique. The authors of the studies speculated that there was either poor development of smooth muscle in children younger than 18 months of age or the bronchial obstruction was due to secretions and airway edema rather than bronchoconstriction.
A study by Turner and associates34 in 1993 found that the level of response to a bronchodilator increases significantly with increasing age in young asthmatics 3 to 9 years old. However, studies of ventilated and nonventilated preterm infants have shown dose-related changes in respiratory mechanics, including airway resistance, and compliance with aerosolized bronchodilators.35–43 Table 17-5 lists several studies and the outcomes measured that have documented clinical efficacy of aerosolized bronchodilators in infants.
TABLE 17-5
Results of Studies Examining Clinical Response to Aerosolized Albuterol in Infants
REFERENCE | AGE | DELIVERY | OUTCOME MEASURED |
Rotschild, 198940 | 1-4 weeks | SVN via ETT | Improved: respiratory resistance and dynamic compliance |
Sivakumar, 199941 | 12 ± 8 days | MDI/spacer via ETT versus SVN via ETT | Improved: respiratory resistance and dynamic compliance* |
Modl, 200542 | 8.5 ± 4.2 months | SVN | Improved: oxygen saturation, respiratory distress |
Hyvarinem, 200643 | 14.1 ± 6.1 months | Not specified | Improved: oxygen saturation, respiratory distress |
ETT, Endotracheal tube; MDI, metered dose inhaler; SVN, small volume nebulizer.
*MDI delivery resulted in significantly better dynamic compliance than SVN delivery, with no difference between delivery systems in respiratory resistance.
Selection of delivery devices
The current methods to deliver therapeutic aerosols can be classified in three categories: nebulizers (jet or ultrasonic); pMDIs that can be used with a press-and-breathe technique, as a breath actuated device, or in combination with a valved-holding chamber (VHC) or spacer; and dry powder inhalers (DPIs). Table 17-6 gives the age guidelines for use of current aerosol delivery devices in infants and children, based on the 2007 National Asthma Education and Prevention Program (NAEPP) guidelines.44 Either a jet nebulizer or an MDI can be used with suitable auxiliary devices attached. An evidence-based review by the American College of Chest Physicians determined that for most patients with asthma, SVNs, DPIs, and MDIs are equally effective in delivering short-acting β agonists.45,46 Nebulizer systems and the pMDI plus spacer are the most suitable aerosol delivery systems for young children because they only require tidal breathing to inhale the aerosol. Even in acute asthma attacks, delivery of bronchodilators by pMDI plus spacer are equally effective as nebulizers.4,47 Nebulizers could be considered as an alternative if the child appears very distressed during the administration of pMDI. Studies cited in Chapter 3 (see Table 3-4) showing equivalent clinical response between the MDI-reservoir systems, with or without a mask as needed by age, and jet nebulizers have been validated in young children.48
TABLE 17-6
Age Guidelines for Use of Current Aerosol Delivery Devices
DELIVERY DEVICE | AGE RECOMMENDED |
SVN with mask | ≤3 years |
SVN with mouthpiece | ≥3 years |
pMDI with spacer/VHC and mask | <4 years |
pMDI with spacer/VHC | ≥4 years |
DPI | ≥4 years |
pMDI | ≥5 years |
Breath-actuated MDI | ≥5 years |
Breath-actuated nebulizers | ≥5 years |
Data from National Asthma Education and Prevention Program: Expert Panel Report III: guidelines for the diagnosis and management of asthma, Bethesda, MD, 2007, National Institutes of Health.
Nebulizers
Jet nebulizers used to be the mainstay of aerosol therapy in infants and young children. However, they require a pressurized gas source, bulky equipment, a long treatment period, and additional preparation and cleaning time. A significant disadvantage of nebulizer therapy in children is the poor tolerance often exhibited because of noise of operation and the need for a tight-fitting mask.23 Although nebulizers may not routinely be used compared with pMDIs, nebulizers are sometimes preferred by numerous patients and parents. They are also the preferred delivery device for emerging therapies, such as aerosolized surfactants49,50 and antibiotics,51 currently under investigation.
Pressurized metered dose inhalers
pMDIs are the method of choice in infants and children younger than 5 years old, only when used in combination with an appropriate spacer or VHC.52 A pMDI should not be used without a spacer or VHC, even in children 8 years old or older, because most patients are unable to coordinate actuation of the pMDI with the breathing maneuver. An additional advantage of the pMDI-spacer combination is that it considerably reduces the oropharyngeal deposition. The pMDI delivery system has the advantage of smallness and portability and shorter treatment time, even with an increased number of actuations. However, drug delivery to the lungs using pMDIs can vary greatly, depending on the formulation used and the age of the child. Children 4 to 8 years old should be encouraged to use a mouthpiece along with the pMDI whenever possible, whereas a mask attached to the spacer is typically recommended in children younger than 4 years. Similar issues regarding leaks around the mask already mentioned for nebulizers apply to the pMDI-spacer-mask interface.53–59 The use of a pMDI-autohaler may be a sound alternative to the pMDI-spacer in children with asthma who are older than 8 years.
As mentioned in a previous chapter, immediately after the aerosol cloud is released from the pMDI, gravitational forces result in sedimentation of aerosol particles onto the spacer wall. This effect is more pronounced when a plastic spacer is used with electrostatic charge.60,61 A metal spacer could deliver to the mouth twice the amount of drug as a plastic spacer.62 However, although the electrostatic charge is totally eliminated with the use of a metal chamber, the simple act of coating a plastic spacer with household detergent minimizes the electrostatic charge and substantially increases lung deposition.63,64 The improvement in lung deposition associated with elimination of the electrostatic charge could be seriously compromised by a suboptimal face mask seal, as previously shown by Smaldone and colleagues.59
Dry powder inhalers
DPIs are usually not appropriate for children younger than 5 or 6 years of age because they are driven by peak inspiratory flows much greater than those required by pMDIs.45 Even if some young children are able to generate the 30 to 60 L/min range of inspiratory flow required by most inhaled corticosteroids to disperse adequate mass and particle size, it is questionable whether or not children can generate reproducible inspiratory flow patterns.
Compliance and cooperation during aerosol therapy
The most important factor to consider in the administration of aerosol therapy in infants and young children is their compliance. Two practical issues are important when dealing with young children, particularly infants: face mask and crying. The interaction between crying and the face mask is complex. It is very likely that crying and distress, even with the best-designed face mask, explain the poor seal that seriously affects the efficiency of aerosol administration in young children.65
Effects of crying on aerosol delivery
It is widely accepted that delivery devices that produce small particles are less flow dependent. This characteristic may be of critical importance for less cooperative children. Crying is associated with high inspiratory flows, high dose variability, and almost no lung deposition.66–69 A typical pediatric patient does not tolerate a mask applied to the face, and agitation and crying are frequently observed. The efficacy of aerosol therapy administered to a combative or crying toddler is known to be negligible because of the changes in respiratory patterns during nebulization. Amirav and associates27 have shown that the more distress infants were in during the treatment, the more aerosol was deposited extrathoracically. This finding should alert clinicians to the potential for increased systemic absorption and greater risk for side effects.
Although theoretically the poor aerosol delivery associated with crying could make aerosol administration during sleep an attractive alternative, the positive results of in vitro studies70 have not been found in vivo. Almost 70% of children wake up when aerosol is given during sleep and become distressed resulting in similar lung deposition found with crying. Because crying may be inevitable, the caregiver may have to find creative ways to prepare the child for the face mask at a time when the child does not need the treatment. This strategy would apply to maintenance therapy and not to the emergency setting or transport, where distracting and comforting the child may be the only available options to improve compliance. Dose variability seems to be independent of face mask design and more dependent on cooperation (Figure 17-6).71
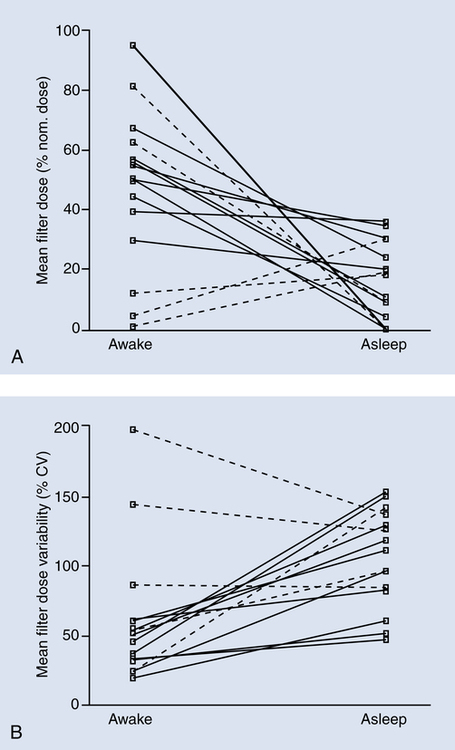
Mask-fit-to-face and mask design
Because children must be about 3 years old before they fully understand how to use a mouthpiece, younger children must use a mask. Efficient aerosol therapy requires the mask to fit to the face tightly and still be comfortable. The absence of a tight seal between the mask and the patient’s face results in a decrease in the amount of medication available for inhalation.59,62,72 Many parents are forced to hold the face mask near the face to avoid struggling with the child. This technique is well known as “blow-by.” However, it has been clearly documented in numerous studies that even a 0.5-cm gap between the mask and the face reduces the inhaled drug mass as a percentage of the nominal dose in a pediatric lung model of spontaneous breathing up to 50%.73,74
Development of more efficient, more acceptable, and friendlier face mask interfaces has been evaluated as an option to improve the inhaled drug mass. More recent reports comparing the standard aerosol pediatric mask with other proprietary masks have shown that the newly designed masks significantly increase the inhaled drug mass (Figure 17-7).75–77 It is recommended that children be switched from mask to mouthpiece as soon as they are old enough to inhale through the mouth voluntarily.15
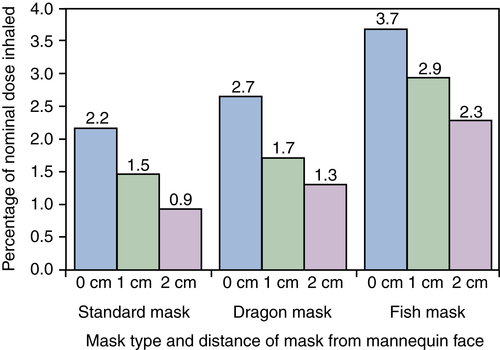
Nebulizer hood
Because it is very difficult to hold a mask snugly fitted to the face, particularly with infants, other alternatives have been explored. The nebulizer hood was designed as an attempt to develop more acceptable and patient-friendly interfaces. Janssens and coworkers,69 Amirav,78 and Kesser and colleagues79 all reported much greater aerosol delivery with a hood than the mask in vivo and in vitro. Hoods seem to reduce crying in infants significantly because nothing comes in contact with the infant’s face (Figure 17-8),
Aerosol administration in intubated neonatal and pediatric patients
Aerosol delivery seems to be less efficient in pediatric intubated patients than in spontaneously breathing patients.80 It is important to consider changes associated with the use of jet nebulizers in-line on pediatric and particularly neonatal ventilated patients because unexpected changes in volume and pressure may have deleterious effects. An externally powered nebulizer increases volume and pressure during volume-targeted ventilation, creates a bias flow in the ventilator circuit that may interfere with patient-triggered modes of ventilation,81 may result in increased airway pressure and unexpected positive end-expiratory pressure (PEEP), and may result in variable fractional inspired oxygen (Fio2) levels. Constant flow during expiration seriously limits the concentration of the inspired aerosol.10 Other nebulizer designs, such as vibrating mesh/membrane systems, do not require a gas source to operate and do not affect airway pressures during operation as usually seen with externally gas-powered nebulizers.82,83 If rapid respiratory rates are used, the ventilator duty cycle may be inadequate for the aerosol cloud to develop in the circuit and may seriously decrease aerosol deposition.84
The respiratory clinician should also be aware that aerosol delivery may be less effective with manual ventilation versus mechanical ventilation.58 Placement of the aerosol delivery device in an intubated infant requires the clinician’s attention. Although most studies show a slightly higher lung deposition with the pMDI when inserted between the Y-piece and the endotracheal tube, with an SVN placed in the inspiratory limb away from the Y-piece, lung deposition also averages 1% of the nominal dose.38 In pediatric patients, a pMDI and spacer with one-way valve is associated with a significantly larger amount of inhaled drug mass.85 No difference has been found between in vitro and in vivo studies.86 It has been suggested that the pMDI be actuated before inspiration to improve lung deposition.87
Summary
There is no difference in clinical effect for bronchodilators between pMDI and nebulizer administration. The pMDI dose may need to be adjusted upward for neonatal, pediatric, or adult use because the pMDI nominal dose is usually lower than the nebulizer dose. Neonates and very young pediatric patients show a clinical response to aerosolized bronchodilator administration. How to determine doses for aerosolized drugs delivered to neonatal and pediatric patients is not completely understood. Despite the differences in drug action between adults and children, the ability to control particle size by selecting the optimal aerosol delivery, placement of the aerosol device, and understanding patterns of aerosol generation are the key elements to improve efficiency of aerosol administration. Identifying the ability of the patient, rather than specific age, is also essential to selecting the most appropriate device in any population.88
It needs to be remembered that optimal aerosol administration requires matching patient characteristics with the aerosol delivery system. Infants and young children are a special group of patients with different anatomic, physiologic, and behavioral characteristics that dramatically affect outcomes of pharmacotherapy. Physicians, respiratory therapists, and nurses need to know how to select the right aerosol delivery device appropriately for children of different ages. Lack of coordination, inadequate inspiratory flows, limited cooperation, and crying are factors in infants and young children that greatly affect the efficiency of aerosol therapy in this age group. Because of the seriousness of treatment and possible errors in the inhalation technique, it is recommended that caregivers be reassessed at each patient encounter and that instructions be written in a manner the caregivers can understand. The development of in vitro models that better replicate not only the anatomy but also realistic breathing patterns may allow a better prediction of in vivo lung deposition of aerosols in neonatal and pediatric patients.89