CHAPTER 155 Molecular Basis of Nociception
This chapter describes the cellular and molecular substrate of nociception. Nociception comprises the afferent activity that is produced in the peripheral and central nervous systems by stimuli of sufficient intensity to (potentially) damage tissue.1 Nociception is initiated by a subset of primary afferent (dorsal root ganglion and trigeminal ganglion) neurons that is specialized for the detection of tissue damage. This specialized subset (commonly referred to as nociceptors) was first identified and its very existence documented in neurophysiologic experiments performed in the late 1960s.2,3 Nociceptors innervate all tissues of the body except for the neuraxis. The cornea, teeth, internal surface of the tympanic membrane, dura, and venous and bony sinuses within the cranium are innervated mainly, if not exclusively by nociceptors. Other tissues of the body are innervated by both nociceptive and non-nociceptive (low-threshold mechanoreceptor and thermoreceptor) primary afferent neurons. Innervation of the body surface by peripheral nerves follows the well-known pattern of radicular dermatomes.
Table 155-1 provides a breakdown of the various nociceptive and non-nociceptive fiber groups in peripheral nerves and the velocities at which they conduct action potentials along their axons. It can be seen that conduction velocity varies as a function of both axon diameter and extent of myelination. The vast majority of nociceptors conduct action potentials relatively slowly because they have small-diameter, thinly myelinated (group III/Aδ fiber), or unmyelinated (group IV/C fiber) axons, as do low-threshold thermoreceptors. Low-threshold, non-nociceptive mechanosensitive primary afferent neurons tend to have large-diameter, myelinated (Aβ) axons. Contrary to general belief, nociceptors with large-diameter, myelinated axons do exist4 but are much less numerous than nociceptors with small-diameter myelinated or unmyelinated fibers, and their input may not reach consciousness. Consequently, input from nociceptors with thinly myelinated (Aδ) axons is typically responsible for the “first pain” felt after sudden stimulation of the foot (by stepping on a nail or a hot surface), whereas nociceptors with unmyelinated (C) fibers are responsible for the “second pain” evoked by such stimuli.
Under normal circumstances, nociceptors produce action potential discharge only in response to tissue-damaging stimuli and have the ability to code the intensity of a noxious stimulus by proportionally varying the frequency of action potential discharge. In contrast, non-nociceptive afferents (sensory receptors specialized for the detection of low-intensity, innocuous stimuli) are unable to signal the intensity of noxious stimuli because the firing frequency of these neurons becomes saturated at noxious levels of stimulation. Microneurographic recordings5 and microstimulation with microelectrodes inserted into the fascicles of peripheral nerves make possible a direct correlation between action potential discharge in identified primary afferent neurons and concomitant reports of sensations in humans.6–10 Skin is densely innervated by nociceptors, and the sensory pathways preserve a high degree of somatotopic organization, as shown by the small projected receptive fields of the sensations that are evoked by electrical stimulation of nociceptors through the microneurography electrodes. The electrical receptive fields agree rather well with the receptive fields determined by natural stimulation of nerve endings.8 Electrical activation of very few, perhaps even a single peripheral nociceptor, is sufficient to produce a pain sensation. Initiation of the sensation in these circumstances requires temporal summation of several action potentials. Above the threshold level, the intensity of the sensation is proportional to the stimulus frequency. The quality of pain evoked by electrical stimulation of nociceptors depends on the target tissue and the subtype of nociceptor that is stimulated. Activation of cutaneous Aδ nociceptor afferents typically evokes sharp, prickling pain, whereas stimulation of C-fiber nociceptors causes dull or burning pain (and in some cases itching).8 Recordings in humans have demonstrated that the intensity of burning pain evoked by heat applied to the skin is best matched by the frequency of action potential discharge in mechanoheat and heat-specific nociceptors with C fibers.11 In contrast, the intense burning pain felt after intracutaneous injection of capsaicin is best matched by the level of discharge in mechanoinsensitive (chemospecific) and heat-specific nociceptors with C fibers.12 Activation of nociceptive C fibers that innervate muscle gives rise to cramping pain.13
Properties of Nociceptors That Innervate Different Peripheral Organs
Visceral Nociceptors
Viscera are particularly sensitive to distention but may be quite insensitive to cutting or burning. The peripheral basis of visceral pain differs from that in other body regions.14,15 The distinction between responses to innocuous and noxious stimulation has been more difficult to make for visceral neurons because there is a much wider overlap in their response properties and some neurons are spontaneously active even in the absence of obvious sensitization or intentional stimulation.16 When compared with cutaneous tissues, pain from visceral tissues is more difficult to localize, most likely because of lower innervation density.
Deep Nociceptors of Muscles and Joints
Muscle pain occurs during sustained muscular contraction and ischemia, after trauma, or after eccentric exercise.17,18 Slightly less than half of the group III and IV fibers in muscle are thought to be nociceptors; the remainder are low-threshold afferents thought to signal deep pressure and thermosensitive afferents believed to be involved in thermoregulation (for an extensive review see Mense19). Pain is the major sensation that is ascribed to the joint.20 Joints are frequently affected by inflammatory and degenerative disorders and by injury. Experimentally induced arthritis causes dramatic changes in the response properties of joint afferent fibers.21 Sensitization has been directly documented in long-term electrophysiologic recordings from single identified afferent fibers during the development of acute inflammation.22 These experiments have shown that joints receive substantial innervation by the so-called silent (or mechanically insensitive) nociceptors, which only begin to respond to moderate-strength stimuli once inflammation becomes established.
Nociceptors at the Body Surface
Because of easy accessibility to experimental manipulation, cutaneous2,3 and corneal24,25 nociceptive neurons have been studied extensively. Much of the information on nociceptors provided later is based on studies of cutaneous nociceptors.
Physiologic Subtypes of Cutaneous Nociceptors
Collectively, nociceptive primary afferent neurons are able to sense three (thermal, mechanical, and chemical) submodalities of noxious stimulation, whereas individual nociceptors are inhomogeneous and respond to various combinations of the three submodalities. Most common are polymodal nociceptors that respond to mechanical and heat stimuli. These include A-fiber type II mechanoheat nociceptors (AMHs), which have a heat threshold of approximately 45°C, and type I AMHs, which have a heat threshold that is higher (>51°C). The latter become sensitized to heat by brief (≈30-second) exposure to high temperatures. Polymodal nociceptors in the C-fiber group are referred to as type 1A nociceptors.26 Type 1A nociceptors are typically excited by moderately intense mechanical and heat stimuli in excess of 42°C. Approximately half of type 1A nociceptors also respond to noxious cold (near-freezing) temperatures. A second major group of C-fiber nociceptors is the type 1B afferents,26 which respond with a vigorous discharge to noxious chemical stimuli but are essentially insensitive to mechanical and thermal stimulation. Mechanically insensitive C-fiber nociceptors that react only to noxious heat or cold are known to also exist.12 The existence of different subtypes of nociceptor, some that respond to all submodalities of noxious stimulation and others that respond to only some, implies that the nociceptors express different, more or less specialized, transducer molecules.
Nociceptive Transduction Mechanisms
Thermosensitivity
A major breakthrough in the search for molecules that may be responsible for the transduction of noxious stimuli arrived with the cloning of a membrane ion channel called TRPV1 (for transient receptor potential vanilloid subtype 1).27 TRPV1 responds to heat with a threshold of about 42°C, a temperature comparable to the heat threshold of most heat-sensitive C-fiber nociceptors. Additional thermosensitive channels (members of the TRP superfamily) were cloned soon thereafter: TRPV2, a membrane channel with a higher threshold for heat activation (>52°C28) that is comparable to the temperature threshold of some mechanoheat nociceptors with Aδ fibers (the type I AMHs); TRPA1, which may mediate response to noxious cold stimuli (with a threshold for activation of around 15°C); the warm-sensitive TRPV3 and TRPV4 (apparent thresholds of 32°C to 39°C and 27°C to 34°C, respectively),29 and TRPM8, an ion channel that is activated by gentle cooling from neutral skin temperature (≈32°C). All of these channels are excellent candidates for thermal transduction by non-nociceptive thermoreceptors (the so-called warm and cold fibers, respectively). Responses of TRPV3 receptors continue to increase as the stimulus temperature is increased into the noxious range,30 thus suggesting that in addition to sensing warmth, TRPV3 receptors may contribute to nociception. Three additional TRP channels, TRPM2, TRPM4, and TRPM5, can also be activated by warm temperatures; however, evidence for their expression in skin is still lacking.29 All thermosensitive TRP molecules are nonselective cation channels with a high temperature coefficient (Q10 ≈ 20) governing the kinetics of opening and closing of the channel pore. Other molecules, such as the highly mechanosensitive and thermosensitive channels TREK-1 (a “TWIK-related potassium channel,” where TWIK stands for “tandem P domain in a weak inward rectifier K+ channel”) and TRAAK (TWIK-related arachidonic acid–stimulated K+ channel), may contribute to the thermosensitivity of primary afferent neurons.31 Although these known thermosensitive channels cover the entire temperature range from 0°C to higher than 50°C,32 behavioral studies on transgenic mice, pharmacologic studies on native sensory nerve endings in vitro, and patch-clamp recordings from cultured dorsal root ganglion neurons indicate that additional thermosensitive ion channels remain to be cloned.
Chemosensitivity
Chemonociceptors (Fig. 155-1) express a multitude of specific molecular receptors for the endogenous inflammatory mediators bradykinin (both B2 and B1 receptors), serotonin (5-HT3 receptors), prostaglandin E2 (EP receptors), histamine, and protons (the acid-sensitive ion channels ASIC 1, 2, and 3).33–42 Sensitivity of nociceptors to these inflammatory mediators plays a central role in many forms of inflammatory pain.43,44 Nociceptive neurons also express receptors for extracellular adenosine triphosphate (ATP), which is released in large quantities from neighboring cells when they are damaged. Most prominent among the receptors for ATP are metabotropic receptors made of P2Y subunits and ionotropic receptors consisting of P2X2 and P2X3 subunits. Administration of P2X3 antagonist drugs or antisense oligonucleotides to animals reduces both inflammatory hyperalgesia and neuropathic pain.45
TRPA1 stands out by being activated by environmental pollutants and acrid chemicals such formaldehyde, acrolein, and the tear gases CN, CR, and CS.46 Other exogenous chemicals that activate TRPA1 are allicin, mustard oils (allyl isothiocyanates) in wasabi and horseradish, cinnamaldehyde, eugenol, gingerol, carvacrol, 2-pentenal, icilin, low concentrations of menthol (<100 µM, higher concentrations cause an open channel block), and Δ9-tetrahydrocannabinol. TRPA1 is also activated by chemicals originating from within the body such as arachidonic acid, bradykinin, 15-deoxy-Δ12,14-prostaglandin J2 (a cyclopentane prostaglandin D2 metabolite), and products of oxidative stress, including hydrogen peroxide, 4-hydroxynonenal, 4-oxononenal, and 4-hydroxyhexenal.47 Most compounds known to activate TRPA1 are able to covalently bind cysteine residues.48
Mechanosensitivity
There are good reasons to believe that noxious mechanical stimuli are transduced by ion channels that are gated open or closed by stretch or a change in curvature of the membrane. Such channels were recorded with patch-clamp methods, but the molecular identities of these channels remain largely to be elucidated. On the basis of sequence similarity to mechanosensitive molecules found in Caenorhabditis elegans, certain mec-related proteins have been tested in mammals. Mutant mice lacking a mec-2–related protein, stomatin-like protein 3 (SLP3), show a marked loss of touch sensitivity. SLP3 appears to be essential for mechanotransduction in a subset of cutaneous touch receptors. By contrast, similar studies indicate that three neuronal degenerin/epithelial sodium channel isoforms (the acid-sensing ion channels ASIC 1, 2, and 3) are not indispensable for mechanotransduction.29 It therefore appears that several families of mechanosensitive molecules contribute to low-threshold mechanotransduction, but the ion channels involved in high-threshold mechanotransduction (mechanonociception) remain to identified.
Some thermosensitive TRP channels have a dual function as mechanotransducer molecules. In animal models of painful peripheral neuropathy associated with vincristine chemotherapy, alcoholism, diabetes, and human immunodeficiency virus/acquired immunodeficiency syndrome therapy, mechanical hyperalgesia (hypersensitivity to mechanical stimuli) was markedly reduced by spinal intrathecal administration of oligodeoxynucleotides antisense to TRPV4 mRNA.49 (This treatment of mechanical hyperalgesia is effective because the dorsal root ganglia that harbor the cell bodies of primary afferent neurons reside within the intrathecal space and are therefore accessed directly by compounds injected intrathecally.) Two additional thermosensitive TRPV channels (TRPV1 and TRP2) have been implicated in mechanosensory signaling. TRPV2 is a candidate mechanotransduction channel because it can be activated by hypotonic and stretch stimuli in vitro.29 TRPV1 might contribute to mechanotransduction in visceral organs.29 It is unclear whether TRPV2 participates in mechanonociception because TRPV2 is expressed in large-diameter somatosensory neurons, most of which are not nociceptive (see earlier). TRPA1 has likewise been proposed to be involved in mechanotransduction. Recent work has demonstrated that inhibition of TRPA1 function reduces the mechanical hypersensitivity produced by inflammation. Acute application in a nerve-skin preparation of HC-030031, a selective TRPA1 antagonist, markedly reduced mechanically evoked action potential firing in rodent C fibers, particularly at high-intensity forces, but had no effect on the mechanical responsiveness of Aδ-fiber nociceptors.50 Another recent study demonstrated that ND-C cells (a hybrid cell line derived from neonatal rat dorsal root ganglion neurons that expresses mechanosensitive ion channels and provides a useful expression system for testing candidate mechanosensitive ion channels), when transfected with the candidate mechanotransducer channel TRPA1, does not show an increase in mechanoactivated currents.51 Together, these perplexing and controversial results indicate that many types of molecules (yet to be identified) must work in concert to control the sensitivity of mechanonociceptors.
Sensitization of Nociceptors (Primary Hyperalgesia)
“Mechanically insensitive” afferents52–54 can be quite insensitive in normal healthy tissue but become markedly sensitized to mechanical stimuli by exposure to inflammatory mediators52 released by resident mast cells or by immune cells (macrophages, others) attracted to the site of tissue injury. Inflammatory mediators tend to lower the threshold for nociceptor excitation (causing allodynia, or pain evoked by normally innocuous stimuli) and increase nociceptor responses to suprathreshold stimuli (causing primary hyperalgesia, or more intense pain evoked by normally less painful stimuli).43 Among the compounds that sensitize nociceptive neurons are eicosanoids (the cyclooxygenase products prostaglandins E1 and E2 and the 15-lipoxygenase product 8[R],15[S]-dihydroxyeicosatetraenoic acid55,56), kinins (bradykinin57 and T-kinin58), 5-hydroxytryptamine (5-HT), low pH,59,60 and ATP (see Fig. 155-1).61 Nociceptors are also sensitized by extracellular enzymes acting on the G protein–coupled protease-activated receptor 2 (PAR-2). Mast cell tryptase, trypsin, and trypsin-like proteases and coagulation factors VIIa and Xa are considered endogenous agonists of PAR-2. Inflammatory mediators control nociceptor action potential discharge by modulating voltage-gated ion channels through intracellular pathways that involve second messenger molecules such as inositol 1,4,5,-triphosphate, diacylglycerol, or cyclic adenosine monophosphate.33,62
Epithelial Cells as Sensory Receptor Cells
The previous description of nociceptive cells is based on the traditional view that nociceptive transduction in mammals occurs in the terminals of primary sensory neurons. In this traditional view, the surrounding cells (e.g., skin keratinocytes) are thought to provide only physical and trophic support to the nerve terminals. An emerging alternative view is that rather than being mere bystanders in the transduction process, non-neuronal cells are involved actively by providing function as primary transducers of physical and chemical stimuli and communicating excitatory events to neighboring somatosensory afferent fibers by releasing certain chemical messenger molecules. Keratinocytes express a number of receptor molecules that have been implicated in nociception or temperature sensation. In fact, some of these receptor molecules (e.g., TRPV3 and TRPV4) are more readily detected in keratinocytes than in primary somatosensory neurons. When tested in culture, keratinocytes generate measurable membrane currents after thermal or chemical stimulation that lead to changes in intracellular free calcium concentration. Thus, keratinocytes possess the transduction mechanism that would be required for stimulated release of chemical substances (e.g., ATP) for which nociceptive neurons express molecular receptors. Although such a mode of transduction of mechanical stimuli was demonstrated recently in vitro, direct evidence of keratinocyte to sensory neuron signaling in vivo is thus far lacking.29 It is worth noting in this context that specialized epithelial (non-neuronal) cells in the nasal epithelium (called solitary chemoreceptor cells) appear to play an active role in the transduction of sensory irritant stimuli by relaying nociceptive information to the adjacent nerve endings of trigeminal ganglion neurons.63
Neurogenic Inflammation
Some small-diameter primary afferent neurons, when activated by stimuli in the noxious range, play a major efferent function in inflammatory processes through the release of proinflammatory (calcitonin gene–related peptide [CGRP], substance P) molecules via the so-called axon reflex.64 “Axon reflex” implies action potential conduction in the efferent direction (which happens when an action potential conducted in the orthodromic direction along one terminal branch in the periphery splits or is deflected at the axonal branch point and then, after being propagated in the antidromic direction, invades the other sensory terminal branch). Depolarization at the terminal causes the influx of calcium, which leads to release of neuropeptides (see Fig. 155-1). There is some evidence that neurogenic release of substance P contributes to the severity of arthritis.65 It has been suggested that neurogenic release of CGRP is one of the driving forces in migraine, but this issue remains controversial.66,67 In addition to the proinflammatory action of substance P and CGRP, there is also experimental evidence for the anti-inflammatory action of other neuropeptides (e.g., somatostatin) released from the sensory nerve endings of primary afferent neurons.68
Centripetal Transfer of Information
Molecules Responsible for Generation and Conduction of Action Potentials in Peripheral Nerve Fibers
Activation of mechanosensitive, thermosensitive, or chemosensitive molecules leads to depolarization of the sensory nerve ending. This process is highly modulated by intracellular messenger molecules (Fig. 155-2). Depolarization of the nerve ending above a certain threshold causes the generation of action potentials and their conduction along the axon. This process is mediated by voltage-gated sodium channels in conjunction with voltage-gated calcium and voltage-gated potassium channels. Primary afferent neurons use a variety of voltage-gated Na+ channels that differ in their sensitivity to tetrodotoxin (TTX). Some voltage-gated sodium channels are highly sensitive to TTX, some are resistant to TTX, and some are insensitive to TTX. TTX-resistant Na+ currents are mediated by the NaV1.8 and NaV1.9 protein subunits, TTX-insensitive currents by NaV1.5, and TTX-sensitive currents by the NaV1.1, NaV1.2, NaV1.3, NaV1.6, and NaV1.7 subunits. The prevailing view is that NaV1.8-mediated currents are mainly responsible for spike initiation in nociceptor terminals and cell bodies whereas axonal conduction is mediated by the TTX-sensitive channels NaV1.6 and NaV1.7. NaV1.7 subunits no doubt play a central role because mutations in NaV1.7 have been found to be responsible for a spectrum of pain disorders ranging from erythermalgia to complete insensitivity to painful stimuli.69 However, the exclusion of NaV1.8 channels from axonal conduction in nociceptors may have to be revised in view of a recent study70 in which it was documented that activity-dependent slowing26,71 in meningeal C-fiber nociceptors is mediated by use-dependent entry of TTX-resistant Na+ channels into the inactivated, slow-recovery state. Mechanosensitive and mechanoinsensitive nociceptors probably use a somewhat different mix of voltage-gated ion channel subunits for conduction of action potentials because these two major classes of nociceptors differ in the pattern of activity-dependent slowing of conduction velocity,26 but the particular ion channel subunits and ion pumps that contribute to this biophysical difference remain to be identified.
Axonal Projections
The cell bodies of all nociceptors are located in either the dorsal root ganglia or the trigeminal ganglia, where they are mixed with the cell bodies of non-nociceptive neurons and arranged in a loosely somatotopic fashion. Each cell body is connected to the peripheral and central branches of its axon via a short branch forming a T-shaped connection. Axons of nociceptive neurons project through the peripheral nerves, through the dorsal root ganglia (or the trigeminal ganglia), and through the dorsal roots accompanied by axons of non-nociceptive neurons (Fig. 155-3). The axons of visceral nociceptive neurons project through visceral nerves along with the axons of sympathetic and parasympathetic neurons (see Fig. 155-3). The central branch projects through the dorsal root to the spinal cord (or in the case of trigeminal nociceptors to the brainstem). As the spinal nerve root approaches the spinal cord, it splits into smaller rootlets. Axons with a larger diameter tend to aggregate in the medial and central rootlets, whereas small-diameter axons congregate in the more lateral rootlets, but the separation is not absolute. Early workers reported that cutting the lateral rootlets abolishes some behavior and reflexes that are normally triggered by nociceptive input,72 thus suggesting that chronic pain of peripheral origin could be treated by selective rhizotomy. However, subsequent studies showed that it is probably impossible to destroy nociceptive fibers selectively by cutting the lateral rootlets. Modern interpretation of the classic experiments is that the nociceptive deficits were due to vascular damage associated with cutting the dorsal roots.73 As nociceptive axons in the dorsal roots enter the superficial root entry zone of the spinal cord, they bifurcate into short ascending and descending branches to form Lissauer’s tract, which caps the dorsal horn. From Lissauer’s tract, the nociceptors enter particular layers (laminae) of the dorsal horn (Fig. 155-4), where they synapse with local interneurons and projection neurons.
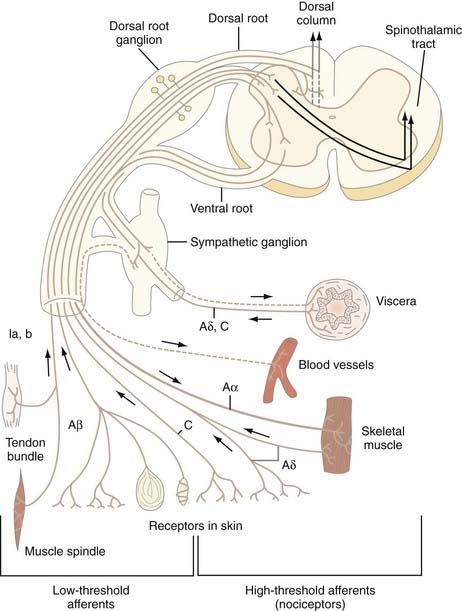
FIGURE 155-3 Schema of a spinal nerve and the different types of low- and high-threshold afferent fibers that it contains.
(From Bonica JJ. Anatomic and physiological basis of nociception and pain. In: Bonica JJ, ed. The Management of Pain, vol 1. Philadelphia: Lea & Febiger; 1990:28-94.)
There are some exceptions to the general rule that sensory information enters the spinal cord via the dorsal roots.74 Some afferent axons enter via the ventral root.74 About 30% of axons in the ventral root are unmyelinated, even in segments that contribute little to autonomic outflow. These unmyelinated axons might carry nociceptive information. Figure 155-5 shows the two possibilities for the ultimate course of these aberrant axons. Most fibers that enter the ventral root turn back and then enter the spinal cord through the dorsal root as usual (Fig. 155-5B). A few fibers also probably penetrate the cord from the ventral root and traverse the ventral horn to terminate in superficial layers of the dorsal horn (see Fig. 155-5A). These findings have been cited as one reason why dorsal rhizotomy frequently fails to relieve pain75 and why dorsal root ganglionectomy may be a better surgical strategy for the elimination of all nociceptive afferent fibers to a spinal segment.
Synaptic Transmission and Segmental Facilitatory Mechanisms
Primary afferent neurons synthesize excitatory amino acids and a wide variety of neuropeptides, any of which could potentially act as a synaptic neurotransmitter/neuromodulator.76 The excitatory acid glutamate is the substance responsible for the fast excitatory synaptic actions of primary nociceptive neurons on second-order neurons in the spinal cord or trigeminal brainstem nuclei. Fast excitation is mediated by ion currents flowing through the membrane-spanning pore of α-amino-3-hydroxy-5-methyl-4-isoxazolepropionate (AMPA) and N-methyl-D-aspartate (NMDA) receptors. Substance P in conjunction with kinin receptors appears to mediate some of the slower synaptic actions. Other neuropeptides that are released from small-diameter primary afferent neurons (e.g., CGRP, vasoactive intestinal polypeptide, and somatostatin) may modulate synaptic transmission.77
Secondary Hyperalgesia
Intense activation of nociceptive input has profound effects on synaptic transmission in the nociceptive pathways. Central nociceptive neurons become sensitized and begin to respond vigorously to previously less effective or ineffective input from low-threshold sensory receptors.78–84 Such activation of ascending nociceptive neurons is believed to be the cause of secondary hyperalgesia and allodynia after peripheral injury.
Preemptive Anesthesia
The surgeon and anesthesiologist need to take into consideration that unless proper countermeasures are taken, a surgical intervention is likely to trigger secondary hyperalgesia. General anesthesia does not prevent the activation of nociceptors, nor does it prevent the development of hyperalgesia. Discharge of nociceptive fibers and the development of hyperalgesia can be blocked effectively by infiltrating the surgical site with a local anesthetic (preemptive anesthesia). Controlled studies have shown that prevention of secondary hyperalgesia by preemptive local anesthesia substantially lessens the requirement for analgesic medication after surgery and hastens patients’ recovery.85 Still, the local anesthetic needs to be chosen carefully because lidocaine was recently shown to cause neurogenic inflammation by activating TRPV1 and TRPA1 channels.86
Alessandri-Haber N, Dina OA, Joseph EK, et al. Interaction of transient receptor potential vanilloid 4, integrin, and SRC tyrosine kinase in mechanical hyperalgesia. J Neurosci. 2008;28:1046-1057.
Andersson DA, Gentry C, Moss S, et al. Transient receptor potential A1 is a sensory receptor for multiple products of oxidative stress. J Neurosci. 2008;28:2485-2494.
Belmonte C, Viana F. Molecular and cellular limits to somatosensory specificity. Mol Pain. 2008;4:14.
Burgess PR, Perl ER. Myelinated afferent fibres responding specifically to noxious stimulation of the skin. J Physiol. 1967;190:541-562.
Caterina MJ, Schumacher MA, Tominaga M, et al. The capsaicin receptor: a heat-activated ion channel in the pain pathway. Nature. 1997;389:816-824.
Coggeshall RE, Applebaum ML, Fazen M, et al. Unmyelinated axons in human ventral roots, a possible explanation for the failure of dorsal rhizotomy to relieve pain. Brain. 1975;98:157-166.
De Col R, Messlinger K, Carr RW. Conduction velocity is regulated by sodium channel inactivation in unmyelinated axons innervating the rat cranial meninges. J Physiol. 2008;586:1089-1103.
Drenth JPH, Waxman SG. Mutations in sodium-channel gene SCN9A cause a spectrum of human genetic pain disorders. J Clin Invest. 2007;117:3603-3609.
Edvinsson L. CGRP blockers in migraine therapy: where do they act? Br J Pharmacol. 2008;155:967-969.
Hilliges M, Weidner C, Schmelz M, et al. ATP responses in human C nociceptors. Pain. 2002;98:59-68.
LaMotte RH, Campbell JN. Comparison of responses of warm and nociceptive C-fiber afferents in monkey with human judgements of thermal pain. J Neurophysiol. 1978;41:509-528.
Leffler A, Fischer MJ, Rehner D, et al. The vanilloid receptor TRPV1 is activated and sensitized by local anesthetics in rodent sensory neurons. J Clin Invest. 2008;118:763-776.
Marchettini P, Simone DA, Caputi G, et al. Pain from excitation of identified muscle nociceptors in humans. Brain Res. 1996;740:109-116.
Silver WL, Finger TE. The anatomical and electrophysiological basis of peripheral nasal trigeminal chemoreception. Ann N Y Acad Sci. 2009;1170:202-205.
Sugiura T, Tominaga M, Katsuya H, et al. Bradykinin lowers the threshold temperature for heat activation of vanilloid receptor 1. J Neurophysiol. 2002;88:544-548.
Tominaga M, Caterina MJ, Malmberg AB, et al. The cloned capsaicin receptor integrates multiple pain-producing stimuli. Neuron. 1998;21:531-543.
Torebjörk HE, Ochoa JL. Specific sensations evoked by activity in single identified sensory units in man. Acta Physiol Scand. 1980;110:445-447.
Vallbo AB, Hagbarth K-E, Torebjörk HE, et al. Somatosensory, proprioceptive, and sympathetic activity in human peripheral nerves. Physiol Rev. 1979;59:919-957.
Weidner C, Schmidt R, Schmelz M, et al. Time course of post-excitatory effects separates afferent human C fibre classes. J Physiol. 2000;527:185-191.
1 Loeser JD, Treede R-D. The Kyoto protocol of IASP basic pain terminology. Pain. 2008;137:473-477.
2 Burgess PR, Perl ER. Myelinated afferent fibres responding specifically to noxious stimulation of the skin. J Physiol. 1967;190:541-562.
3 Perl ER. Pain and nociception. In: Brookhart JM, Mouncastle VB, editors. Handbook of Physiology, Section 1, The Nervous System, vol III: Sensory Processes, Part 2. Bethesda, MD: American Physiological Society; 1984:915-975.
4 Lawson SN. Phenotype and function of somatic primary afferent nociceptive neurones with C-, Aδ- or Aα/β-fibres. Exp Physiol. 2002;87:239-244.
5 Vallbo AB, Hagbarth K-E, Torebjörk HE, et al. Somatosensory, proprioceptive, and sympathetic activity in human peripheral nerves. Physiol Rev. 1979;59:919-957.
6 Torebjörk HE. Afferent C units responding to mechanical, thermal, and chemical stimuli in human non-glabrous skin. Acta Physiol Scand. 1974;92:374-390.
7 Torebjörk HE, Ochoa JL. Specific sensations evoked by activity in single identified sensory units in man. Acta Physiol Scand. 1980;110:445-447.
8 Ochoa J, Torebjörk E. Sensations evoked by intraneural microstimulation of C nociceptor fibres in human skin nerves. J Physiol. 1989;415:583-599.
9 Handwerker HO, Forster C, Kirchhoff C. Discharge patterns of human C-fibers induced by itching and burning stimuli. J Neurophysiol. 1991;66:307-315.
10 Handwerker HO, Kobal G. Psychophysiology of experimentally induced pain. Physiol Rev. 1993;73:639-671.
11 LaMotte RH, Campbell JN. Comparison of responses of warm and nociceptive C-fiber afferents in monkey with human judgements of thermal pain. J Neurophysiol. 1978;41:509-528.
12 Baumann TK, Simone DA, Shain C, et al. Neurogenic hyperalgesia: the search for the primary cutaneous afferent fibers that contribute to capsaicin-induced pain and hyperalgesia. J Neurophysiol. 1991;66:212-227.
13 Marchettini P, Simone DA, Caputi G, et al. Pain from excitation of identified muscle nociceptors in humans. Brain Res. 1996;740:109-116.
14 Cervero F. Sensory innervation of the viscera: peripheral basis of visceral pain. Physiol Rev. 1994;74:95-138.
15 Sengupta JN, Gebhart GF. Gastrointestinal afferent fibers and sensation. In: Johnson LR, editor. Physiology of the Gastrointestinal Tract. 3rd ed. New York: Raven Press; 1994:483-519.
16 McMahon SB. Mechanisms of cutaneous, deep and visceral pain. In: Wall PD, Melzack R, editors. Textbook of Pain. 2nd ed. Edinburgh: Churchill Livingstone; 1994:129-151.
17 Mense S. Physiology of nociception in muscles. In: Fricton JR, Awad E, editors. Advances in Pain Research and Therapy, vol 17. New York: Raven Press; 1990:67-85.
18 Mense S. Considerations concerning the neurobiological basis of muscle pain. Can J Physiol Pharmacol. 1991;69:610-616.
19 Mense S. Nociception from skeletal muscle in relation to clinical muscle pain. Pain. 1993;54:241-289.
20 Schaible H-G, Grubb BD. Afferent and spinal mechanisms of joint pain. Pain. 1993;55:5-54.
21 Hanesch U, Heppelmann B, Messlinger K, et al. Nociception in normal and arthritic joints. In: Willis WDJr, editor. Hyperalgesia and Allodynia. New York: Raven Press; 1992:81-105.
22 Schaible H-G, Schmidt RF. Direct observation of the sensitization of articular afferents during an experimental arthritis. eds. Proceedings of the Vth world conference on Pain. R. Gebhart, GF. Bond. MR, Dubner. Philadelphia: Elsevier. 1988:44-50.
23 Meyer RA, Campbell JN, Raja SN. Peripheral neural mechanisms of nociception. In: Wall PD, Melzack R, editors. Textbook of Pain. 2nd ed. Edinburgh: Churchill Livingstone; 1994:13-44.
24 Belmonte C, Giraldez F. Responses of cat corneal sensory receptors to mechanical and thermal stimulation. J Physiol. 1981;321:355-368.
25 Belmonte C, Gallar J, Pozo MA, et al. Excitation by irritant chemical substances of sensory afferent units in the cat’s cornea. J Physiol. 1991;437:709-725.
26 Serra J, Campero M, Ochoa J, et al. Activity-dependent slowing of conduction differentiates functional subtypes of C fibres innervating human skin. J Physiol. 1999;515:799-811.
27 Caterina MJ, Schumacher MA, Tominaga M, et al. The capsaicin receptor: a heat-activated ion channel in the pain pathway. Nature. 1997;389:816-824.
28 Caterina MJ, Rosen TA, Tominaga M, et al. A capsaicin-receptor homologue with a high threshold for noxious heat. Nature. 1999;398:436-441.
29 Lumpkin EA, Caterina MJ. Mechanisms of sensory transduction in the skin. Nature. 2007;445:858-865.
30 Smith GD, Gunthorpe MJ, Kelsell RE, et al. TRPV3 is a temperature-sensitive vanilloid receptor–like protein. Nature. 2002;418:186-190.
31 Noel J, Zimmermann K, Busserolles J, et al. The mechano-activated K+ channels TRAAK and TREK-1 control both warm and cold perception. EMBO J. 2009;28:1308-1318.
32 Belmonte C, Viana F. Molecular and cellular limits to somatosensory specificity. Mol Pain. 2008;4:14.
33 Rang HP, Bevan S, Dray A. Chemical activation of nociceptive peripheral neurones. Br Med Bull. 1991;47:534-548.
34 Hwang SW, Cho H, Kwak J, et al. Direct activation of capsaicin receptors by products of lipoxygenases: endogenous capsaicin-like substances. Proc Natl Acad Sci U S A. 2000;97:6155-6160.
35 Tominaga M, Caterina MJ, Malmberg AB, et al. The cloned capsaicin receptor integrates multiple pain-producing stimuli. Neuron. 1998;21:531-543.
36 Sutherland SP, Cook SP, McCleskey EW. Chemical mediators of pain due to tissue damage and ischemia. Prog Brain Res. 2000;129:21-38.
37 Robertson B, Bevan S. Properties of 5-hydroxytryptamine3 receptor-gated currents in adult rat dorsal root ganglion neurones. Br J Pharmacol. 1991;102:272-276.
38 Gauldie SD, McQueen DS, Pertwee R, et al. Anandamide activates peripheral nociceptors in normal and arthritic rat knee joints. Br J Pharmacol. 2001;132:617-621.
39 Olah Z, Karai L, Iadarola MJ. Anandamide activates vanilloid receptor 1 (VR1) at acidic pH in dorsal root ganglia neurons and cells ectopically expressing VR1. J Biol Chem. 2001;276:31163-31170.
40 Roberts LA. Anandamide is a partial agonist at native vanilloid receptors in acutely isolated mouse trigeminal sensory neurons. Br J Pharmacol. 2002;137:421-428.
41 Burnstock G. P2X receptors in sensory neurones. Br J Anaesth. 2000;84:476-488.
42 Hilliges M, Weidner C, Schmelz M, et al. ATP responses in human C nociceptors. Pain. 2002;98:59-68.
43 Handwerker HO, Reeh PW. Pain and inflammation. In: Bond MR, Charlton JE, Woolf CJ, editors. Proceedings of the VIth World Congress on Pain. Amsterdam: Elsevier; 1991:59-70.
44 Levine J, Taiwo Y. Inflammatory pain. In: Wall PD, Melzack R, editors. Textbook of Pain. 2nd ed. Edinburgh: Churchill Livingstone; 1994:45-56.
45 Donnelly-Roberts D, McGaraughty S, Shieh C-C, et al. Painful purinergic receptors. J Pharmacol Exp Ther. 2008;324:409-415.
46 Brone B, Peeters PJ, Marrannes R, et al. Tear gasses CN, CR, and CS are potent activators of the human TRPA1 receptor. Toxicol Appl Pharmacol. 2008;231:150-156.
47 Andersson DA, Gentry C, Moss S, et al. Transient receptor potential A1 is a sensory receptor for multiple products of oxidative stress. J Neurosci. 2008;28:2485-2494.
48 Macpherson LJ, Dubin AE, Evans MJ, et al. Noxious compounds activate TRPA1 ion channels through covalent modification of cysteines. Nature. 2007;445:541-545.
49 Alessandri-Haber N, Dina OA, Joseph EK, et al. Interaction of transient receptor potential vanilloid 4, integrin, and SRC tyrosine kinase in mechanical hyperalgesia. J Neurosci. 2008;28:1046-1057.
50 Kerstein PC, del Camino D, Moran MM, et al. Pharmacological blockade of TRPA1 inhibits mechanical firing in nociceptors. Mol Pain. 2009;5:19.
51 Rugiero F, Wood JN. The mechanosensitive cell line ND-C does not express functional thermoTRP channels. Neuropharmacology. 2009;56:1138-1146.
52 Meyer RA, Davis KD, Cohen RH, et al. Mechanically insensitive afferents (MIAs) in cutaneous nerves of monkey. Brain Res. 1991;561:252-261.
53 Handwerker HO, Kilo S, Reeh PW. Unresponsive afferent nerve fibres in the sural nerve of the rat. J Physiol. 1991;435:229-242.
54 McMahon SB, Koltzenburg M. Novel classes of nociceptors: beyond Sherrington. Trends Neurosci. 1990;13:199-201.
55 Martin HA, Basbaum AI, Goetzl EJ, et al. Leukotriene B4 decreases the mechanical and thermal thresholds of C-fiber nociceptors in the hairy skin of the rat. J Neurophysiol. 1988;60:438-445.
56 Cohen RH, Perl ER. Contributions of arachidonic acid derivatives and substance P to the sensitization of cutaneous nociceptors. J Neurophysiol. 1990;64:457-464.
57 Sugiura T, Tominaga M, Katsuya H, et al. Bradykinin lowers the threshold temperature for heat activation of vanilloid receptor 1. J Neurophysiol. 2002;88:544-548.
58 Cohen RH, Perl ER. Chemical factors in the sensitization of cutaneous nociceptors. In: Hamann W, Iggo A, editors. Transduction and Cellular Mechanisms in Sensory Receptors, vol 74, Progress in Brain Research edition. New York: Elsevier, 1988.
59 Steen KH, Reeh PW, Anton F, et al. Protons selectively induce lasting excitation and sensitization to mechanical stimulation of nociceptors in rat skin, in vitro. J Neurosci. 1992;12:86-95.
60 Steen KH, Reeh PW. Sustained graded pain and hyperalgesia from experimental tissue acidosis in human subjects. Soc Neurosci Abstr. 1992;18:384.
61 Tominaga M, Wada M, Masu M. Potentiation of capsaicin receptor activity by metabotropic ATP receptors as a possible mechanism for ATP-evoked pain and hyperalgesia. Proc Natl Acad Sci U S A. 2001;98:6951-6956.
62 Rang HP, Bevan S, Dray A. Nociceptive peripheral neurons: cellular properties. In: Wall PD, Melzack R, editors. Textbook of Pain. 2nd ed. Edinburgh: Churchill Livingstone; 1994:57-78.
63 Silver WL, Finger TE. The anatomical and electrophysiological basis of peripheral nasal trigeminal chemoreception. Ann N Y Acad Sci. 2009;1170:202-205.
64 Levine JD, Fields HL, Basbaum AI. Peptides and primary afferent nociceptor. J Neurosci. 1993;13:2273-2286.
65 Levine JD, Clark R, Devor M, et al. Intraneuronal substance P contributes to the severity of experimental arthritis. Science. 1984;226:547-549.
66 Tfelt-Hansen P, Le H. Calcitonin gene–related peptide in blood: is it increased in the external jugular vein during migraine and cluster headache? A review. J Headache Pain. 2009;10:137-143.
67 Edvinsson L. CGRP blockers in migraine therapy: where do they act? Br J Pharmacol. 2008;155:967-969.
68 Szolcsányi J, Pintér E, Helyes ZS. Sensocrine function of capsaicin-sensitive nociceptors mediated by somatostatin regulates against inflammation and hyperalgesia. In: Handwerker HO, Brune K, editors. Hyperalgesia: Molecular Mechanisms and Clinical Applications. Seattle: IASP Press; 2004:112-128.
69 Drenth JPH, Waxman SG. Mutations in sodium-channel gene SCN9A cause a spectrum of human genetic pain disorders. J Clin Invest. 2007;117:3603-3609.
70 De Col R, Messlinger K, Carr RW. Conduction velocity is regulated by sodium channel inactivation in unmyelinated axons innervating the rat cranial meninges. J Physiol. 2008;586:1089-1103.
71 Weidner C, Schmidt R, Schmelz M, et al. Time course of post-excitatory effects separates afferent human C fibre classes. J Physiol. 2000;527:185-191.
72 Ranson SW, Billingsley PR. The conduction of painful afferent impulses in the spinal nerves. Am J Physiol. 1916;40:571-584.
73 Willis WD, Coggeshall RE. Sensory Mechanisms in the Spinal Cord. New York: Plenum Press; 1978.
74 Coggeshall RE. Law of separation of function of the spinal roots. Physiol Rev. 1980;60:716-755.
75 Coggeshall RE, Applebaum ML, Fazen M, et al. Unmyelinated axons in human ventral roots, a possible explanation for the failure of dorsal rhizotomy to relieve pain. Brain. 1975;98:157-166.
76 Carr PA, Nagy JI. Emerging relationships between cytochemical properties and sensory modality transmission in primary sensory neurons. Brain Res Bull. 1993;30:209-219.
77 Millan MJ. The induction of pain: an integrative review. Prog Neurobiol. 1999;57:1-164.
78 Dougherty PM, Willis WD. Enhancement of spinothalamic neuron responses to chemical and mechanical stimuli following combined micro-iontophoretic application of N-methyl-D-aspartic acid and substance-P. Pain. 1991;47:85-93.
79 Meller ST. Thermal and mechanical hyperalgesia. APS J. 1994;3:215-231.
80 Coderre TJ. Examination of the evidence that distinct excitatory amino acid receptors and intracellular messengers mediate thermal and mechanical hyperalgesia. APS J. 1994;3:232-239.
81 Dougherty PM, Mittman S, Sorkin LS. Hyperalgesia and amino acids. Receptor selectivity based on stimulus intensity and a role for neuropeptides. APS J. 1994;3:240-248.
82 Henry JL, Radhakrishnan V. Hyperalgesia following noxious thermal, mechanical, or chemical stimulation involves overlapping spinal mechanisms and interactive participation of excitatory amino acids and neuropeptides. APS J. 1994;3:249-256.
83 Yaksh TL, Malmberg AB. Central pharmacology of nociceptive transmission. In: Wall PD, editor. Textbook of Pain. 2nd ed. Edinburgh: Churchill Livingstone; 1994:165-200.
84 Simone DA, Sorkin LS, Oh U, et al. Neurogenic hyperalgesia: central neural correlates in responses of spinothalamic neurons. J Neurophysiol. 1991;66:228-246.
85 Kelly DJ, Ahmad M, Brull SJ. Preemptive analgesia II: recent advances and current trends. Can J Anaesth. 2001;48:1091-1101.
86 Leffler A, Fischer MJ, Rehner D, et al. The vanilloid receptor TRPV1 is activated and sensitized by local anesthetics in rodent sensory neurons. J Clin Invest. 2008;118:763-776.