CHAPTER 8 Molecular Biology and Genetics of Meningiomas
INTRODUCTION
Meningiomas are slow-growing tumors and are generally diagnosed in middle aged or older individuals. They account for up to 37% of all symptomatic primary brain tumors. Small and slowly growing meningiomas may be identified only incidentally during neuroimaging for other reasons1 or at autopsy.2 The prevalence of meningioma is difficult to assess and is likely to be higher than might be concluded from the numbers diagnosed due to symptoms. In a Swedish autopsy study encompassing almost 12,000 cases, the incidence of incidental meningiomas was 1.44%.2 There are reports that the incidence has increased in the last 40 years, but this period coincides with the more widespread use of new imaging technologies, making definite conclusions difficult. Meningiomas are more common in females, with a male:female ratio of approximately 1:2 for all meningiomas at all sites, while in the spine the ratio is 1:10. Multiple tumors are frequently associated with hereditary tumor syndromes such as neurofibromatosis type 2 (NF2), as are the rare childhood meningiomas, which are more common in males. The more aggressive and malignant forms are also more common in males. These differences indicate that genetic or hormonal factors, or both, affect the frequency of the disease.
The tumors are generally fixed to the dura and can be located anywhere in the cranial cavity or spinal canal. Many histologic types of meningioma are described, with at least nine being recognized by the current World Health Organization (WHO) classification of tumors of the central nervous system (CNS) as grade I tumors (meningothelial, fibrous, transitional, psammomatous, angiomatous, microcystic, secretory, lymphoplasmacyte-rich, and metaplastic as well as intermediate and more difficult to classify types). The grade II meningiomas include chordoid, clear cell, and atypical meningiomas and the more aggressive grade III meningeal tumors include papillary, rhabdoid, and anaplastic meningiomas. Both the atypical and the anaplastic meningiomas can belong to any of the aforementioned histologic types but must also fulfill additional criteria such as increased cellularity and mitotic index, the presence of necrosis, and a malignant cytology. Meningiomas rarely metastasize and generally grow by expansion and local invasion. The pathology of meningiomas is described in detail in Chapter 5.
FAMILIAL TUMOR SYNDROMES CLINICALLY ASSOCIATED WITH MENINGIOMA
It has long been known that meningiomas are, together with bilateral acoustic schwannomas, a key feature of NF2 syndrome. However, there are additional families that show an increased susceptibility to meningiomas, but without any other evidence of the NF2 syndrome.3 More recent molecular genetic studies of some of these families has shown no linkage of the disease state to the NF2 locus,4 indicating there are one or more independent syndromes predisposing to specifically meningioma development. The type of meningioma that develops in these families is frequently meningothelial, which, as described later, is particularly interesting as sporadic meningothelial meningiomas have a low incidence of NF2 mutations compared to the other more common meningioma types.3,5 Other inherited tumor syndromes in which an increase in the incidence of meningiomas has been reported include Werner, Cowden, Gorlin, and even multiple endocrine neoplasia (MEN) type 1.3,6,7 Meningiomas have also been reported in patients suffering from both other tumor syndromes and nontumor syndromes such as Li–Fraumeni, Turner, and Hunter–MacDonald.8,9
CYTOGENETICS
Meningiomas were one of the earliest human solid tumors to be studied with regard to their cytogenetics. Studies conducted in the 1960s before the development of chromosomal banding techniques10 reported one acrocentric chromosome to be frequently lost.11,12 Use of banding techniques showed the lost chromosome to be chromosome 22.13,14 This finding was subsequently confirmed by multiple studies. As cytogenetic analysis requires short-term culture with attendant selection, it remained unknown whether the findings were representative of the majority of the meningioma tumor cells in a patient. In 1987, molecular genetic methods studying DNA from homogenized tumor tissue using polymorphic DNA markers provided evidence that loss of chromosome 22 occurred frequently in the vast majority, if not all of the cells in patients’ tumors.15–18
MOLECULAR GENETICS
Identification of the NF2 Gene as a Tumor Suppressor Gene in Meningiomas
With the establishment of molecular methods, it was rapidly determined that approximately 50% of WHO grade I meningiomas have monosomy chromosome 22, with a further 10% showing interstitial allelic losses that involve band 22q12.15,17–19 The incidence of loss of alleles on chromosome 22 was observed to vary between meningioma types.20 A recent study of 126 sporadic tumors using array-CGH reported losses to be most common in fibroblastic meningiomas (>80%) followed by transitional meningiomas (>60%), but relatively infrequent in meningothelial meningiomas (<40%).21 The figures for the other and less common variants are less well established.
In parallel with such studies, the hunt for the NF2 gene showed linkage to the same 22q12 region. In 1993 the NF2 gene was identified22,23 and with the clinically well established association of meningiomas with the NF2 syndrome, mutations of the retained NF2 allele were rapidly shown in the majority of meningiomas with loss of one copy of chromosome 22. Thus NF2 was identified as a tumor suppressor gene in meningiomas.20,24,25 In meningiomas, the loss of this tumor suppressor protein most commonly occurs by loss of one NF2 gene allele and mutation of the retained allele. Loss of the protein products of a tumor suppressor gene may occur due to inhibition of expression by promotor-methylation of a wild-type gene copy. However, in meningiomas there is little evidence to support the methylation of the NF2 promoter as an alternative mechanism.21
As there is a close relationship between the loss of alleles on chromosome 22 (encompassing one NF2 gene) and the frequency of mutation of the retained NF2 gene, the incidence of loss of both wild-type NF2 genes varies in the different subtypes of meningioma in a similar manner. Fibroblastic meningiomas have the highest incidence of NF2 mutations (>50%) while mutations in the meningothelial variant are as low as 18%.21 Loss of both wild type NF2 genes has also been shown to occur in the uncommon childhood meningiomas.26 In line with these genetic data, immunocytochemistry for a protein product of the NF2 gene—most commonly known as merlin—has been found frequently in meningothelial tumors but is commonly absent in many of the other variants.27–30
Meningiomas are not the only tumors with loss of both copies of wild-type NF2. As expected this occurs in the majority of schwannomas31,32 as well in approximately 60% of spinal ependymomas and malignant mesotheliomas.33,34 Other tumors in which mutations have been found include malignant melanomas, and thyroid carcinomas. The status of the NF2 gene in other tumor types remains poorly documented.
Thus, at least 30% of all meningiomas and the vast majority of meningothelial meningiomas do not lose alleles on 22q, nor do they have mutations or aberrant methylation of the NF2 gene. The genes involved in the oncogenesis of these meningiomas remain uncertain. As NF2 codes for a member of the 4.1 superfamily of genes other members have been investigated to some degree. Loss of heterozygosity studies have indicated losses of one copy of the DAL1 gene (also known as EPB41L3; 4.1B) at 18p11.32 and its expression in meningiomas.35,36 It is not clear how frequently this occurs, with widely differing values reported in different studies, while immunocytochemically assessed loss of expression of the protein product, has been documented in up to 76% of all meningiomas.30,35,37 However, no or very few definite mutations of retained alleles of DAL1 have been found;38 nor has any evidence for promoter methylation been reported. The EPB41 gene (1p36; coding the 4.1R protein) has also been studied and no convincing evidence for its involvement has been presented.39 No copy number abnormalities have been noted in the region of the other family members ezrin and radixin and screening for mutations in moesin has proved negative.37
Interstitial deletions of 22q not involving the NF2 locus have been interpreted to indicate another meningioma tumor suppressor gene located on this chromosomal arm. Several candidate genes have been investigated including the SMARCB1/INI1 gene (no wild-type in atypical teratoid / rhaboid tumors) and the MN1 gene, and single cases with abnormalities have been identified.40,41 A homozygous deletion at 22q12 in a sporadic meningioma resulted in the identification of a β-adaptin gene (AP1B1 or BAM22) in the deleted region. Loss of expression was found in 9/70 meningiomas, 7 of which had no detectable abnormalities of the NF2 gene. However, no further indications that this might constitute another tumor suppressor gene for meningioma on 22q have been presented.42,43
The NF2 Gene and Its Transcripts and Protein Products
The NF2 tumor suppressor gene at 22q12.2 is composed of 17 exons and covers a genomic region of 95 kb (Fig. 8-1). It encodes transcripts with at least eight well-documented alternatively spliced open reading frames. There are two dominant splice variants. The first and longest open reading frame found in transcripts is 1785 bases with the splicing out of exon 16 and encodes a protein of 595 residues, merlin (also referred to as isoform 1). The second has a slightly shorter open reading frame and splices in exon 16, altering the 3′ sequence, and encodes a protein of 590 residues (see Fig. 8-1). The other commonly expressed splice variants lack exon 2, exon 3, or both, with the remaining isoforms expressed at low levels.44 Most investigations have focused on isoform 1 and while there have been some studies of isoform 2, almost nothing is known of the function of the proteins the other splice variants code for. Transcripts of approximately 6.1 kb and 2.7 kb are expressed ubiquitously but in a ratio that is tissue specific.44 Some tissues also expressed a transcript of approximately 3.9 kb. There is also some evidence that initiation of transcription can occur at several start sites, affecting the length of the 5′ untranslated sequences while the 3′ untranslated sequences in the large transcripts consist of thousands of bases.44 The full-length splice variants encode a deduced protein with approximately 47% identity to several members of the 4.1/ERM family of proteins believed to be involved in linking cytoskeletal components to cell membrane proteins including moesin, ezrin, and radixin: thus the name merlin.23
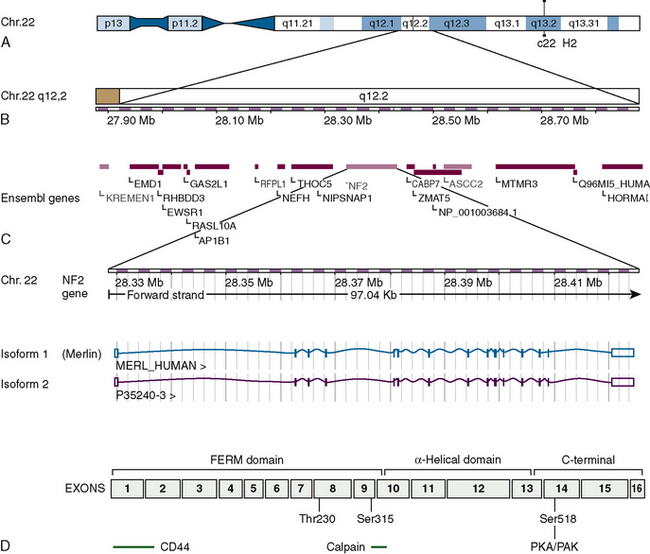
FIGURE 8-1 Overview of the location, the genetic makeup, and the two dominant splice variants of the NF2 gene as presently understood. (Data from Ensembl release 49, March 2008. http://www.ensembl.org/index.html). A and B, The location of the NF2