Chapter 7 Mononuclear Phagocytes in Immune Defense
• Macrophages: The ‘big eaters’. Macrophages are endowed with a remarkable capacity to internalize material through phagocytosis.
• Macrophages differentiate from circulating blood monocytes and are widely distributed throughout the body. Macrophages belong to the family of mononuclear phagocytes, which also comprise monocytes, osteoclasts, and dendritic cells. These cells share a common hematopoietic precursor that cannot differentiate into neutrophils. Phenotypically distinct populations of macrophages are present in each organ.
• Macrophages are highly effective endocytic and phagocytic cells. Macrophages have a highly developed endocytic compartment that mediates the uptake of a wide range of stimuli and targets them for degradation in lysosomes.
• Macrophages sample their environment through opsonic and non-opsonic receptors. Macrophages express a wide range of receptors that act as sensors of the physiological status of organs, including the presence of infection.
• Clearance of apoptotic cells by macrophages produces anti-inflammatory signals. Macrophages produce IL-10 and TGF-β upon internalization of apoptotic cells.
• Macrophages coordinate the inflammatory response. Recognition of necrotic cells and microbial compounds by macrophages initiates inflammation leading to the recruitment of neutrophils. Monocyte recruitment to sites of inflammation is promoted by activated neutrophils and there is a collaborative effort between macrophages and neutrophils to eliminate the triggering insult. Macrophages are actively involved in the resolution of the inflammatory reaction.
• There are different pathways of macrophage activation. TH1 cytokines such as IFNγ enhance inflammation and anti-microbial activity. TH2 cytokines induce an alternate activation that promotes tissue repair. TGFβ, corticosteroids and IL-10 can induce an anti-inflammatory phenotype.
Macrophages: the ‘big eaters’
Macrophages differentiate from blood monocytes
Within tissues the mononuclear phagocytes undergo maturation, adapt to their local microenvironment, and differentiate into various cell types (Fig. 7.1). Distinctive populations of resident macrophages are found in most tissues of the body; they differ in their life span, morphology, and phenotype, for example the microglial cells in the brain appear quite unlike mononuclear phagocytes in other tissues (Fig. 7.2). Resident cells have usually ceased to proliferate, but may remain as relatively long-lived cells, with low turnover, unlike neutrophils.
Macrophage populations have distinctive phenotypes
Some of the key features of resident macrophage populations in tissues are shown in Figure 7.w1. The study of the heterogeneity and distribution of mononuclear phagocytes has been made possible through the use of antibodies against differentiation markers. These markers can be located at the plasma membrane or endosomal compartments. In the mouse the F4/80 antigen and macrosialin (CD68) have proved useful in defining the distribution of mature macrophages in many (but not all) tissues. Differentiation antigens such as sialoadhesin, a lectin-like receptor for sialylated glycoconjugates, are particularly strongly present on populations of macrophages in lymphoid organs that do not express F4/80 or CD68. Macrophages expressing sialoadhesin are exposed to the blood and lymph and are thought to be involved in antigen delivery. In humans, the CD68 antigen, the human homolog of macrosialin, is widely expressed in macrophages while the F4/80 homolog EMR2 labels subsets of macrophages. Anatomical differences between mouse and human spleen correlate with different distribution of macrophage differentiation markers. The mannose receptor is expressed by macrophages in the red pulp of mouse spleen but is absent from these cells in human spleen.
Some of the important stimuli that modulate macrophage phenotype are listed in Figure 7.w2 and some of their characteristic cell surface receptors in Figure 7.w3.
The tissue environment controls differentiation of resident macrophages
It is possible to reconstruct a constitutive migration pathway in which monocytes become endothelial-like and line vascular sinusoids, as in the liver (Kupffer cells, see Fig. 2.3), or penetrate between endothelial cells. They underlie endothelia or epithelia or enter the interstitial space or serosal cavities (Fig. 7.3). The molecular mechanisms of constitutive macrophage distribution and induced migration are beginning to be defined, and involve cellular adhesion molecules, cytokines, and growth factors, as well as chemokines and chemokine receptors.
Secondary lymphoid organs contain several distinct types of macrophages. These macrophages have been better characterized in the mouse (Fig. 7.4) and subsets involved in the clearance of apoptotic lymphocytes (tingible body macrophages) or presentation of naive antigens to B cells (subcapsular sinus macrophages) have been identified. Anatomical differences between human and mouse spleen, such as the absence of a well defined marginal sinus, correlates with phenotypical differences in splenic macrophages (see Fig. 7.4)
Macrophages can act as antigen-presenting cells
Macrophages, like dendritic cells, have all the machinery required for antigen processing and presentation of exogenous peptides and endogenous peptides on MHC class II and class I, respectively. Cross-presentation, a process by which peptides of exogenous origin are presented on MHC class I, also takes place in macrophages. While dendritic cells are uniquely suited for stimulating naive T cells in secondary lymphoid organs, macrophages present antigen in the periphery to activated (already primed) T cells. This interaction makes macrophages important effector cells during adaptive immunity (Fig. 7.5). The specialization of dendritic cells for antigen presentation correlates with a reduced degradative capacity that facilitates the generation of MHC-peptide complexes.
Macrophages act as sentinels within the tissues
Macrophages react to a wide range of environmental influences that help to fulfill their role as sentinels of the innate immune system (Fig. 7.6). The presence of cells within tissues with the potential to initiate inflammation through the release of cytokines and chemokines and to cause tissue damage through the production of reactive oxygen species requires control systems capable of downmodulating macrophage activation. One of these systems involves the molecule CD200L, which is an inhibitory receptor expressed by myeloid cells. CD200L inhibitory signaling is triggered through interaction with CD200 expressed by non-hematopoietic cells as well as macrophages. The CD200–CD200L interaction is important for the control of macrophage activation by other cells present in tissues.
Phagocytosis and endocytosis
Large particles are internalized by phagocytosis
Phagocytosis involves the uptake of particulate material (>0.5 μm) after recognition by opsonic or non-opsonic receptors (see Fig 7.6), its engulfment through the generation of pseudopodia and the formation of phagosomes. Phagosomes follow a similar maturation process to endosomes through the fusion with components of the early and late endocytic compartments so that maturing phagosomes sequentially adopt characteristics of early and late endosomes; this process culminates in the fusion of phagosomes to lysosomes to form phagolysosomes (Fig. 7.7). Phagosomal maturation is accompanied by acidification of the lumen (from 6.1–6.5 in early phagosomes to 4.5 in phagolysosomes), which controls membrane traffic and has a direct effect on microbial growth. Other microbicidal mechanisms associated with phagosome maturation are the generation of reactive oxygen and nitrogen species and the presence of antimicrobial proteins and peptides.
Macrophages sample their environment through opsonic and non-opsonic receptors
Opsonic receptors require antibody or complement to recognize the target
FcRs belong to the immunoglobulin superfamily (see Fig. 3.17). The best characterized FcR is CD64 (FcγRI), the high affinity receptor for IgG which signals through the common γ chain that contains an immunoreceptor tyrosine based activation motif (ITAM). The common γ chain is also used by some non-opsonic receptors that bind carbohydrates (see below) and it signals through the key kinase Syk. In humans other activating receptors for IgG are low affinity FcγRIIa (CD32) and FcγRIII (CD16), which require the recognition of immune complexes for inducing internalisation. IgG-opsonized material is readily internalized by macrophages and leads to the production of reactive oxygen species and cellular activation. The activating effect of ITAM-associated FcγRs is regulated by the presence of the inhibitory form of CD32 (FcγRIIb), which bears an ITIM.
The mechanism for ingestion of antibody-coated particles is distinct from that mediated by CR3 (Fig. 7.8). FcR-mediated uptake proceeds by a zipper-like process where sequential attachment between receptors and ligands guides pseudopod flow around the circumference of the particle. In contrast, CR3 contact sites are discontinuous for complement-coated particles which ‘sink’ into the macrophage cytoplasm. Small GTPases play distinct roles in actin cytoskeleton engagement by each receptor-mediated process.
The best characterized non-opsonic receptors are the Toll-like receptors (TLRs)
Non-opsonic receptors or pattern recognition receptors (PRRs) recognize unusual features characteristic of damaged, malfunctioning or infected tissues and their general characteristics are described in Chapter 6.
TLRs are membrane glycoproteins with an extracellular region responsible for ligand binding and a cytoplasmic domain responsible for triggering an intracellular signaling cascade. They can form hetero- or homo-dimers with each other or complex with other receptors in order to detect a wide range of microbial components. They are located at the cell surface or within endosomes. In humans there are 10 of these receptors and together they are able to recognize a wide range of microbes including Gram-positive bacteria and mycobacteria (see Fig. 6.20). For example, TLR4 detects Gram-negative bacteria because of its ability to recognize endotoxin. It then signals to the cell using similar systems to those mediated by IL-1 (Fig. 7.9). It can also activate the macrophage by a second pathway that is initiated by Trif, which leads to a secondary production of IFNβ and autocrine activation of additional macrophage genes.
CD14 is a GPI-linked membrane protein that facilitates the recognition of LPS by TLR4 so that it increases LPS sensitivity (see Fig. 7.9). Recently CD14 has also been shown to facilitate recognition of ligands by TLR2 and TLR3, which opens the possibility of CD14 acting as a multifunctional adaptor protein.
TLRs activate macrophages through several different pathways
The key adaptor molecules that mediate TLR-signalling are MyD88 (Fig. 7.w4) and Trif, both of which interact with the TIR cytoplasmic domain of TLRs. Most TLRs signal either through MyD88 or Trif, but TLR4 is unique in its ability to signal through both. The range of responses elicited by TLRs in macrophages is vast and includes the activation of the NFκB signaling pathway (see Fig. 7.w4) and MAP kinases, responsible for the production of proinflammatory cytokines and induction of microbicidal mechanisms. Signaling through the IL-1 and IL-18 receptors is also mediated by MyD88.
The Trif pathway induces IRF transcription factors that will lead to the production of type 1 interferons, which can then stimulate the macrophage to cause a second, delayed wave of gene activation (Fig. 7.w5).
Lectin and scavenger receptors are non-opsonic receptors that recognize carbohydrates and modified proteins directly
Scavenger receptors (SR) (Fig. 7.10) such as SR-A are involved in LPS clearance, and may serve to downregulate responses induced via the TLR4-CD14 pathway (see Fig. 7.9) and therefore limit the systemic release of TNFα and resultant septic shock. SR-A has also been involved in bacterial uptake. Another member of this family, CD36, collaborates with TLR2 in the recognition of S. aureus and M. tuberculosis.
Dectin-1 (Fig. 7.11), a lectin with a single lectin-like domain and an intracellular ITAM-like motif, is highly specific for the fungi-derived compound β-glucan (Fig. 7.12). Dectin-1-mediated effects are largely mediated by the kinase, Syk and Card 9 Dectin-1 mediates phagocytosis of β-glucan particles and synergizes with TLRs to boost immune responses. It also has a role in Th cell differentiation and β-glucan treated dendritic cells promote the development of TH17 cells. Humans deficient in dectin-1 are more susceptible to mucosal candidiasis.
The mannose receptor (MR) may play a unique role in tissue homeostasis as well as host defense (see Fig. 7.11). Endogenous ligands include lysosomal hydrolases and myeloperoxidase. The N terminal cysteine-rich domain of the MR is a distinct lectin for sulfated glycoconjugates highly expressed in secondary lymphoid organs. The cysteine-rich domain also contributes to the clearance of hormones such as lutropin. MR can internalize collagen, which is recognized through the fibronectin type II domain and recent evidence suggests that MR promotes TH2 responses, which correlates with its capacity to interact with multiple glycosylated allergens and secreted helminth products.
DC-SIGN, another mannose-binding C-type lectin (see Fig. 7.11), is expressed on some macrophages. It forms tetramers and lacks obvious signaling motifs at its cytoplasmic region. It has been implicated in interactions between APCs and T cells and in microbial recognition. DC-SIGN has been shown to modulate TLR signaling to promote transcription of various cytokine genes, particularly IL-10 and IL-8.
Other lectin receptors are langerin and dectin-2, which have mannose specificity, and Mincle, which recognizes ligands expressed by necrotic cells in addition to fungal pathogens. Dectin-2 and Mincle (see Fig. 7.11) signal through the common γ-chain that also mediates signaling by the FcR CD64.
Cytosolic receptors recognize intracellular pathogens
• the nucleotide binding and oligomerization domain (NOD)-like receptors (NLR) recognize, among others, bacterial compounds such as peptidoglycan; and
• the retinoic acid-inducible gene I (RIG-I)-like helicases (RLHs) recognize nucleic acids such as dsRNA which are produced during viral replication (Fig. 7.13).
Mechanism of action of NLRs
All NLR proteins have a similar domain structure:
• an N-terminal region that is involved in the recruitment of adaptor molecules that link ligand recognition to signal transduction;
• a central nucleotide binding and oligomerization domain (NACHT/NOD) that mediates oligomerization; and
• a C-terminal leucine-rich repeat (LRR) that mediates recognition of PAMPs or DAMPs.
Two key members of the NLR family are NOD-1 and NOD-2, which recognize fragments of peptidoglycan generated during the division of Gram-negative and Gram-positive bacteria (Fig. 7.w6). Detection of peptidoglycan through NOD receptors leads to the recruitment of the adaptor RICK, activation of NFkB and promotion of inflammation (see Fig. 7-w6).
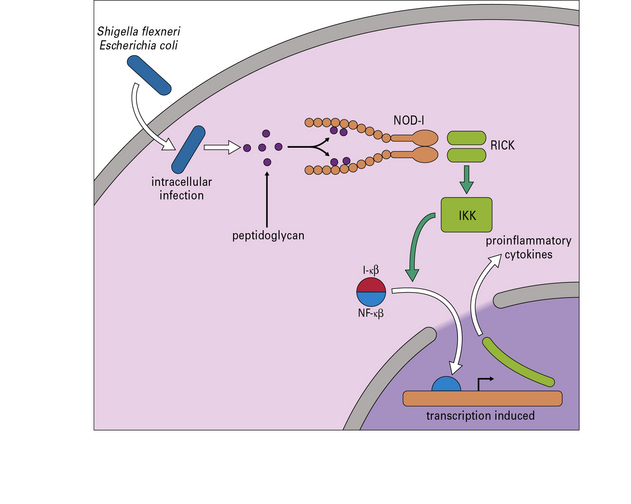
Fig. 7.w6 NOD-1 signal transduction
NOD-1 is an intracellular pattern recognition molecule. Peptidoglycan from, for example, Shigella flexneri and some strains of Escherichia coli binds to the protein. A domain (RICK) then activates IKK (see Fig. 7.w4) leading to the transcription of proteins dependent on NF-κB.
Other NLR proteins such as NLRP3, NLRP1 and IPAF, are components of multiprotein complexes called inflammasomes that lead to the activation of inflammatory caspases. Inflammatory caspases mediate the processing of pro-IL-1β and pro-IL-18 into their active counterparts and can lead to inflammation (see Fig. 7.13). Inflammatory caspases also lead to the induction of inflammatory cell death or pyroptosis in which the affected cell dies alerting the immune system of the presence of DAMPs by releasing its contents.
Functions of phagocytic cells
Clearance of apoptotic cells by macrophages produces anti-inflammatory signals
Q. Give an example in the immune system where macrophages phagocytose apoptotic cells
A. Thymocytes that fail the processes of positive and negative selection die by apoptosis and are phagocytosed by thymic macrophages (Chapter 2). Also B cells that die within lymphoid follicles are taken up by tingible body macrophages as described in Chapter 9.
Cellular and biochemical pathways resulting in apoptosis are conserved in evolution and apoptotic cells are rapidly and efficiently cleared by macrophages (Fig. 7.14), although they can also be engulfed by non-professional phagocytes. The appearance of phosphatidylserine (PS) in the outer leaflet of the plasma membrane is characteristic of apoptotic cells. PS recognition by PS-binding proteins stimulates the uptake of apoptotic cells and the production of anti-inflammatory mediators, especially TGFβ, which inhibit production of proinflammatory chemokines and cytokines.
Macrophages coordinate the inflammatory response
Recognition of necrotic cells and microbial compounds by macrophages initiates inflammation
In contrast to the recognition of apoptotic cells, uptake of microbial products and necrotic cells by resident macrophages promotes cellular activation leading to the production of secreted molecules (Fig. 7.15). Recognition of PAMPS and molecules from damaged cells through PRRs leads to the activation of the NFκB pathway (see Fig. 7.w4) and the production of cytokines and chemokines. The activation of cytosolic phospholipase A2 causes the release of arachidonic acid, the precursor of prostaglandins and leukotrienes, through the actions of the cycloxygenase and lipoxygenase pathways, respectively. Proinflammatory prostaglandins control blood flow and vascular dilation and permeability at sites of inflammation. Trafficking of lymphocytes and neutrophils into tissues is induced by leukotriene-B4 (see Figs. 6.17 & 6.18).
Resident macrophages recruit neutrophils to inflammatory sites
Neutrophils are the first cells recruited to the site of inflammation and play a key role in elimination of the inflammatory insult (Fig. 7.16). Appropriate neutrophil recruitment is essential for successful resolution of the inflammatory response as deficiencies in the clearance of the triggering stimuli will result in chronic inflammation and tissue dysfunction (Fig. 7.17). Although macrophages and neutrophils share the capacity to mediate phagocytosis and intracellular killing, there are key differences between them:
• neutrophils are short lived, store a wide range of antimicrobial polypeptides in intracellular granules and readily produce oxygen radicals;
• resident macrophages are long lived, less microbicidal and cytotoxic, and display a higher degree of specificity which makes them better suited as sentinels of the immune system.
Activated tissue macrophages produce chemokines CXCL5 and CXCL8 that promote neutrophil recruitment (see Fig. 7.16) and neutrophil extravasation is also promoted through proteolytic cleavage of chemokines by metalloproteases (MMP8 and MMP9), which enhance their chemotactic activity. The primary role of neutrophils in a wound is to eliminate invading pathogens. To aid this goal recruited neutrophils that fail to encounter bacteria in a short period of time will soon release their microbicidal compounds leading to the liquefaction of tissue and the formation of pus. Tissue destruction facilitates bacterial clearance by eliminating collagen fibrils that limit cellular movement.
Macrophages and neutrophils have complementary microbicidal actions
• secretory vesicles loaded with membrane-bound receptors are mobilized to the plasma membrane;
• secondary and tertiary granules containing lactoferrin, lipocalin, lysozyme and LL37 are released. These granules also contain matrix metalloproteases MMP-8, -9 and -25, that digest extracellular matrix and facilitate tissue destruction;
• primary or azurophilic granules containing defensins (see below) and myeloperoxidase are released –myeloperoxidase converts H2O2 to hypochlorous acid which reacts with amines to produce anti-bacterial chloramines (Fig. 7.18).
• are small peptides (30–33 amino acids) found in some macrophages of many species and specifically in human neutrophils, where they comprise up to 50% of the granule proteins;
• form ion-permeable channels in lipid bilayers and probably act before acidification of the phagolysosome;
• are able to kill a range of pathogens, including bacteria (S. aureus, Pseudomonas aeruginosa, E. coli), fungi (Cryptococcus neoformans), and enveloped viruses (Herpes simplex).
Phagocytes kill pathogens with reactive oxygen and nitrogen intermediates
Macrophages and neutrophils can also kill pathogens by secreting highly toxic reactive oxygen intermediates (ROIs) and reactive nitrogen intermediates (RNIs) into the phagosome (see Fig. 7.18). The NOX2 NADPH oxidase located at the phagosomal membrane generates ROIs and this property is most prominent in neutrophils. This oxidase transfers electrons from cytosolic NADPH to molecular oxygen releasing O−2 into the lumen. ROIs can interact with macromolecules (for instance through sulfur groups) rendering them inactive. Patients with chronic granulomatous disease lacking essential oxidase components suffer from repeated bacterial infections.
Macrophages can also be activated by IFNγ to express high levels of inducible nitric oxide synthase (i-NOS, NOS2), which catalyzes the production of nitric oxide (NO) from arginine (Fig. 7.19). ROIs and RNIs can interact to produce peroxynitrites and these reactive species all act within the phagosome to cause toxic effects on intraphagosomal pathogens; they interact with thiols, metal centers and tyrosines, damaging nucleic acids and converting lipids through oxidative damage. In this way bacterial metabolism and replication are impaired.
Q. Describe four roles of IFNγ in the development of the immune response, each modulating a different group of cells
A. IFNγ induces MHC molecules and co-stimulatory molecules on APCs, and induces adhesion molecules and chemokines on endothelium. It also activates macrophage microbicidal activity, modulates class switching by B cells (see Chapter 9), and regulates the balance between TH1 and TH2 populations of T cells. It has some capacity to induce production of anti-viral proteins.
Functions of secreted molecules
Lysozyme acts directly on the bacterial cell-wall proteoglycans, present especially in the exposed cell wall of Gram-positive bacteria (Fig.7.w7). The cell walls of Gram-negative bacteria may also become exposed to lysozyme if they have been damaged by complement membrane attack complexes. Lysozyme is constitutively produced by macrophages.
Cytotoxic products and powerful neutral proteinases such as elastase, collagenase, and urokinase (generating plasmin) (Fig.7.w8) are able to induce tissue injury and contribute to destructive chronic inflammation in joints and lung. Monocyte-derived procoagulant/tissue factor can also induce vascular occlusion and tissue damage.
Resolution of inflammation by macrophages is an active process
Complete removal of the inflammatory trigger initiates the resolution of inflammation, in which neutrophil infiltration stops and apoptotic neutrophils are phagocytozed. A key event in this process is ‘lipid mediator class-switch’ in which prostaglandin and leukotriene synthesis is replaced by the synthesis of lipoxins, resolvins and protectins (see Fig. 7.16). Intriguingly, the signaling pathways leading to the proinflammatory prostaglandins E2 and D2, synthesized early during inflammation, also lead to the transcription of the enzyme responsible for lipoxin synthesis. Lipoxin synthesis by neutrophils occurs through their interaction with platelets and epithelial cells that provide metabolic precursors through a process called transcellular biosynthesis. In the case of macrophages, lipoxin synthesis is triggered by uptake of apoptotic cells. Lipoxin A4 reduces neutrophil activity, increases the migration of monocytes, favors uptake of apoptotic neutrophils and inhibits the synthesis of CXCL8. Resolvin E1 and protectin D1 increase the expression of CXCR5 on the surface of apoptotic neutrophils which facilitates clearance of CXCL3 and CXCL5 (neutrophil chemoattractants) from the inflammatory site. Apoptotic neutrophils produce ‘find me’ signals (e.g. lysophosphatidylcholine) that attract macrophages. Uptake of these neutrophils by macrophages inhibits production of IL-23, a cytokine involved in promoting granulopoiesis. As described above, recognition of apoptotic cells by macrophages also leads to the production of the anti-inflammatory cytokines IL-10 and TGFβ that together with factors such as vascular endothelial growth factor promote tissue repair.
Different pathways of macrophage activation
• M1 activation refers to macrophages with enhanced microbicidal activity that can be generated in culture by treating with selected TLR ligands or pathogens in the presence (classical activation) or absence (innate activation) of IFNγ;
• M2 activation generally refers to macrophages exposed to IL-4 or IL-13 although within the spectrum of M2 activation, macrophages with a regulatory phenotype can also be obtained in response to immunocomplexes and TLR engagement (Fig. 7.20).
Major attempts are being made to correlate the phenotypes observed in vitro with patterns of differentiation in vivo through the analysis of signature markers, some of them having been identified during parasite infection in mice (Fig. 7.21). Classical activation appears to be consistent with the role of macrophages as effector cells during the course of cell-mediated immune responses where IFNγ produced by TH1 T cells will enable the elimination of intracellular pathogens by macrophages (see above). Additionally M1 macrophages promote TH1 responses by producing IL-12, and secreting CXCL9 and CXCL10 which selectively recruit TH1 T cells.
Macrophages treated with IL-4 and IL-13 have a more developed endocytic compartment, produce reduced levels of proinflammatory cytokines and IL-12, increased levels of IL-10 and the decoy receptor IL1RA and can recruit Tregs and TH2 cells, eosinophils and basophils through the production of CCL17, CCL22 and CCL24, thereby amplifying TH2-type responses. M2 macrophages are less efficient in producing ROIs and RNIs. In these cells arginine is processed into ornithine and polyamines through activation of arginase. Arginase activity has been proposed as a way of controlling T cell activation (see Fig. 7.19).
Critical thinking: The role of macrophages in toxic shock syndrome (see p. 435 for explanations)
1. What cytokines would you measure in the 2-hour serum sample?
2. What clinical signs would be indicative of incipient septic shock?
3. What mechanisms contribute to septic shock?
4. What outcome would you expect if the following knockout mouse strains were used instead of wild-type controls: CD14, scavenger receptor class A (SR-A), IFNγ?
The distinction between inflammatory responses and housekeeping of apoptotic cells
8. How would you investigate the macrophage surface receptors responsible for apoptotic cell uptake?
9. Suggest a possible mechanism by which apoptotic cell uptake promotes T. cruzi survival.
10. How would you investigate this experimental model?
11. What is the possible in vivo and clinical significance of this observation?
The role of macrophages in TH1 and TH2 responses
12. Interpret the significance and possible functional relevance of these results in relation to concepts of TH1/TH2 differentiation.
13. What further work could be done to investigate the possibility that macrophage activation could by analogy be classified as M1/M2?
14. How would you investigate the role of macrophages and dendritic cells as possible inducers of CD4+ T cell subset differentiation?
Allen J., Wynn T. Evolution of TH2 immunity: a rapid repair response to tissue destructive pathogens. PLOS Pathogens. 2011;7:e1002003.
Auffray C., Sieweke M.H., Geissmann F. Blood monocytes: development, heterogeneity, and relationship with dendritic cells. Annu Rev Immunol. 2009;27:669–692.
Bergsbaken T., Fink S.L., Cookson B.T. Pyroptosis: host cell death and inflammation. Nat Rev Microbiol. 2009;7:99–109.
Biswas S.K., Lopez-Collazo E. Endotoxin tolerance: new mechanisms, molecules and clinical significance. Trends Immunol. 2009;30:475–487.
Biswas S.K., Mantovani A. Macrophage plasticity and interaction with lymphocyte subsets: cancer as a paradigm. Nat Immunol. 2010;11:889–896.
Burdette D.L, Monroe K.M, Sotelo-Troha K., et al. STING is a direct innate immune sensor of cyclic di-GMP. Nature. 2011;478:515–518.
Elliott M.R., Ravichandran K.S. Clearance of apoptotic cells: implications in health and disease. J Cell Biol. 2010;189:1059–1070.
Flannagan R.S., Cosio G., Grinstein S. Antimicrobial mechanisms of phagocytes and bacterial evasion strategies. Nat Rev Microbiol. 2009;7:355–366.
Geijtenbeek T.B., Gringhuis S.I. Signalling through C-type lectin receptors: shaping immune responses. Nat Rev Immunol. 2009;9:465–479.
Geissmann F., Gordon S., Hume D.A., et al. Unravelling mononuclear phagocyte heterogeneity. Nat Rev Immunol. 2010;10:453–460.
Geissmann F., Manz M.G., Jung S., et al. Development of monocytes, macrophages, and dendritic cells. Science. 2010;327:656–661.
Gordon S., Martinez F.O. Alternative activation of macrophages: mechanism and functions. Immunity. 2010;32:593–604.
Hamilton J.A. Colony-stimulating factors in inflammation and autoimmunity. Nat Rev Immunol. 2008;8:533–544.
Hampton M.B., Kettle A.J., Winterbourn C.C. Inside the neutrophil phagosome: oxidants, myeloperoxidase, and bacterial killing. Blood. 1998;92:3007–3017.
Hornung V., Latz E. Intracellular DNA recognition. Nat Rev Immunol. 2010;10:123–130.
Kerrigan A., Brown G.D. Syk-coupled C-type lectins in immunity. Trends Immunol. 2011;32:151–156.
Lawrence T., Gilroy D.W. Chronic inflammation: a failure of resolution? Int J Exp Pathol. 2007;88:85–94.
Levine B., Mizushima N., Virgin H.W. Autophagy in immunity and inflammation. Nature. 2011;469:323–335.
Martinez F.O., Helming L., Gordon S. Alternative activation of macrophages: an immunologic functional perspective. Annu Rev Immunol. 2009;27:451–483.
Mosser D.M., Edwards J.P. Exploring the full spectrum of macrophage activation. Nat Rev Immunol. 2008;8:958–969.
Nathan C. Neutrophils and immunity: challenges and opportunities. Nat Rev Immunol. 2006;6:173–182.
Nathan C. Ding A. Nonresolving inflammation. Cell. 2010;140:871–882.
Nguyen K.D., Qiu Y., Cui X., et al. Alternatively activated macrophages produce catecholamines to sustain adaptive thermogenesis. Nature. 2011;480:104–109.
Olefsky J.M., Glass C.K. Macrophages, inflammation, and insulin resistance. Annu Rev Physiol. 2010;72:219–246.
O’Neill L.A., Bowie A.G. The family of five: TIR-domain-containing adaptors in Toll-like receptor signalling. Nat Rev Immunol. 2007;7:353–364.
O’Neill L.A., Bowie A.G. Sensing and signaling in antiviral innate immunity. Curr Biol. 2010;20:R328–R333.
Peiser L., Mukhopadhyay S., Gordon S. Scavenger receptors in innate immunity. Curr Opin Immunol. 2002;14:123–128.
Ray K., Marteyn B., Sansonetti P.J., Tang C.M. Life on the inside: the intracellular lifestyle of cytosolic bacteria. Nat Rev Microbiol. 2009;7:333–340.
Robinson M.J., Sancho D., Slack E.C., et al. Myeloid C-type lectins in innate immunity. Nat Immunol. 2006;7:1258–1265.
Schroder K. Tschopp J. The inflammasomes. Cell. 2010;140:821–832.
Serhan C.N., Savill J. Resolution of inflammation: the beginning programs the end. Nat Immunol. 2005;6:1191–1197.
Serhan C.N., Yacoubian S., Yang R. Anti-inflammatory and proresolving lipid mediators. Annu Rev Pathol. 2008;3:279–312.
Soehnlein O., Lindbom L. Phagocyte partnership during the onset and resolution of inflammation. Nat Rev Immunol. 2010;10:427–439.
Soehnlein O., Lindbom L., Weber C. Mechanisms underlying neutrophil-mediated monocyte recruitment. Blood. 2009;114:4613–4623.
Taylor P.R., Gordon S., Martinez-Pomares L. The mannose receptor: linking homeostasis and immunity through sugar recognition. Trends Immunol. 2005;26:104–110.
Taylor P.R., Martinez-Pomares L., Stacey M., et al. Macrophage receptors and immune recognition. Annu Rev Immunol. 2005;23:901–944.
Vieira O.V., Botelho R.J., Grinstein S. Phagosome maturation: aging gracefully. Biochem J. 2002;366:689–704.
Woodward J.J., Iavarone A.T., Portnoy D.A. c-di-AMP secreted by intracellular Listeria monocytogenes activates a host type I interferon response. Science. 2010;328:1703–1705.
Emara M., Royer P.J., Abbas Z., et al. Recognition of the major cat allergen Fel d 1 through the cysteine-rich domain of the mannose receptor determines its allergenicity. J Biol Chem. 2011;286:13033–13040.
Ferwerda B., Ferwerda G., Plantinga T.S., et al. Human dectin-1 deficiency and mucocutaneous fungal infections. N Engl J Med. 2009;361:1760–1767.
Heinsbroek S.E., Taylor P.R., Martinez F.O., et al. Stage-specific sampling by pattern recognition receptors during Candida albicans phagocytosis. PLoS Pathog. 2008;4:e1000218.
Krausgruber T., Blazek K., Smallie T., et al. IRF5 promotes inflammatory macrophage polarization and TH1-TH17 responses. Nat Immunol. 2011;12:231–238.
LeibundGut-Landmann S., Gross O., et al. Syk- and CARD9-dependent coupling of innate immunity to the induction of T helper cells that produce interleukin 17. Nat Immunol. 2007;8:630–638.
Liu Y., Stewart K.N., Bishop E., et al. Unique expression of suppressor of cytokine signaling 3 is essential for classical macrophage activation in rodents in vitro and in vivo. J Immunol. 2008;180:6270–6278.
Pesce J.T., Ramalingam T.R., Mentink-Kane M.M., et al. Arginase-1-expressing macrophages suppress Th2 cytokine-driven inflammation and fibrosis. PLoS Pathog. 2009;5:e1000371.
Royer P.J., Emara M., Yang C., et al. The mannose receptor mediates the uptake of diverse native allergens by dendritic cells and determines allergen-induced T cell polarization through modulation of IDO activity. J Immunol. 2010;185:1522–1531.
Satoh T., Takeuchi O., Vandenbon A., et al. The Jmjd3-Irf4 axis regulates M2 macrophage polarization and host responses against helminth infection. Nat Immunol. 2010;11:936–944.
Smith A.M., Rahman F.Z., Hayee B., et al. Disordered macrophage cytokine secretion underlies impaired acute inflammation and bacterial clearance in Crohn’s disease. J Exp Med. 2009;206:1883–1897.