Chapter 23 Metastatic Disease
Bone, Spinal Cord, Brain, Liver, and Lung
Although palliative care is a large part of the clinical practice of oncology, studies show that cancer pain is often inadequately managed.1,2 Among painful metastases, osseous metastases3 remain the most common cause of intractable pain in cancer patients. Bone is the third most common site of metastases after lung and liver.4 Metastases usually become apparent after the diagnosis of the primary tumor, but in up to 23% of patients they are the presenting problem. Bone pain results in immobility, anxiety, and depression and it severely affects a patient’s quality of life. Therefore treatment of bone pain is a high priority.
Brain metastases are a devastating complication of malignant disease. Most patients with brain metastases succumb to metastatic disease within a few months.5,6 Relatively few are candidates for open surgery, which can carry the risk of severe sequelae. The advent of radiosurgical techniques and high-quality magnetic resonance imaging (MRI) of the brain has greatly changed the outcomes of patients with brain metastases, especially when combined with whole-brain irradiation. Patients commonly have improved duration of cognitive performance and, in some, prolonged survival times.6 The success of this approach is limited to patients with tumors in favorable regions of the brain. Among patients with lung cancer, the actuarial incidence of brain metastases can exceed 70%.7,8 Other cancers may also have high rates of metastasis, including 19% in female breast cancers.9
Liver metastases are common in many cancers and, like brain metastases, are usually associated with a limited median survival time. Liver metastases occur in 40% to 70% of patients with progressive colorectal cancer and in a similar range of patients with progressive cancer of the breast or lung.10,11 Treatment for liver metastases is often difficult and, therefore, inadequately managed.9 Recently there has been an effort to treat liver metastases using localized therapies such as stereotactic radiation techniques, radiofrequency ablation, hyperthermia, and embolic therapy in addition to resection. There has been satisfactory success with many of these treatments, which appear to be improving patient outcomes.
Bone Metastases
Table 23-1 shows the prevalence of skeletal metastases in several autopsy series.12 The marked variation may be attributed to differences in the thoroughness of the pathologic examination of the skeleton. Bone scintigraphic surveys have, in general, reported higher rates of bone metastases. In a study by Tofe and colleagues,13 bone scans of 1143 patients with a nonosseous primary tumor were examined; 61% of the patients had an abnormal bone scan finding, and 33% had breast, lung, or prostate primary cancer.
TABLE 23-1 Prevalence of Skeletal Metastases at Primary Site
Primary Site | Prevalence (%) |
---|---|
Breast | 47-85 |
Prostate | 54-85 |
Thyroid | 28-60 |
Kidney | 33-40 |
Bronchus | 32-40 |
Esophagus | 5-7 |
Other gastrointestinal sites | 3-11 |
Rectum | 8-13 |
Bladder | 42 |
Cervix | 0 |
Ovaries | 9 |
Liver | 16 |
Data from Galasko CSB: Incidence and distribution of skeletal metastases. Clin Orthop Relat Res 210:14-22, 1986.
In a prospective series of hospital patients with bone metastases, the tumors carrying the highest risk of bone metastases were those originating in the prostate (32.4%), breast (21.9%), kidney (16.4%), thyroid (11.7%), lung (10.9%), and testes (10.2%).2 Table 23-2 shows the incidence of patients developing bone metastases by primary site.
TABLE 23-2 Incidence of Bone Metastases According to Primary Site
Primary Site | No. Patients | Patients with Bone Metastases (%) |
---|---|---|
Breast | 6423 | 17 |
Prostate | 144 | 16 |
Esophagus | 451 | 6 |
Lung | 589 | 5 |
Bladder | 172 | 5 |
Rectum | 274 | 4 |
Thyroid | 107 | 4 |
Uterine cervix | 1981 | 3 |
Uterine corpus | 509 | 3 |
Head and neck | 2860 | 2 |
Ovaries | 586 | 1 |
Colon | 153 | 1 |
Stomach | 118 | 1 |
Data from Tubiana-Hulin M: Incidence, prevalence and distribution of bone mets. Bone 12:S9-S10, 1991.
The distribution of skeletal metastases from breast cancer is shown in Table 23-3. Similar distributions have been noted from primary cancers in the prostate, lung, and breast.14,15,16,17
TABLE 23-3 Distribution of Skeletal Metastases in 212 Breast Cancer Patients
Anatomic Site | At Presentation (%)* | At Any Time (%)* |
---|---|---|
Lumbar spine | 52 | 59 |
Thoracic spine | 35 | 57 |
Pelvis | 31 | 49 |
Ribs | 18 | 30 |
Femur | 15 | 24 |
Skull | 12 | 20 |
Cervical spine | 11 | 17 |
Humerus | 8 | 13 |
Other sites | 3 | 3 |
Diffuse | 1 | 12 |
Data from Tubiana-Hulin M: Incidence, prevalence and distribution of bone mets. Bone 12:S9-S10, 1991.
Pathophysiology
Numerous tumor cells gain access to the systemic circulation, primarily through the capillary system, but some gain access through the lymphatics; only a few of these cells are able to establish a metastatic focus successfully.18
Cancer cells metastasize to bone mostly via hematogenous spread. Skeletal blood flow accounts for only 4% to 10% of the cardiac output,19 and some authors think that the incidence of skeletal metastases is higher than expected from the relatively low perfusion of bone. A mechanism explaining the high incidence has been described by Weiss.20 The microstructure of the hematopoietic marrow renders it particularly vulnerable to tumor cell accumulation and ultimate invasion. Nutrient arteries to the bone tend to subdivide into capillaries as they near the endosteal margin of the bone. These capillaries become continuous with a rich venous sinusoidal system, with a capacity six to eight times that of the osseous arterial system. More important, the circulation comes to a near standstill at this point, allowing tumor cells more time to invade the matrix.
To sustain growth, a colony of tumor cells needs to obtain its own vascular supply once it has been established. A hypothesis is that a tumor angiogenesis factor attracts vessels to a small tumor colony that would otherwise be dependent on local tissue circulation and incapable of further invasion.21 The production of such a tumor angiogenesis factor may be partly blocked by the immune responses, presumably mediated through lymphocytes. Therefore an established micrometastasis may attract vasculature required for growth several years later. This theory may explain the late appearance of metastases long after definitive treatment of the primary tumor.
Some tumors, notably of the breast, prostate, lung, kidney, and thyroid gland, produce and secrete humeral mediators that stimulate osteoclast activity. These include transforming growth factor, platelet-derived growth factor, tumor necrosis factor, prostaglandins, procathepsin D, interleukins, parathyroid hormone–related protein, and granulocyte-macrophage colony-stimulating factors.22,23
The distribution of metastases in the skeletal system is not uniform. Bone metastases tend to involve the axial skeleton more often than the appendicular skeleton. Considering the equally rich hematopoietic system in the appendicular skeleton, this higher predilection argues for specific bone marrow-derived growth factors that fertilize the “soil” of the bone for tumor growth.19
Diagnosis
Laboratory Studies
Biochemical parameters include alkaline phosphatase, urinary hydroxyproline, and the urinary hydroxyl-proline-creatinine ratio. These lack specificity, and are of no value in the diagnosis of skeletal metastases.24,25
Imaging Studies
Skeletal scintigraphy is usually the first-line imaging technique used for detecting skeletal metastases. A bone scan is more sensitive than plain radiographs and has the advantage of examining the entire skeleton. Most lesions evoke an osteoblastic response, which shows up as an increased tracer uptake.26 Occasionally, metastases may show up as areas of decreased uptake. This may be observed in rapidly growing lesions, when bone destruction far exceeds new bone formation, or secondary to an infarction. Highly vascular metastases, such as those from a renal primary cancer, may be seen on the early vascular phase of the bone scan. Metastases not detected by a bone scan include tumors that do not evoke an osteoblastic response, such as myeloma, some lymphomas, and very small deposits.27
Widespread metastatic disease may be misinterpreted as a normal scan with symmetric uptake. In these situations, a reduction in the urinary excretion of the isotope and faint or absent renal uptake with decreased bladder activity are clues of an abnormal scan.28
Most skeletal metastases develop in the medulla and involve the cortex late; therefore plain radiographs are generally insensitive.28 Within the spine, the vertebral body is affected first, although the radiologic findings of pedicle destruction are noted first.29
Computed tomography (CT) scanning has been found to differentiate between metastases and degenerative joint disease, even though the two coexist, and the latter is a common cause of increased uptake on a bone scan. Muindi and colleagues30 reported that 50% of patients with breast cancer who have a positive bone scan and a normal radiograph had obvious skeletal metastases on a CT scan; 25% had a benign cause and 25% had a negative CT scan. None of the patients with a CT scan that was negative for metastases subsequently experienced metastases. CT scan is also valuable in evaluating soft tissue involvement and can be combined with myelography for detecting extradural tumor spread in patients unable to undergo MRI.
MRI has recently been described as the method of choice for examining the spine. It is more sensitive than a bone scan for detecting early metastases within the medulla, but both T1- and T2-weighted images are required.31 It is the procedure of choice when neural compression is suspected,31 because it is less invasive than CT myelography, and a small incidence of acute deterioration of neurologic function has been reported by CT myelography.32 When cord impingement is suspected, imaging of the entire spine should be considered because approximately 10% of patients have multiple levels of cord impingement.33 It is also used in discriminating between benign and malignant vertebral collapse. In the future, whole body MRI could emerge for metastasis screening.34 Disadvantages of an MRI include its high cost, exclusion of patients with metal implants, those with severe claustrophobia, and inferior visualization of the cortex compared with a CT scan.
Positron emission tomography (PET) with 18F-fluoride or 2-fluoro-deoxy-D-glucose (FDG) is used for the initial staging of many cancers and is helpful in the diagnosis of bony metastasis. 18F-fluoride is a bone imaging agent and forms fluoroapatite in osteoblastic cells. Uptake is higher than for the technetium-99m (99mTc) used for bone scintigraphy.35 FDG is a tumor imaging agent that exploits the higher glycolysis activity in the tumor cells.36 FDG-PET scan compared with bone scintigraphy shows a similarly high sensitivity (range from 74% to 95%) but a higher specificity (range of 90% to 97%).37–41 Limitations include traumatic, infectious, and inflammatory processes that can also accumulate glucose. Accumulation of FDG requires the tumor to have an adequate metabolic rate, and neoplasms such as prostate adenocarcinoma are not consistently seen with PET scans.42,43 PET images provide poor anatomic imaging and are most useful when employed with a fused CT or MRI image.35,44,45
Treatment
Treatment recommendations must be individualized. A key consideration is the patient’s overall prognosis. This assessment should be based on an understanding of the natural course of the specific disease. Although the survival of patients with bone metastases is generally poor, potential long-term survivors must be identified. Not only would they require more durable relief, but they are also more at risk for a late, treatment-related complication. In the Radiation Therapy Oncology Group (RTOG) trial,46 median survival times in patients with solitary and multiple bone metastases were 36 weeks and 24 weeks, respectively. Patients with breast and prostate primary tumors survived significantly longer (30 to 73 weeks), whereas patients with lung cancer died within a median of 12 to 14 weeks. Patients with renal cell carcinoma with solitary metastasis are also likely to be long-term survivors. Mogens47 monitored 25 such patients for 10 to 14 years. The median survival time was 4.3 years; the 5-year survival rate was 36%; and the 10-year survival rate was 16%.
Pharmacologic Treatment
Bisphosphonates
Four phase II trials of intravenous pamidronate every 2 to 4 weeks as the sole treatment of osteolytic bone metastases in patients with breast cancer reported similar results.48–51 Relief of pain was noted in approximately 50% of patients, and approximately 25% showed radiographic evidence of bone healing. Similar results for bone pain have also been reported in patients with prostate cancer.
In more recent studies, one phase II52 and one phase III53 trial showed equivalence between zoledronic acid and pamidronate. Rosen and colleagues53 conducted a three-arm study in 1648 patients with bone lytic or mixed disease from either breast cancer or multiple myeloma. Patients received intravenous pamidronate (90 mg) or zoledronate (4 mg or 8 mg) every 3 weeks for 13 months. The primary endpoint was the incidence of a skeletal event and secondary endpoints were pain relief and performance status (Eastern Cooperative Oncology Group [ECOG] trial). All treatment groups showed equivalence with a skeletal event at 12 months and pain scores decreased by an average of 0.5 on a scale of 1 to 5. This randomized trial led to modification of the American Society of Clinical Oncology (ASCO) 2003 and the Cochrane Breast Cancer Review Group update recommendations on the use of bisphosphonates in breast cancer.54,55 Both boards now recommend either pamidronate 90 mg intravenously over 2 hours or zoledronic acid 4 mg intravenously over 15 minutes for patients with an abnormal bone scan and abnormal imaging by plain radiographs on CT scan or MRI. There is no current evidence to treat asymptomatic patients with an abnormal bone scan.
Zoledronic acid has also been used in patients with prostate cancer to treat blastic metastases.56 Saad and associates56 randomized 643 patients to placebo or to zoledronic acid 4 or 8 mg by intravenous infusion every 3 weeks for 15 months. Results show a reduction in skeletal-related events from 44% to 33%, with a significant p value of .021. Pathologic fractures were reduced from 22% to 13% (p = .015). Onset of the events occurred at a median time of longer than 420 days (median not reached) in the group receiving zoledronic acid and at 321 days in the placebo group. Time to disease progression or survival was similar in both groups. The need for local field irradiation was not significantly different in the two groups.
Zoledronic acid has also been evaluated for the treatment of bone metastases from other disease sites; 773 patients with lung, renal, head and neck, thyroid, and unknown primary tumors received either a placebo or zoledronic acid 4 or 8 mg.57 Skeletal events, including hypercalcemia, were significantly reduced from 47% to 38% (p = .039), and the median time to the first event was slower in the zoledronic acid group (225 days vs. 155 days; p = .023).
Although zoledronic acid is well tolerated, the treating physician is advised to monitor the serum creatinine before each administration. Caution is also advised for patients receiving concomitant aminoglycoside or a loop diuretic because of an increased risk of hypocalcemia. Ruggiero and colleagues58 published a retrospective review of 63 patients taking bisphosphonates who suffered osteonecrosis of the jaw; 57% received pamidronate and 21% zoledronic acid. Surgical treatment was required. Oral agents such as clodronate have low bioavailability (2%) and produce gastrointestinal side effects.
Analgesics
The optimal management of pain begins with careful assessment of the degree of pain, the site, functional limitations, and concurrent neurologic symptoms.59 The World Health Organization (WHO) analgesic ladder for cancer pain management provides a good guideline for analgesic use.60
Attention should be paid to selecting the appropriate analgesic, dose, route, and schedule. Continuous, slow-release medications are generally preferred over short-acting medications. The latter can be used effectively for breakthrough pain. Allowing pain to recur between doses causes unnecessary suffering and may allow tolerance to develop. When prescribing oral opioids, the dosage is about two times that of the subcutaneous dose and three times that of the intravenous dose. For patients unable to take oral medications, suppositories and transdermal patches are good options. When combining drugs, it is important to use drugs that act at different levels of the pain pathway (Fig. 23-1). The combined effect can be additive and at times synergistic.
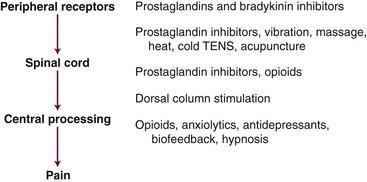
Figure 23-1 Pain pathway and analgesia interventions. TENS, transcutaneous electrical nerve stimulation.
The pain of bone metastases is generally only partially responsive to opioids.61 Many osseous metastases produce prostaglandins that induce osteolysis. NSAIDs alleviate pain by inhibiting the synthesis of prostaglandins. Corticosteroids prevent the formation of arachidonic acid (the precursor of prostaglandins) from cell membrane phospholipids. The use of NSAIDs or corticosteroids combined with morphine is usually effective.
Surgical Treatment
Surgery should be considered for patients with pathologic fractures or impending fractures (Fig. 23-2). In the former situation, fixation can reduce pain and expedite healing. In the latter, prophylactic fixation may prevent a fracture, thereby eliminating the functional loss and reducing the risk of nonunion of a fracture.
To understand the role of surgery better, we need first to elaborate on the biomechanics of pathologic fractures. Cortical defects weaken bone, especially in the setting of torsional stress. The two general categories of cortical defects are (1) stress riser, a defect with dimensions less than the diameter of the bone, and (2) open-section defect, a discontinuity of dimensions greater than the diameter of the bone.62 By creating a nonuniform distribution of stresses in bone, stress risers can decrease bone strength by 60% to 70%.63 An open-section defect has a greater impact on decreasing shear and torque-loading resistance. The volume of bone able to resist the load is significantly decreased compared with a closed section. A 90% reduction in load to failure and energy storage to failure is noted in torsion testing of the human adult tibia with open section.64 Torsional or rotational forces occur in various daily movements such as getting out of a chair. Bone is weakest during torsion. A single quarter-inch hole made for a bone biopsy can decrease torsional strength by 50%.65
The nature of the metastatic lesion affects the overall bone strength. Both lytic and blastic lesions dramatically alter bone elasticity; lytic lesions reduce bone strength more than blastic lesions. Irregular lesions are not necessarily more detrimental to the bone than smooth lesions, but elongated lesions drastically reduce bone strength.66
Fidler66 described a technique for estimating the percentage of cortical bone involvement at any level of a long bone using anterior-posterior (AP) and lateral films. This technique is thought to be as accurate a prognostic indicator as cross-sectional CT images, except when cortical destruction progresses in a patchy or spiral form.62
The distribution of pathologic fractures is shown in Table 23-4. Several series have examined various criteria predicting the risk of a pathologic fracture. Keene and associates68 evaluated 2673 breast cancer patients in an attempt to predict pathologic fracture of the femur using clinical and radiologic criteria. Only 26 (13%) of 203 patients with evaluable proximal femur metastasis had pathologic fractures. They were unable to correlate lesion size and risk of pathologic fracture. No other risk factor was identified. The authors concluded that plain radiographs are insufficient diagnostic tools for identifying high-risk lesions. Of note is that this study was limited to single AP films.
Location | No. | % |
---|---|---|
Femur | 258 | 65 |
Femoral neck | 69 | 17 |
Peritrochanteric site | 50 | 13 |
Subtrochanteric site | 84 | 21 |
Femoral shaft | 38 | 10 |
Supracondylar site | 17 | 4 |
Acetabulum | 34 | 8.5 |
Tibia | 31 | 7.5 |
Humerus | 68 | 17 |
Forearm | 8 | 2 |
Total | 399 | 100 |
From Mirels H: Metastatic disease in long bones. A proposed scoring system. Clin Orthop Relat Res 249:256, 1989.
Mirels69 designed a score system to predict the risk of a pathologic fracture (Tables 23-5 and 23-6). Of 78 patients, 51 experienced a fracture and 27 did not. The mean score for the nonfracture group was 7 versus 10 for the fracture group. This system provides a useful tool to evaluate patients for prophylactic fixation. Patients with a score of 10 to 12 should undergo surgery. Patients with scores of 7 or less are not likely to benefit from such therapy. In patients with a “gray zone” score, the status of surrounding bone and lifestyle (elderly osteoporotic woman vs. young athlete) should be considered.
TABLE 23-6 Pathologic Fracture Rate
Score | No. Patients | Fracture Rate (%) |
---|---|---|
0-6 | 11 | 0 |
7 | 19 | 5 |
8 | 12 | 33 |
9 | 7 | 57 |
10-12 | 18 | 100 |
From Mirels H: Metastatic disease in long bones. A proposed scoring system. Clin Orthop Relat Res 249:256, 1989.
The following principles govern the surgery of impending fractures:
Pathologic fractures of the humerus commonly occur in the diaphysis followed by the proximal humerus. Fractures of the diaphysis can be fixed using an intramedullary interlocking device, such as a Brooker-Wills nail, which provides excellent strength and effective resistance against varus, torque, and distraction forces.70 Proximal humerus fractures commonly require prosthesis. These patients usually achieve a limited flexion and abduction of about 90 to 100 degrees and enjoy good overall function, joint stability, and pain relief.62
Fixation of the femoral neck-intertrochanteric area can be achieved with the use of a compression hip screw and side plate. Fractures involving the femoral neck may be better treated with prosthetic replacement because they are rarely amenable to internal fixation.71 Subtrochanteric, femoral shaft, and supracondylar femoral lesions are amenable to internal fixation, but large cortical lesions may benefit from an intramedullary acrylic cement filling.
An aggressive surgical approach to spinal metastases is usually not warranted.72 Spinal stabilization is a major operation involving multiple risks and prolonged recovery. Most patients with spinal metastases do not have progressive spinal instability or neurologic involvement and can be treated with irradiation, hormone therapy, chemotherapy, or temporary bracing. Even patients with vertebral body compression fractures can be treated with temporary bed rest and soft bracing. Indications for surgical intervention include (1) progressive spinal canal impingement and cord compression by a radioresistant tumor or a recurrence after the maximum tolerance dose to the intended area has been given; (2) bone or soft tissue detritus extruded into the canal as a result of progressive spinal deformity, with or without spinal instability; (3) progressive spinal deformity; (4) progressive kyphotic deformity associated with posterior disruption and shear deformity; and (5) solitary metastases of a histologic type that is unlikely to be controlled over the long term with tolerable doses of irradiation.
Vertebroplasty of bone metastasis was first described in 1987 and consists of direct injection of the affected vertebra with cement. Polymethylmethacrylate (PMMA) is active through several pathways and produces pain relief in 80% of patients.73 The procedure is done under intravenous sedation or general anesthesia. Pain receptor destruction is achieved with exothermic reaction of the polymonomer and compressive effect on small nerves. Vertebroplasty effects are not modified by external beam radiation therapy, and PMMA conserves its properties despite irradiation.73 Both treatments could become complementary measures in the future.
Radiotherapy
Local-Field Radiotherapy
The vast majority of patients can be managed successfully with external beam radiotherapy (EBRT). A large body of clinical evidence documents the effectiveness of such therapy.74 The optimal dose and fractionation schedule are still not resolved. A summary of the major prospective clinical trials that addressed these issues is provided in Table 23-7. The results of these studies should be interpreted with caution because the inherent heterogeneity within the randomization groups may have precluded detection of significant differences, even when such differences could have existed. The use of different pain scoring systems (physician based vs. patient based) and different handling of concomitant use of analgesics, chemotherapy, or hormonal therapy preclude meaningful comparison of the results of these studies.
Between 1974 and 1980, the RTOG conducted a large national study to determine the effectiveness of five different dose fractionation schedules.46 A total of 1016 patients were entered, 266 into a solitary metastasis stratum and 750 into a multiple metastasis stratum. The former were randomly assigned to treatment with 40.5 Gy in 15 fractions or 20 Gy in 5 fractions. The latter were assigned to 30 Gy in 10 fractions, 15 Gy in 5 fractions, 20 Gy in 5 fractions, or 25 Gy in 5 fractions. A quantitative measure of pain, based on severity and frequency of pain and the type and frequency of pain medications used, was devised to evaluate response. Overall, 89% of patients experienced minimal relief, whereas 83% achieved partial relief and 54% obtained complete relief. There were no significant differences between the treatment arms in both strata. The initial pain score was found to be a useful predictor; patients with high scores were less likely to respond and less likely to experience a complete response. Patients with breast and prostate cancer were significantly more likely to respond than those with lung or other primary lesions. Patients completing their treatment as planned had a significantly higher rate of complete response than those who did not. Although some pain relief was experienced almost invariably within the first 4 weeks, complete relief was first reported later than 4 weeks after the start of treatment in about 50% of patients. The median duration of minimal and complete pain relief was 20 and 12 weeks, respectively. There were no significant differences in the duration of pain relief between the different arms. The authors concluded that all treatment dose schedules were equally effective.
Blitzer75 performed a reanalysis of the RTOG study. Using a stepwise logistic regression, he examined the effect of the number of fractions, the dose per fraction, and the presence of solitary versus multiple metastases on the probability of attaining complete pain relief and the need for retreatment. This multivariate technique allowed patients with solitary and multiple metastases to be analyzed together. By increasing the number of patients and events, the statistical power of the analysis was increased. The number of fractions was the only variable that was significantly associated with outcome. There was no correlation of the time-dose factor (TDF)76 with outcome. It was concluded that the more protracted schedules resulted in improved pain relief.
The concept of TDF has long been replaced by the linear quadratic model. Using this model, and assuming an α/β of 10 for tumor, the biologically effective dose (BED) was calculated for the various schedules tested by the RTOG. Figures 23-3 and 23-4 depict pain response and freedom from retreatment as a function of BED, respectively. The solid lines are the regression functions, and the dotted lines represent the 95% confidence intervals. The results suggest that schedules with a higher BED resulted in better pain relief and reduced the need for retreatment.
Price and colleagues77 randomized 288 patients to receive either 8 Gy in 1 fraction or 30 Gy in 10 daily fractions. Pain was assessed using a daily questionnaire completed by the patient at home. No differences were found in the probability of attaining pain relief, speed of onset, or duration of relief between the two arms.
Hoskin and colleagues78 randomized 270 patients to receive either 4 Gy or 8 Gy in one fraction. Pain (assessed by the patient) and analgesic usage were recorded before treatment and at 2, 4, 8, and 12 weeks. At 4 weeks, the response rates were 69% for 8 Gy and 44% for 4 Gy (p <.001). The duration of the effect was independent of dose.
Additional studies have evaluated single-fraction versus multiple-fraction regimens. A Danish randomized trial of 241 patients showed no significant difference with regard to pain relief or quality of life after receiving either 8 Gy in a single fraction or 20 Gy in 5 fractions.79 Wu and colleagues80 performed a meta-analysis of 16 trials including 5455 patients and proclaimed equivalence between single and multiple fractions. van der Linden and colleagues81 recently published a re-analysis of a Dutch Bone Metastasis Study that included 1171 patients. This study randomized patients to either a single dose of 8 Gy or to 24 Gy in 6 fractions. This new publication focuses on retreatment need. Mean time to retreatment was shorter (13 weeks vs. 21 weeks) with a single fraction. It was also more frequent (24% after a single fraction and 6% after 6 fractions; p = .001). An initial high pain score also influenced the need for retreatment.
Hemibody Irradiation
Most patients with bone metastases have multiple sites of involvement. As many as 76% of patients receiving therapy to a local field require additional treatment for pain at other sites within 1 year.82 Historically, wide-field radiotherapy was used to address this problem. Although large-field irradiation is less commonly used in the era of systemic therapy, it remains a treatment option. A summary of results of this form of therapy is provided in Table 23-8. Response rates are similar to those observed with local-field radiotherapy, but the onset of relief is more rapid, occurring often within 24 hours of treatment. Hemibody irradiation (HBI) may require hospitalization for hydration and premedication with steroids and antiemetics and tends to be associated with substantial morbidity. Most patients experience acute gastrointestinal toxicity, with nausea, vomiting, and diarrhea persisting for 24 to 48 hours. Myelosuppression is commonly observed but is rarely of clinical significance. Radiation pneumonitis is rare at doses below 7 Gy to the lungs.
The RTOG conducted a phase III study to evaluate the efficacy of HBI in addition to local-field irradiation.82 A total of 499 patients were randomized to receive either HBI or no further therapy after completion of local-field irradiation to a symptomatic site. Entry was stratified by extent of metastatic disease (solitary or multiple) and the targeted hemibody area (upper, middle, or lower). Local-field irradiation consisted of 30 Gy in 10 fractions, and HBI consisted of 8 Gy in 1 fraction given within 7 days of completion of the local field. Partial transmission blocks were used to reduce the dose to the lungs to 7 Gy. Time to disease progression, time to new disease, and time to new course of therapy were significantly longer in the HBI arm. Progression of disease was faster in patients with involvement of the upper and middle hemibody (compared with the lower hemibody) and in patients with multiple metastases (compared with a solitary tumor). As expected, toxicity was significantly higher in the HBI arm, but there were no fatalities and no occurrences of radiation pneumonitis. Although an impact of HBI on clinically occult metastatic disease was demonstrated, the long-term benefit was relatively small. The ultimate progression rates were not significantly different between the arms, and at 1 year 60% of the HBI patients had to be retreated.
Stereotactic Radiosurgery of the Spine
A recent review offers a comprehensive analysis of outcomes and toxicity after spinal SBRT and SRS.57 Generally, pain control and local tumor control is on the order of 85%.83,84–88 There appears to be a dose response; greater palliation is seen with prescribed isocenter doses of 14 Gy or more.89 Although traditional palliative spine radiation fields encompass the involved vertebral body plus a vertebral body above and below, SRS and SBRT generally treat only the involved vertebral body or a portion of the involved vertebral body. In a study from Henry Ford Hospital (Detroit), the development of subsequent metastases to adjoining vertebral bodies after SRS was rare (~5%) and associated with progression of disease elsewhere.85 In a study from the M.D. Anderson Cancer Center (Houston), in-field failures occurred in approximately 25% of recurrences, whereas roughly half of the recurrences were in the epidural space, which was attributed to underdosing of this region to maintain spinal cord dose constraints. Patients also failed in other regions not included in the treatment volume (of any given patient), including the pedicles, posterior elements, and prevertebral and paravertebral regions.83
Recent clinical data have shown that SRS and SBRT treatment of the spine is tolerable, albeit with limited patient follow-up90 because patients with spinal metastases generally have a poor survival rate, even those with a solitary spinal metastasis.91 It appears that myelopathy and radiculopathy rarely occur.57,90 For single-fraction SRS, most institutions try to achieve a spinal cord maximum dose below 10 Gy.57 In the University of Pittsburgh experience of spinal SRS in 393 patients with 500 lesions, for which the cord maximum was kept below 8 Gy, no acute or late neurotoxicity was observed, and no late toxicity was reported after a follow-up of 3 to 53 months (median, 21 months).84 Several institutions have demonstrated that spinal cord maximum doses of 12 Gy86 to 20 Gy90,92 are tolerated in some patients, though from a recent multi-institutional pooled analysis, radiation myelopathy has only been documented to occur after a fractional dose maximum of 10 Gy to the spinal cord has been exceeded.93 In the pooled-analysis study, dose-volume parameters such as maximal dose and mean and median dose to 0.1 to 5 mL of the spinal cord significantly correlated with the risk of radiation myelopathy. In the ongoing RTOG 0631 study (which is a randomization of spinal SRS delivered with 16 Gy versus conventional irradiation delivered in one fraction of 8 Gy), spinal cord dose constraints are 10% and 0.35 mL of spinal cord less than 10 Gy, and 0.035 mL less than 14 Gy, with the spinal cord volume defined as 5 to 6 mm above and below the target, based upon T2- and T1-weighted MRI.
Systemic Radionuclide Therapy
The first report on the use of systemic radionuclides for the treatment of bone metastases was published by Pecher94 more than 50 years ago. Using this modality, all involved osseous sites can be addressed simultaneously. Selective absorption into bone metastases limits irradiation of normal tissues and increases the therapeutic ratio. Administration as a single intravenous injection in the outpatient clinic is a further advantage for many patients.
Systemic radionuclides should be considered in the following circumstances:
Historically, phosphorus-32 (32P) was the first radionuclide to be widely used in the treatment of bone metastases,95 although this isotope is now rarely used for bone disease due in part to myelosuppression with pancytopenia and an increased incidence of acute leukemia.82 Phosphorus-32 has since been replaced by newer, less toxic radionuclides (Tables 23-9 and 23-10).
Strontium-89
Strontium-89 (89Sr) decays by beta emission to yttrium-89 (89Y) with a half-life of 50.6 days. The average beta energy is 1.46 MeV. Chemically similar to calcium, 89Sr is quickly taken up into the mineral matrix of bone. The fraction of 89Sr retained is proportional to the metastatic tumor burden and varies between 20% and 80% of the administered dose.96 Accumulation is preferred in and around metastatic deposits, where active bone formation takes place, which is likely to be adjacent to but not in the site of the malignant tumor.96,97,98 Once incorporated into the metastatic lesion, 89Sr is not removed metabolically and remains deposited for as long as 100 days.96 Accurate tumor dosimetry is very difficult and usually based primarily on the location of tracer accumulation. Estimates of the total dose absorbed within the metastatic lesion vary between 0.9 and 231 cGy per megabecquerel (MBq), with the typical mean total dose at 23 cGy/MBq and high doses usually corresponding to superscans.97,98,99,100 Typical doses are 1.5 MBq/kg, leading to a nominal tumor dose of 20 to 25 Gy. Elimination is through the kidneys, and careful disposal of urine is needed for 7 to 10 days after administration. Extra care is advised for incontinent patients. Because 89Sr emits extremely little gamma radiation, the patient is not a radiation hazard to family members or hospital staff.
The efficacy of 89Sr has been well documented in dose-seeking studies.96,100–106 Laing and associates103 reported on the results of a dose escalation study. The optimal dose was found to be 1.5 MBq/kg with no appreciable increment in efficacy above this dose. Of 83 patients treated with at least 1.5 MBq/kg, 75% had partial relief of pain and 22% were rendered pain free. Pain relief began 10 to 20 days after treatment and peaked at 6 weeks. Response was maintained for a median of 6 months (range, 4 to 15 months). The RTOG conducted a dose escalation study and concluded that the maximum tolerated dose of 89Sr is 6.5 mCi (≈3.4 MBq/kg).
Toxicity of 89Sr is mainly hematologic. Platelet depression is dose dependent and can be prolonged. Most patients have a 20% to 50% drop in their counts after doses of 3 to 4 mCi (1.5 to 2 MBq/kg). Grade III toxicity is rare. Other adverse effects include a transient increase in bone pain in up to 10% of patients and, rarely, facial flushing. The pain flare occurs 1 to 2 weeks after treatment, may last a few days, and usually heralds a favorable response.
Porter and colleagues107 reported the results of the Trans-Canada study. This trial evaluated the efficacy of 89Sr adjuvant to local-field EBRT in patients with hormone-refractory prostate cancer. A total of 126 patients were randomized to local-field EBRT (20 Gy in 5 fractions or 30 Gy in 10 fractions) followed by placebo or by 89Sr (10.8 mCi). Overall and complete responses (relief of pain at the index site) were higher in the treatment arm, but the differences did not reach statistical significance. At 3 months after treatment, 58.7% and 34% of patients in the treatment arm and control arm, respectively, were free of new painful metastases. The median time to further radiotherapy was 35.3 weeks and 20.3 weeks in the treatment and control arms, respectively. Hematologic toxicity was, as expected, higher in 89Sr-treated patients.
Samarium-153
Samarium-153 (153Sm) is a artificial radionuclide that emits beta particles of 0.81 MeV (20%), 0.71 MeV (30%), and 0.64 MeV (50%) and gamma photons of 103 keV (28%). It has a relatively short half-life of 46.3 hours and, consequently, a relatively high dose rate. Samarium-153 has been chelated to a phosphonate, ethylenediaminetetramethylene (EDTMP), to produce a bone-seeking complex. About 50% of an intravenously administered dose is retained in bone.108,109 Absorbed dose in bone and red marrow has been estimated at 2.5 cGy/MBq and 0.57 cGy/MBq, respectively.109 Clinical experience with 153Sm is still limited. In a phase I/II clinical trial,110 the maximally tolerated dose (MTD) was determined to be 2.5 mCi/kg. The principal toxicity observed was hematologic; maximum myelosuppression occurred at 3 to 4 weeks. A flare of bone pain occurred in 12% of patients. The overall pain relief rate was 74%, with a median duration of palliation of 2.6 months. In responders, relief was obtained promptly within 7 to 14 days of treatment. Response rates were significantly higher with 2.5 mCi/kg than with 1 mCi/kg.
Rhenium-186
Rhenium-186 (186Re) emits beta particles of 1.07 MeV and a 137-keV gamma ray and has a short half-life of 3.8 days. Like 153Sm, it has been complexed to a bone-seeking phosphonate, hydroxyethylenediphosphonic acid (HEDP). Retention in bone is about 50% of the injected dose; the rest is excreted through the kidneys into the urine.111 Rhenium-186 has been studied in a small number of patients with metastatic cancer of the prostate, breast, colon, and lung.112 After administration of 33 to 35 mCi, 75% to 80% of patients experienced pain relief, most often within 2 weeks.111–113 The therapeutic efficacy of 186Re has been confirmed in a double-blind, crossover comparison with placebo.113 Myelosuppression begins 2 weeks after treatment, peaks at 4 to 6 weeks, and resolves by 8 weeks.112 A pain flare occurs in 10% of patients 2 to 3 days after treatment and resolves within 1 week.
Choice of Radiotherapeutic Approaches
In the United States, the usual approach to painful osseous metastases includes fractionated EBRT to index painful sites. Asymptomatic sites are less commonly irradiated. Patients with multiple lesions will commonly receive radionuclides in addition to EBRT to cytoreduce occult metastases and reduce the need for future local irradiation. Radionuclides alone and HBI are less common in the United States, although the latter is often used in Europe and Canada. The advent of improved EBRT targeting technologies and the earlier discovery of patients with limited metastases have spawned efforts for local targeting of bone metastases. The utility of these high-dose treatments to achieve improved tumor control with reduced marrow and other toxicity is under investigation.114
Spinal Cord Compression
Malignant spinal cord compression occurs in 5% of all patients with malignant disease and in approximately 20% of patients with metastases to the vertebral column.115 More than 95% of spinal cord compressions are due to extramedullary malignant disease, most commonly secondary to involvement of the vertebral column anterior to the spinal cord, less frequently by tumors posterior to the spinal cord, and occasionally by invasion of the epidural space. Thankfully, the frequency of severe spinal cord compressions leading to paralysis and incontinence is decreasing with the availability of high-quality MRI technologies.116
Clinical Manifestations and Patient Evaluation
The majority of patients present with pain, motor loss, autonomic dysfunction, and sensory loss.117 Pain is often radicular for weeks or months before the onset of neurologic symptoms, offering ample time for early diagnosis. Autonomic dysfunction may occur early and manifest as hesitancy and urgency. Weakness usually precedes sensory loss; incontinence, paraplegia, and paralysis are late effects. Pain was the initial symptom in 96% of the patients but is a poor indicator of spinal-epidural involvement.118 In contrast, 75% of patients with major neurologic involvement have involvement of the epidural space.
Diagnosis
Early diagnosis is essential because recovery of neurologic function is related to the degree of loss. A careful history and physical examination focusing on neurologic assessment with a high index of suspicion in patients with known cancer is key to early diagnosis. MRI is the diagnostic study of choice. The entire length of the spinal cord and its relationship with surrounding structures can be visualized without the use of intrathecal contrast media.119,120 Distortion of the theca by extradural lesions and soft tissue abnormalities can be easily identified.118,121 In case of compression fracture, protrusion of the vertebral body or tumor into the spinal canal is seen clearly, as is the impingement of nerve roots and neural foramina.119 Approximately 10% of patients have multiple sites of cord compression and perhaps benefit from imaging of the entire spine.
Treatment
A randomized trial comparing laminectomy followed by radiation versus radiation alone in the treatment of spinal epidural metastasis showed no significant difference in the effectiveness of treatment in regard to pain relief, improved ambulation, and improved sphincter function.122 Although most cases of spinal cord compression can be managed with steroids and radiotherapy, patients without a histologically proven cancer, radioresistant tumors, previously radiated sites, or mechanical instability should be seen by a neurosurgeon for a laminectomy (Fig. 23-5).
In a multi-institutional randomized study123 of patients with symptomatic spinal cord compression from vertebral metastases, 50 patients were randomized to receive decompressive surgery followed by radiation and 51 were randomized to receive radiation alone (30 Gy in 3-Gy fractions for both arms). The study was closed early due to the superiority in outcomes of surgery plus radiation versus radiation alone. Following surgery and radiation (vs. radiation alone), significantly more patients were able to walk, patients retained the ability to walk significantly longer, significantly more patients regained the ability to walk, and the need for corticosteroids and analgesics was significantly reduced. Patients undergoing surgery also had a significantly longer survival time. Table 23-11 summarizes the results from this landmark study.
Treatment outcome is dependent on pretreatment function. In a study of 137 patients with malignant spinal cord compression, 81% of patients who were ambulatory before treatment remained ambulant, whereas only 16.5% of those who were nonambulatory before treatment became ambulant after treatment. Pain improved after treatment in 73% of the patients regardless of their ambulatory status.115 This was confirmed by Zelefsky and colleagues124 in a retrospective review of 42 patients. They also reported that the presence of a compression fracture of greater than 50% at the level of the spinal cord compression was associated with poor response on refluoromyelography (RFM). In patients with severe compression fractures, 67% had no response on RFM versus 11% without compression fracture (p = .01).
Irradiation Techniques and Doses
Treatment fields are dependent on the site of involved spinal cord. The cervical spine is usually treated using opposed laterals to avoid the oral cavity (see web-only Fig. 23-1, available on the Expert Consult website). For the thoracic spine, a posterior field alone can be used. When treating the lumbar spine or when the target appears to be more at the midline, a parallel opposed AP/PA beam arrangement may be preferred. For either the thoracic or lumbar spine, acceptable alternative techniques include paired posterior obliques or PA and paired lateral fields.
Brain Metastases
Secondary brain tumors are a common complication of systemic cancer. The incidence ranges from 20% to 40% of all patients diagnosed with cancer.125 Cancers known to generate systemic disease are the most common primary tumors involved: cancers of the lung, breast, and colon and melanoma. Current improvements in systemic therapy have improved survival significantly for several cancers but may leave untreated tumor cells beyond the blood-brain barrier. More precise diagnostic tools, such as MRI, also have an impact on the increasing discovery of brain metastases.
The prognosis of brain metastases is poor, and the impact on the patient’s quality of life is important due to the functional neurologic deficits associated. Symptom management is successful in most patients and efforts can be concentrated on improving the outcome of the patients.125
Arterial hematogenous spread results in tumor emboli growth at the gray-white junction126; the most common neuroanatomic sites are the cerebral hemispheres (80%), the cerebellum (15%), and the brainstem (5%).127 Multiple metastases are more common than a single metastasis; the contribution of MRI to this statistic has reached 80% to 90%.125
Contrast-enhanced MRI is the diagnostic modality of choice. The radiologic differential diagnosis includes primary brain tumor, inflammatory lesion, abscess, and brain infarction or hemorrhage. CT may also be used, but it is less specific and warrants MRI confirmation in the case of a single metastasis. Patchell and colleagues128 reported a false-positive rate with MRI of 11%, confirmed by histology.
Treatment
Palliative treatment of brain metastases requires rapid control of the symptoms that are decreasing the patient’s quality of life. Collaboration with colleagues in neurology is preferable. Pharmacologic treatment includes corticosteroids and antiepileptic drugs, although antiepileptic drugs should not be used prophylactically in patients without prior seizure.129 Rapid regression of cerebral edema is the first step and can be achieved with intravenous corticosteroids. Optimal dosage is unknown, but the general practice is to administer a loading dose of dexamethasone (Decadron) (8 to 32 mg) followed by oral medication (4 mg four times a day).130 Side effects are numerous, and a tapering dose schedule should be planned as symptoms improve. As a single modality, corticosteroids achieve poor survival results of 1 to 3 months.
Treatment depends on several prognostic factors; Gaspar and associates 131 have evaluated results of RTOG trials to produce a recursive partitioning analysis. Pretreatment- and treatment-related variables were analyzed. Class 1 (Karnofsky performance status [KPS] score >70, age <65 years, and controlled primary tumor) patients have a better median prognosis of 7 months. Class 2 (KPS score <70, age >65 years, or uncontrolled primary tumor) patients have a median prognosis of 4 months. Class 3 (KPS score <70, age >65 years, and uncontrolled primary tumor) patients have a median of 2 months. Other factors, such as histology of the tumor and the number and size of metastases, are important in the initial evaluation. Treatment options are evolving and now include whole-brain radiotherapy (WBRT), surgical resection, and radiosurgery (by means of the linear accelerator or gamma knife).
Whole-Brain Radiotherapy
Whole-brain radiotherapy (WBRT) is the treatment of choice for many patients because of the high incidence of multiple metastatic sites or uncontrolled primary tumor.125,130,131 The goal of WBRT is to limit tumor progression and, in the middle term, to limit the use of corticosteroids. The optimal dose of irradiation is unknown, but in clinical practice, the range is 20 Gy in 5 fractions over 1 week to 40 Gy in 20 fractions over 4 weeks.125
Surgical Resection
The role of surgery has evolved over the past decade and is now part of the aggressive management of brain metastases. Three randomized controlled trials comparing WBRT alone versus surgery plus WBRT have been published.128,132,133 Although they were relatively small studies, two of them demonstrated a survival advantage of the combined modalities over WBRT alone (Table 23-12).
All three trials addressed the issue of single metastases, and one cannot extrapolate the results to multiple lesions. The negative results of Mintz133 contradict those of others, but this trial also contained a lower complete surgical resection rate and lower WBRT dose.
Radiosurgery
Stereotactic radiosurgery (SRS) is an accepted alternative to resection in patients who are medically inoperable or who have metastases that are not amenable to resection either due to the location of or number of metastatic lesions. SRS is generally offered to patients with one to three brain metastases, less than 4 cm in size, and not amenable to resection.*
The recent RTOG 9508 study randomized 333 patients with one to three brain metastases to WBRT (37.5 Gy in 2.5-Gy fractions) versus WBRT plus SRS within 1 week of completing WBRT.138 All metastases were 4 cm or less in size and only one metastasis could be more than 3 cm. The dose was dependent on the lesion size based upon the RTOG 9005 phase I study 2: 24 Gy to lesions of 2 cm or less, 18 Gy for lesions of >2 to 3 cm, and 15 Gy to lesions of ≤3 to >4 cm. In both RTOG 9005 and RTOG 9508, the prescription dose covering the gross tumor was the 50% to 90% isodose line, equating to central doses of 1.1 to 2 times the prescription dose. The RTOG 9508 trial demonstrated a significant survival advantage with the use of SRS in patients with a single unresectable metastasis, with a median survival of 4.9 months versus 6.5 months (p = .0393). The addition of SRS resulted in improved performance status and reduced extent of steroid use. The authors conclude that SRS should be used for patients with an unresectable solitary metastasis, and considered for patients with one to three metastases.
SRS alone (without WBRT) in patients with few unresectable brain metastases is an alternative approach that remains actively investigated.151,152 In retrospective studies, the 1-year local control rate is generally on the order of 80% to 95% with WBRT plus SRS,† versus 80% to 90% with SRS alone.142,148,149,150 At 2 years, the local control rate is on the order of 80% to 85% versus 50% to 70%. Therefore WBRT does lower the risk of brain failure, and the equivalence in survival likely reflects the need for more salvage therapy in the SRS-alone patients. In a study published by the University of Alabama (Birmingham), the risk of new brain metastases in 100 patients treated with SRS alone was significantly correlated to the number of brain metastases (hazard ratio [HR] of 3.3 in patients with more than three metastases; p = .004), poorly controlled extracranial disease (HR 2.16; p = .04), and melanoma histology (HR 2.14, p = .02).153 Retrospective data suggest similar local control, overall survival, and neurologic death rates with SRS alone versus resection plus WBRT.154,155 Interestingly, in some series the reported local control rate with SRS is greater than that after resection,156,157 probably reflecting the radiosurgical penumbra dose around the tumor periphery that treats microscopic disease.158
A multi-institutional, pooled retrospective analysis examined 569 patients treated with SRS alone versus SRS plus WBRT.137 Among the patients treated with SRS alone, 37% underwent salvage therapy at a median of 5.7 months after SRS versus 7% after a median of 8 months following WBRT plus SRS. This study did not differentiate between salvage for local failures and distant CNS failures. Survival rates were not significantly different (~8-month median survival rate in both arms).
In a randomized study from the M.D. Anderson Cancer Center (Houston), 58 patients were randomized to receive SRS alone versus SRS plus WBRT.159 Patients treated with SRS alone had a significantly inferior 1-year tumor control rate (67% vs. 100%; p = .012) and distant brain tumor control rate (45% vs. 73%; p = .020), but had a significantly improved 1-year survival rate (63% vs. 21%; p = .003), with an HR of more than 2 of death from neurologic causes as well as systemic causes. Postulated reasons for the improved survival rates of the SRS-only group is the earlier administration of systemic therapy as well as the high rate of salvage therapy (87%) for brain metastases.
Avoiding WBRT can potentially prevent acute and late toxicity from WBRT, and allow WBRT to be used as salvage therapy if needed. During WBRT, patients acutely experience alopecia, and may develop skin erythema and mild desquamation. Less commonly, otitis media may develop. More concerning is the late toxicity from WBRT, occurring months to years after radiation therapy, which may be relevant in the population of patients with a solitary metastasis who have a potential for cure. Late toxicity includes cataract formation, dry eye, and neurocognitive defects such as memory loss and dementia.160–162 The extent to which WBRT causes neurocognitive defects is not well reported.163 Neurocognitive decline may in part be due to the poor function of many patients who present with brain metastases and the general deterioration of patients whose cancer progresses.164,165 In a Japanese randomized study of SRS versus WBRT plus SRS, there was not a significant difference in the posttreatment change in neurocognitive function between the two study groups, although those patients in the SRS-alone group experienced a more rapid decline in neurocognitive function, presumably as a consequence of brain failure (local failure and/or distant brain failure).166 Patients treated with WBRT did experience a continued decline in neurocognitive function, due to tumor recurrence and/or effects from WBRT. In the above randomized trial from the M.D. Anderson Cancer Center, the trial was prematurely closed due to the significantly greater likelihood of decline in learning and memory function for patients undergoing WBRT plus SRS versus SRS alone.
Body Oligometastases
Hypothesis
The oligometastasis hypothesis suggests that some individuals will have only a countable number of total metastatic deposits. The hypothesis accepts that there may be additional deposits but that these are small enough to be sterilized by natural immune processes or systemic cytotoxic therapy. The term oligometastasis was coined in 1995167 but the concept predates to the earliest days of cancer treatment. As an example, during the 1950s, Philip Rubin168 wrote a text on the role of aggressive treatment for patients with solitary metastases. At that time, imaging was poor so the frequency of true solitary metastases was likely rare. The notion of oligometastases is based on the reproducible observation that greater amounts of tumor are more ominous than smaller amounts of tumor; a notion which logically extends to include metastatic disease. The need to define such a term emanates from the absence of a detailed staging system for metastatic disease. Local treatment for metastases has commonly been efficacious.
Treatment Options
Surgery
Surgery is the most commonly accepted treatment to obtain local control of primary tumors and logically has a large role to play in the local control of metastatic disease. Surgery is commonly used for sarcoma patients with lung metastases. In the pediatric population, long-term survival is seen even in patients requiring a dozen or more metastasectomies or even multiple bilateral thoracotomies.169 Efforts to control adult tumors are rarely this aggressive, in part because the available systemic therapies required to consolidate the surgery are usually lacking. If a patient has five or fewer colorectal cancer metastases to the liver (and, possibly, the lung170), however, resection is commonly done.171,172 Here, long-term survival without disease relapse is also common. The frequency of control is higher when aggressive imaging is also employed. Patients who have chemotherapy-responsive tumors have survival rates of over 25% at 3 years, even when lesions are initially unresectable due to tumor size, tumor number, or presence of nodal disease.172
Radiation Therapy
Radiation, like surgery, is commonly used to locally control primary tumors and, like surgery, has a well-established success rate at achieving permanent control. Irradiation has the advantage over surgery in cases where a field appears to have been contaminated with tumor or for which an open procedure might have unacceptable morbidity or limited potential for satisfactory excision. Radiation “metastasectomy” is becoming more common with the advent of advanced imaging and techniques for stereotactic body radiosurgery and SBRT.173,174 Many publications have now reported long-term survival rates among patients with limited metastases with no apparent subsequent disease progression.173,175,176
Other Techniques
There has been a proliferation of techniques that allow for local treatment of tumors, some invasive and some minimally invasive. Examples are hyperthermic techniques, which usually involve instrumentation of a tumor, either under visual or radiographic guidance, followed by heating using radiofrequency or ultrasound.177,178 Cryotherapy, a process similar to hyperthermia, involves freezing tissues to kill them. Vascular embolization techniques can be used as well and require cannulation of all the vessels feeding the tumor and insertion of glue to obstruct the blood supply. This procedure can be enhanced by injecting a cytotoxic agent into the tumor before the arterial occlusion. Although these techniques are effective at cytoreduction, it is not yet known which of these can achieve permanent local control.
Liver Metastases
Diagnosis
Most liver metastases are discovered with routine metastatic surveys. Biphasic and triphasic helical CT scanning is the optimal method for the detection of liver metastases. During the portal venous phase of the scan, the tumor is hypointensive due to its dependence on hepatic arterial perfusion, and tumors can be enhanced by arterial phase images. Approximately 90% of lesions greater than 1 cm are detected by portal phase images alone; approximately 10% more lesions are detected when the arterial images are used in combination.179 These imaging characteristics are also useful for distinguishing metastatic disease from many other benign small lesions commonly seen in the liver, including cysts and hemagiomas.180 An MRI can also help detect liver metastases; however, MRIs are expensive, cause motion artifacts, and have a less well defined tumor border. They are generally employed when a patient has a contraindication to a contrast CT scan. MRI can distinguish solid hepatic metastasis from fatty change, cysts, and hemangiomas. On T1-weighted images, hepatic metastases have low signal intensity, whereas on T2-weighted images, tumors have inhomogeneously high signal intensity. Other methods of tumor detection include ultrasound and incidental detection during procedures done for benign diagnoses.
Treatment
Most treatments for liver metastases are systemic. Liver metastasis can respond well to chemotherapy and hormone therapy, but most remissions are short lived. The response to these treatments can be mixed, with some tumors progressing while others subside. Aggressive local treatments for liver metastases can also provide substantial benefits. Although liver transplantation is not recommended, resection of lesions can lead to long-term survival, particularly among patients who respond to chemotherapy.171,172 Benefits for chemotherapy-responsive patients occur even among those who were initially unresectable due to nodes, number or location of lesions, and/or lesion size.172 Generally, resections are limited to patients with disease in a single lobe peripheral to the portal region. This limitation is also true for many other localized techniques, including radiofrequency ablation and cryotherapy.
Chemoablation has arisen as an effective form of therapy. In this invasive radiologic technique, the artery feeding the tumor is infused with chemotherapy, usually after an injection of contrast material defined by the tumor vasculature, followed by vascular ablation to trap the drug and asphyxiate the tumor.181–185 Chemoablation is suitable for patients with a limited number of tumors and for whom vascular access is possible.
Irradiation Techniques and Doses
Irradiation
Liver irradiation for metastatic disease can be of palliative benefit, as found in an RTOG phase II trial of 100 patients in whom palliation of pain was noted in 55%.186 Survival for more than 6 months was significantly correlated with a colorectal primary tumor, good initial performance status, and lack of extrahepatic metastases. Median survival times, however, even when these criteria were employed, were only 4 to 4.5 months.187 Addition of chemotherapy provides little if any additional benefit.188
Bydder used 5 Gy in two fractions and found improvement at 6 weeks in abdominal pain (63%), distention (30%), night sweats (63%), and nausea (44%). This abbreviated treatment provided results similar to those seen with more protracted courses, and may be recommended for patients with poor performance status.189
When partial liver irradiation is used instead of whole liver irradiation, higher EBRT doses are feasible, using three-dimensional conformal or intensity-modulated techniques. EBRT techniques and dose levels are discussed in detail in Chapter 47, Hepatobiliary Tumors, and will not be reiterated in the current chapter.
Selective Internal Radiation Therapy (SIRT)
Liver metastases can be treated with one of several embolization techniques, in which microscopic spheres (microspheres) are administered via the liver’s arterial supply. Specific techniques include “bland embolization,” in which the microspheres are not embedded with cytotoxic agents, transarterial chemotherapy embolization (TACE), in which the microspheres are embedded with chemotherapeutic agents, and selective internal radiation therapy, in which the microspheres are embedded with radioactive 90Y. It remains controversial as to which embolization technique is preferred for any given patient. Arguably, microspheres labeled with 90Y would be preferred in the treatment of chemotherapy-refractory tumors. Yttrium-90–labeled microspheres have also been used in the treatment of hepatocellular carcinoma.190
A recent paper from Northwestern University (Chicago) investigated 137 patients who underwent 227 administrations of 90Y-labeled microspheres for chemotherapy-refractory liver metastases.191 Fifty-nine percent of patients had more than four tumors. Most patients (>80%) had less than 25% of the liver involved. For all lesions in all patients, 87% experienced a biologic response. Toxicity was acceptable. Fifty-one patients had colorectal cancer; their median survival time was about 15 months. Another study from Northwestern University demonstrated the safety and efficacy of 90Y-labeled microspheres in patients with liver metastases.192
Yttrium-90–labeled microspheres are currently approved by the U.S. Food and Drug Administration (FDA) for the treatment of liver metastases from colorectal cancer. In an early study from Australia, the combination of a single injection of 90Y-labeled microspheres plus regional hepatic artery chemotherapy was substantially more effective in increasing tumor responses and progression-free survival times than the same regimen of hepatic artery chemotherapy alone.193 Clinical tumor response, carcinoembryonic antigen (CEA) level, and survival time were all significantly improved with the addition of 90Y-labeled microspheres. In another study by the same group, adding 90Y-labeled microspheres to systemic therapy yielded improved response rates and had acceptable toxicity.194 From a pooled analysis, 19 studies were examined that specifically investigated patients receiving 90Y-labeled microspheres for liver metastases from colorectal cancer. The reported median survival time ranged from 10.8 months to 29.4 months.190
Liver metastases from neuroendocrine tumors also appear to be effectively treated with 90Y-labeled microspheres. In the study from Northwestern University, 19 patients had neuroendocrine carcinomas. Among these patients, the median survival time was 26 months and the 2-year survival rate was 69%.191 In a multi-institutional report, 148 patients with liver metastases from neuroendocrine tumors underwent 185 administrations of 90Y-labeled microspheres.125 After treatment, 23% had stable disease, 61% had partial response, 3% had complete response, and 5% had progressive disease. The 2-year survival rate was approximately 75%, and the median survival time was approximately 70 months. In another multi-institutional study, 42 patients underwent 90Y-labeled microsphere therapy for liver metastases from neuroendocrine tumors.195 More than 90% achieved stable disease or a partial response. The median survival time was on the order of 2 years. In an Australian study, 34 patients with liver metastases from neuroendocrine tumors were treated with 90Y-labeled microspheres. Radiologic liver responses were observed in 50% of patients and included 6 (18%) complete responses and 11 (32%) partial responses; the mean overall survival time was 29 months. Symptoms from the tumor were improved in 50%. The differences in survival times between these studies demonstrate a heterogeneous patient population. Also, the survival time without 90Y-labeled microsphere treatment cannot be determined in these patients, with a condition that is often slowly progressive. Only one of these studies addressed the potential of 90Y-labeled microsphere treatment to alleviate symptoms from carcinoid tumors.
From a recent consensus statement addressing patient eligibility for 90Y-labeled microspheres, “Patients considered for radioembolization therapy would include those with (1) unresectable hepatic primary or metastatic cancer, (2) liver-dominant tumor burden, and (3) a life expectancy of at least 3 months. Contraindications for radioembolization therapy may include (1) a pretreatment 99mTc macroaggregated albumin (MAA) scan demonstrating the potential of 30 Gy or more radiation exposure to the lung or flow to the gastrointestinal tract resulting in extrahepatic deposition of 99mTc MAA that cannot be corrected by catheter embolization techniques, (2) excessive tumor burden with limited hepatic reserve, (3) elevated total bilirubin level (≥2 mg/dL) in the absence of a reversible cause, and (4) compromised portal vein, unless selective or superselective radioembolization can be performed. Patients with prior radiotherapy involving the liver should be carefully reviewed on a case-by-case basis. It is unclear whether capecitabine chemotherapy treatments represent a contraindication to 90Y treatment.”196
Stereotactic Body Radiation Therapy
Because the liver’s regenerative powers make localized high-dose irradiation achievable with minimal toxicity, SBRT has become very popular for patients with a limited number of metastases and minimal extrahepatic tumors.197,198 As with most SBRT approaches, respiratory arrest or gating is preferred, but four-dimensional (4D) CT has also achieved good results. For small central lesions, total doses of 48 or 50 Gy at 10 or 12 Gy per fraction are recommended. Larger tumors or those near the stomach or bowel are more safely treated with fraction sizes of 5 or 6 Gy to a total of 50 to 60 Gy. We generally ensure that 60% of the uninvolved liver receives under 30 Gy and have had no RILD at these doses. Both large lesions and portal lesions can be treated safely, and most studies show local control rates of over 70%.175,197,198 No clear dose response has been measured, but all radiation doses and schedules have produced similar results. Although lethal RILD can occur when the median liver dose exceeds 37 Gy in standard fractionation, little or no toxicity is seen when over 50% to 70% of the liver is maintained under 30 Gy.175,199,200 Patients primarily report decreased appetite and gastritis; moreover, cirrhotic patients may experience exacerbation of hepatitis, and those with subdiaphragmatic tumors can have asymptomatic right pleural effusions. After irradiation, tumors often become hypointensive on CT scans, and surrounding hepatic damage may correspond to the 37-Gy isodose line. These radiographic changes can be confused with tumor progression because tumors reach a maximum size at 6 weeks to 3 months after irradiation and recover at 6 to 9 months. The contralateral lobe of the liver commonly hypertrophies to compensate for the lost liver mass; however, the total liver volume usually maintains normal levels.
Lung Metastases
Disease can occur in the parenchyma and in mediastinal or hilar nodes. Metastases in the lung bases are more common than in upper regions.11 Although peripheral lesions are often asymptomatic, central tumors can cause airway obstruction. Other signs and symptoms include cough, respiratory discomfort, shortness of breath, superior vena cava syndrome, and, in severe cases, hemoptysis or dysphagia. High-speed helical CT allows for high-precision detection of lung tumors smaller than 1 cm. Malignancy can be confirmed using PET-CT for glucose-avid tumor types, including colorectal, lung, and breast cancers.
Treatment
Standard treatment for pulmonary metastases is systemic chemotherapy. Tumor response to chemotherapy can be substantial, but it is usually short lived and often results in eventual recurrence. Minimally invasive surgical techniques paired with advanced imaging of the lung have made it possible to remove many small lesions. Although rarely employed for adults with cancer, resection by open or minimally invasive techniques is commonly used for many pediatric patients with cancer.201,202 As with liver metastases, radiofrequency ablation and radiosurgical techniques are also employed.
Surgical Resection
Surgical management of pulmonary metastases is the accepted approach to therapy of osteosarcoma metastases, other childhood sarcomas, and adult soft tissue sarcomas. Long-term survival and locoregional control are commonly seen with or without postoperative whole lung irradiation.201,202 Bilateral thoracotomies and repeat procedures are also employed. Several studies show that survival is improved with complete metastasectomy, leading some authors to recommend aggressive procedures, including reoperations.203 Other studies recommend these procedures primarily for patients with limited metastases. For example, Aljubran and colleagues201 reported on 85 adolescents and adults with lung metastasis from osteosarcoma. Forty-seven underwent resections, with a 3-year survival rate of 38% following diagnosis of lung metastases compared with 16% in the nonoperated group. Patients with fewer than four lesions had better survival rates, and complete metastasectomy was critical in achieving prolonged survival.
Pulmonary metastasectomy is also employed for renal cell carcinoma, colorectal cancer, lung cancer, and breast cancer. Dahabre and colleagues204 reported on 66 patients with metastatic colorectal cancer limited to the lung. Fifty-seven were treated with surgery and 28 had locoregional control (49% with 2 to 8 years of follow-up). There were no recurrences for a minimum of 8 months in any patient; therefore the study outperformed the usual freedom from progression by systemic therapy. Other studies have been less optimistic. For example, Dalrymple-Hay and associates205 show no significant difference in survival when PET is used to detect and remove pulmonary melanoma metastases; the authors continue to recommend metastasectomy for patients whose disease is limited to the thorax. Similarly, Tanaka and colleagues206 found disease-free survival rates to be no higher than expected in patients with breast cancer who were subjected to pulmonary metastasectomy (5-year survival rate of 31%). In that study, metastasectomy was no longer recommended. However, the availability of video-assisted thoracic surgery (VATS) has greatly improved the ability to remove radiofrequency-ablated pulmonary metastases.207 The full utility of this approach for treatment of metastasis remains to be determined.
Stereotactic Body Radiation Therapy
Several approaches and technologies, including methods and instrumentation for the reduction of respiratory motion, realtime imaging of the lesion during respiration, and gating, have been developed to aid targeting. Radiation doses range from 30 to 66 Gy given in 3 to 10 fractions to 48 to 60 Gy given in 10 to 12 fractions.208,209,210,211 Higher fraction sizes do not necessarily produce higher control rates for metastases, but grade 3 to 5 toxicity is most common with larger fraction sizes and central tumor locations.209 Rusthoven and colleagues211 report on patients with one to three metastases with a cumulative diameter under 7 cm, who were given 48 to 60 Gy in three fractions; 63 lesions were treated in 38 patients, with a local control rate of 96% at 2 years, 8% grade 3 toxicity, and no grade 4 toxicity. Okunieff and associates208 report local failures in 8 of 125 lesions (local control rate of 94%) treated and followed for a minimum of 1 year. Tumors were up to 7.7 cm, and the dose was 50 Gy in 10 fractions. The progression-free survival rate was 16% at 2 years. Grade 3 toxicity was seen in only 2% of patients; there was no grade 4 toxicity.
2 Cleeland CS, Gonin R, Hatfield AK, et al. Pain and its treatment in outpatients with metastatic cancer. N Engl J Med. 1994;330:592-596.
5 Sperduto PW, Berkey B, Gaspar LE, et al. A new prognostic index and comparison to three other indices for patients with brain metastases. An analysis of 1,960 patients in the RTOG database. Int J Radiat Oncol Biol Phys. 2008;70:510-514.
15 Laing AH, Ackery DM, Bayly RJ, et al. Strontium 89 chloride for pain palliation in prostatic skeletal malignancy. Br J Radiol. 1991;64:816-822.
23 Houston SJ, Rubens RD. The systemic treatment of bone metastases. Clin Orthop Relat Res. 1995;312:95-104.
28 Galasko CS. Diagnosis of skeletal metastases and assessment of response to treatment. Clin Orthop Relat Res. 1995;312:64-75.
35 Even-Sapir E, Metser U, Flusser G, et al. Assessment of malignant skeletal disease. Initial experience with 18F-fluoride PET/CT and comparison between 18F-fluoride PET and 18F-fluoride PET/CT. J Nucl Med. 2004;45:272-278.
46 Tong D, Gillick L, Hendrickson FR. The palliation of symptomatic osseous metastases. Final results of the study by the Radiation Therapy Oncology Group. Cancer. 1982;50:893-899.
56 Saad F, Gleason DM, Murray R, et al. A randomized, placebo-controlled trial of zoledronic acid in patients with hormone-refractory metastatic prostate carcinoma. J Natl Cancer Inst. 2002;94:1458-1468.
57 Sahgal A, Larson DA, Chang EL. Stereotactic body radiosurgery for spinal metastases. A critical review. Int J Radiat Oncol Biol Phys. 2008;71:652-665.
66 Fidler M. Prophylactic internal fixation of secondary neoplastic deposits in long bones. Br Med J. 1973;1:341-343.
70 Hipp JA, Springfield DS, Hayes WC. Predicting pathologic fracture risk in the management of metastatic bone defects. Clin Orthop Relat Res. 1995;312:120-135.
75 Blitzer PH. Reanalysis of the RTOG study of the palliation of symptomatic osseous metastasis. Cancer. 1985;55:1468-1472.
77 Price P, Hoskin PJ, Easton D, et al. Prospective randomised trial of single and multifraction radiotherapy schedules in the treatment of painful bony metastases. Radiother Oncol. 1986;6:247-255.
79 Nielsen OS, Bentzen SM, Sandberg E, et al. Randomized trial of single dose versus fractionated palliative radiotherapy of bone metastases. Radiother Oncol. 1998;47:233-240.
80 Wu JS, Wong R, Johnston M, et al. Meta-analysis of dose-fractionation radiotherapy trials for the palliation of painful bone metastases. Int J Radiat Oncol Biol Phys. 2003;55:594-605.
82 Poulter CA, Cosmatos D, Rubin P, et al. A report of RTOG 8206. A phase III study of whether the addition of single dose hemibody irradiation to standard fractionated local field irradiation is more effective than local field irradiation alone in the treatment of symptomatic osseous metastases. Int J Radiat Oncol Biol Phys. 1992;23:207-214.
83 Chang EL, Shiu AS, Mendel E, et al. Phase I/II study of stereotactic body radiotherapy for spinal metastasis and its pattern of failure. J Neurosurg Spine. 2007;7:151-160.
89 Ryu S, Jin R, Jin JY, et al. Pain control by image-guided radiosurgery for solitary spinal metastasis. J Pain Symptom Manage. 2008;35:292-298.
91 Amdur RJ, Bennett J, Olivier K, et al. A prospective, phase II study demonstrating the potential value and limitation of radiosurgery for spine metastases. Am J Clin Oncol. June 12, 2009. [Epub ahead of print.]
98 Ben Josef E, Lucas DR, Vasan S, et al. Selective accumulation of strontium-89 in metastatic deposits in bone: radio-histological correlation. Nucl Med Commun. 1995;16:457-463.
107 Porter AT, McEwan AJ, Powe JE, et al. Results of a randomized phase-III trial to evaluate the efficacy of strontium-89 adjuvant to local field external beam irradiation in the management of endocrine resistant metastatic prostate cancer. Int J Radiat Oncol Biol Phys. 1993;25:805-813.
116 Sun H, Nemecek AN. Optimal management of malignant epidural spinal cord compression. Hematol Oncol Clin North Am. 2010;24:537-551.
122 Young RF, Post EM, King GA. Treatment of spinal epidural metastases. Randomized prospective comparison of laminectomy and radiotherapy. J Neurosurg. 1980;53:741-748.
124 Zelefsky MJ, Scher HI, Krol G, et al. Spinal epidural tumor in patients with prostate cancer. Clinical and radiographic predictors of response to radiation therapy. Cancer. 1992;70:2319-2325.
128 Patchell RA, Tibbs PA, Walsh JW, et al. A randomized trial of surgery in the treatment of single metastases to the brain. N Engl J Med. 1990;322:494-500.
131 Gaspar L, Scott C, Rotman M, et al. Recursive partitioning analysis (RPA) of prognostic factors in three Radiation Therapy Oncology Group (RTOG) brain metastases trials. Int J Radiat Oncol Biol Phys. 1997;37:745-751.
135 Shaw E, Scott C, Souhami L, et al. Single dose radiosurgical treatment of recurrent previously irradiated primary brain tumors and brain metastases. Final report of RTOG protocol 90-05. Int J Radiat Oncol Biol Phys. 2000;47:291-298.
138 Andrews DW, Scott CB, Sperduto PW, et al. Whole brain radiation therapy with or without stereotactic radiosurgery boost for patients with one to three brain metastases. Phase III results of the RTOG 9508 randomised trial. Lancet. 2004;363:1665-1672.
140 Mehta MP, Tsao MN, Whelan TJ, et al. The American Society for Therapeutic Radiology and Oncology (ASTRO) evidence-based review of the role of radiosurgery for brain metastases. Int J Radiat Oncol Biol Phys. 2005;63:37-46.
142 Varlotto JM, Flickinger JC, Niranjan A, et al. The impact of whole-brain radiation therapy on the long-term control and morbidity of patients surviving more than one year after gamma knife radiosurgery for brain metastases. Int J Radiat Oncol Biol Phys. 2005;62:1125-1132.
148 Chidel MA, Suh JH, Reddy CA, et al. Application of recursive partitioning analysis and evaluation of the use of whole brain radiation among patients treated with stereotactic radiosurgery for newly diagnosed brain metastases. Int J Radiat Oncol Biol Phys. 2000;47:993-999.
152 Sneed PK, Lamborn KR, Forstner JM, et al. Radiosurgery for brain metastases. Is whole brain radiotherapy necessary? Int J Radiat Oncol Biol Phys. 1999;43:549-558.
166 Aoyama H, Tago M, Kato N, et al. Neurocognitive function of patients with brain metastasis who received either whole brain radiotherapy plus stereotactic radiosurgery or radiosurgery alone. Int J Radiat Oncol Biol Phys. 2007;68:1388-1395.
170 Baron O, Amini M, Duveau D, et al. Surgical resection of pulmonary metastases from colorectal carcinoma. Five-year survival and main prognostic factors. Eur J Cardiothorac Surg. 1996;10:347-351.
171 Fernandez FG, Drebin JA, Linehan DC, et al. Five-year survival after resection of hepatic metastases from colorectal cancer in patients screened by positron emission tomography with F-18 fluorodeoxyglucose (FDG-PET). Ann Surg. 2004;240:438-447.
175 Katz AW, Carey-Sampson M, Muhs AG, et al. Hypofractionated stereotactic body radiation therapy (SBRT) for limited hepatic metastases. Int J Radiat Oncol Biol Phys. 2007;67:793-798.
190 Vente MA, Wondergem M, van dT I, et al. Yttrium-90 microsphere radioembolization for the treatment of liver malignancies: a structured meta-analysis. Eur Radiol. 2009;19:951-959.
197 Dawood O, Mahadevan A, Goodman KA. Stereotactic body radiation therapy for liver metastases. Eur J Cancer. 2009;45:2947-2959.
198 Lo SS, Fakiris AJ, Teh BS, et al. Stereotactic body radiation therapy for oligometastases. Expert Rev Anticancer Ther. 2009;9:621-635.
200 Dawson LA, Ten Haken RK. Partial volume tolerance of the liver to radiation. Semin Radiat Oncol. 2005;15:279-283.
208 Okunieff P, Petersen AL, Philip A, et al. Stereotactic body radiation therapy (SBRT) for lung metastases. Acta Oncol. 2006;45:808-817.
209 Chi A, Liao Z, Nguyen NP, et al. Systemic review of the patterns of failure following stereotactic body radiation therapy in early-stage non-small-cell lung cancer. Clinical implications. Radiother Oncol. 2010;94:1-11.
211 Rusthoven KE, Kavanagh BD, Burri SH, et al. Multi-institutional phase I/II trial of stereotactic body radiation therapy for lung metastases. J Clin Oncol. 2009;27:1579-1584.
215 Steenland E, Leer JW, van Houwelingen H, et al. The effect of a single fraction compared to multiple fractions on painful bone metastases. A global analysis of the Dutch Bone Metastasis Study. Radiother Oncol. 1999;52:101-109.
216 Sze WM, Shelley MD, Held I, et al. Palliation of metastatic bone pain: single fraction versus multifraction radiotherapy—a systematic review of randomised trials. Clin Oncol (R Coll Radiol). 2003;15:345-352.
1 Von Roenn JH, Cleeland CS, Gonin R, et al. Physician attitudes and practice in cancer pain management. A survey from the Eastern Cooperative Oncology Group. Ann Intern Med. 1993;119:121-126.
2 Cleeland CS, Gonin R, Hatfield AK, et al. Pain and its treatment in outpatients with metastatic cancer. N Engl J Med. 1994;330:592-596.
3 Foley KM. The treatment of cancer pain. N Engl J Med. 1985;313:84-95.
4 Abrams HL, Spiro R, Goldstein N. Metastases in carcinoma. Analysis of 1000 autopsied cases. Cancer. 1950;3:74-85.
5 Sperduto PW, Berkey B, Gaspar LE, et al. A new prognostic index and comparison to three other indices for patients with brain metastases. An analysis of 1,960 patients in the RTOG database. Int J Radiat Oncol Biol Phys. 2008;70:510-514.
6 Nieder C, Geinitz H, Molls M. Validation of the graded prognostic assessment index for surgically treated patients with brain metastases. Anticancer Res. 2008;28:3015-3017.
7 Lyss AP, Lilenbaum RC. Accrual to National Cancer Institute-sponsored non-small-cell lung cancer trials. Insights and contributions from the CCOP program. Clin Lung Cancer. 2009;10:410-413.
8 Catinella FP, Kittle CF, Faber LP, et al. Surgical treatment of primary lung cancer and solitary intracranial metastasis. Chest. 2009;136:e30.
9 Aoyama H. Radiation therapy for brain metastases in breast cancer patients. Breast Cancer. May 11, 2010. [Epub ahead of print.]
10 DeVita VT, Lawrence TS, Rosenberg SA, et al. DeVita, Hellman, and Rosenberg’s Cancer: Principles and practice of oncology, ed 8. Philadelphia: Wolters Kluwer/Lippincott Williams & Wilkins; 2008.
11 Friedel G, Pastorino U, Ginsberg RJ, et al. Results of lung metastasectomy from breast cancer: prognostic criteria on the basis of 467 cases of the International Registry of Lung Metastases. Eur J Cardiothorac Surg. 2002;22:335-344.
12 Galasko CSB. Incidence and distribution of skeletal metastases. Clin Orthop Relat Res. 1986;210:14-22.
13 Tofe AJ, Francis MD, Harvey WJ. Correlation of neoplasms with incidence and localization of skeletal metastases. An analysis of 1,355 diphosphonate bone scans. J Nucl Med. 1975;16:986-989.
14 Clain A. Secondary malignant disease of bone. Br J Cancer. 1965;19:15-29.
15 Laing AH, Ackery DM, Bayly RJ, et al. Strontium 89 chloride for pain palliation in prostatic skeletal malignancy. Br J Radiol. 1991;64:816-822.
16 Morgan JW, Adcock KA, Donohue RE. Distribution of skeletal metastases in prostatic and lung cancer. Mechanisms of skeletal metastases. Urology. 1990;36:31-34.
17 Wilson MA, Calhoun FW. The distribution of skeletal metastases in breast and pulmonary cancer: concise communication. J Nucl Med. 1981;22:594-597.
18 Harrington KD. Mechanisms of metastases. In: In, Harrington KD. Orthopedic management of metastatic bone disease. St. Louis, C.V: Mosby; 1988:19-30.
19 Cumming JD. A study of blood flow through bone marrow by a method of venous effluent collection. J Physiol. 1962;162:13-20.
20 Weiss L. Dynamic aspects of cancer cell populations in metastasis. Am J Pathol. 1979;97:601-608.
21 Springfield DS. Mechanisms of metastasis. Clin Orthop Relat Res. 1982;169:15-19.
22 Garrett IR. Bone destruction in cancer. Semin Oncol. 1993;20:4-9.
23 Houston SJ, Rubens RD. The systemic treatment of bone metastases. Clin Orthop Relat Res. 1995;312:95-104.
24 Galasko CS. Skeletal metastases and mammary cancer. Ann R Coll Surg Engl. 1972;50:3-28.
25 Cuschieri A, Jarvie R, Taylor WH, et al. Three-centre study on urinary hydroxyproline excretion in cancer of the breast. Br J Cancer. 1978;37:1002-1005.
26 Galasko CSB. The mechanism of uptake of bone-seeking isotopes by skeletal metastases. In: In, editor. Medical radionuclide imaging. ed 2. Vienna: International Atomic Energy Agency (IAEA); 1982:125-134.
27 Galasko CSB. The pathophysiological basis for skeletal scintigraphy. In: In, Galasko CSB, Weber DA. Radionuclide Scintigraphy in Orthopaedics (Current Problems in Orthopaedics). Edinburgh: Churchill Livingstone; 1984:210-234.
28 Galasko CS. Diagnosis of skeletal metastases and assessment of response to treatment. Clin Orthop Relat Res. 1995;312:64-75.
29 Johnston AD. Pathology of metastatic tumors in bone. Clin Orthop Relat Res. 1970;73:8-32.
30 Muindi J, Coombes RC, Golding S, et al. The role of computed tomography in the detection of bone metastases in breast cancer patients. Br J Radiol. 1983;56:233-236.
31 Daffner RH, Lupetin AR, Dash N, et al. MRI in the detection of malignant infiltration of bone marrow. AJR Am J Roentgenol. 1986;146:353-358.
32 Hollis PH, Malis LI, Zappulla RA. Neurological deterioration after lumbar puncture below complete spinal subarachnoid block. J Neurosurg. 1986;64:253-256.
33 Bonner JA, Lichter AS. A caution about the use of MRI to diagnose spinal cord compression. N Engl J Med. 1990;322:556-557.
34 Lauenstein TC, Goehde SC, Herborn CU, et al. Whole-body MR imaging. Evaluation of patients for metastases. Radiology. 2004;233:139-148.
35 Even-Sapir E, Metser U, Flusser G, et al. Assessment of malignant skeletal disease. Initial experience with 18F-fluoride PET/CT and comparison between 18F-fluoride PET and 18F-fluoride PET/CT. J Nucl Med. 2004;45:272-278.
36 Yang SN, Liang JA, Lin FJ, et al. Comparing whole body (18)F-2-deoxyglucose positron emission tomography and technetium-99m methylene diphosphonate bone scan to detect bone metastases in patients with breast cancer. J Cancer Res Clin Oncol. 2002;128:325-328.
37 Duarte PS, Zhuang H, Castellucci P, et al. The receiver operating characteristic curve for the standard uptake value in a group of patients with bone marrow metastasis. Mol Imaging Biol. 2002;4:157-160.
38 Gayed I, Vu T, Johnson M, et al. Comparison of bone and 2-deoxy-2-[18F]fluoro-D-glucose positron emission tomography in the evaluation of bony metastases in lung cancer. Mol Imaging Biol. 2003;5:26-31.
39 Ohta M, Tokuda Y, Suzuki Y, et al. Whole body PET for the evaluation of bony metastases in patients with breast cancer. Comparison with 99Tcm-MDP bone scintigraphy. Nucl Med Commun. 2001;22:875-879.
40 Wong J, Sharpe M, Jaffray D: The use of active breathing control (ABC) to minimize breathing motion in conformal therapy. Proceedings of the XIIth ICCR Medical Physics Publishing, 1997, pp 220-222.
41 Hamaoka T, Madewell JE, Podoloff DA, et al. Bone imaging in metastatic breast cancer. J Clin Oncol. 2004;22:2942-2953.
42 Hoh CK, Seltzer MA, Franklin J, et al. Positron emission tomography in urological oncology. J Urol. 1998;159:347-356.
43 Shreve PD, Grossman HB, Gross MD, et al. Metastatic prostate cancer. Initial findings of PET with 2-deoxy-2-[F-18]fluoro-D-glucose. Radiology. 1996;199:751-756.
44 Charron M, Beyer T, Bohnen NN, et al. Image analysis in patients with cancer studied with a combined PET and CT scanner. Clin Nucl Med. 2000;25:905-910.
45 Metser U, Lerman H, Blank A, et al. Malignant involvement of the spine. Assessment by 18F-FDG PET/CT. J Nucl Med. 2004;45:279-284.
46 Tong D, Gillick L, Hendrickson FR. The palliation of symptomatic osseous metastases. Final results of the study by the Radiation Therapy Oncology Group. Cancer. 1982;50:893-899.
47 Kjaer M. The treatment and prognosis of patients with renal adenocarcinoma with solitary metastasis. 10 year survival results. Int J Radiat Oncol Biol Phys. 1987;13:619-621.
48 Burckhardt P, Thiebaud D, Perey L, et al. Treatment of tumor-induced osteolysis by APD. Recent Results Cancer Res. 1989;116:54-66.
49 Coleman RE, Woll PJ, Miles M, et al. Treatment of bone metastases from breast cancer with (3-amino-1-hydroxypropylidene)-1,1-bisphosphonate (APD). Br J Cancer. 1988;58:621-625.
50 Lipton A, Glover D, Harvey H, et al. Pamidronate in the treatment of bone metastases. Results of 2 dose-ranging trials in patients with breast or prostate cancer. Ann Oncol. 1994;5(Suppl 7):S31-S35.
51 Morton AR, Cantrill JA, Pillai GV, et al. Sclerosis of lytic bone metastases after disodium aminohydroxypropylidene bisphosphonate (APD) in patients with breast carcinoma. BMJ. 1988;297:772-773.
52 Berenson JR, Rosen LS, Howell A, et al. Zoledronic acid reduces skeletal-related events in patients with osteolytic metastases. Cancer. 2001;91:1191-1200.
53 Rosen LS, Gordon D, Kaminski M, et al. Zoledronic acid versus pamidronate in the treatment of skeletal metastases in patients with breast cancer or osteolytic lesions of multiple myeloma. A phase III, double-blind, comparative trial. Cancer J. 2001;7:377-387.
54 Hillner BE, Ingle JN, Chlebowski RT, et al. American Society of Clinical Oncology 2003 update on the role of bisphosphonates and bone health issues in women with breast cancer. J Clin Oncol. 2003;21:4042-4057.
55 Pavlakis N, Stockler M: Bisphosphonates for breast cancer. Cochrane Database Syst Rev 1:CD003474, 2002.
56 Saad F, Gleason DM, Murray R, et al. A randomized, placebo-controlled trial of zoledronic acid in patients with hormone-refractory metastatic prostate carcinoma. J Natl Cancer Inst. 2002;94:1458-1468.
57 Sahgal A, Larson DA, Chang EL. Stereotactic body radiosurgery for spinal metastases. A critical review. Int J Radiat Oncol Biol Phys. 2008;71:652-665.
58 Ruggiero SL, Mehrotra B, Rosenberg TJ, et al. Osteonecrosis of the jaws associated with the use of bisphosphonates. A review of 63 cases. J Oral Maxillofac Surg. 2004;62:527-534.
59 Bonica JJ. The management of pain. Philadelphia: Lea & Febiger; 1990.
60 Levy MH. Pharmacologic treatment of cancer pain. N Engl J Med. 1996;335:1124-1132.
61 Twycross RG. Management of pain in skeletal metastases. Clin Orthop Relat Res. 1995;312:187-196.
62 Harrington KD. Prophylactic management of impending fractures. In: In, Harrington KD. Orthopaedic management of metastatic bone disease. St. Louis, C.V: Mosby; 1988:283-307.
63 Pugh J, Sherry HS, Futterman B, et al. Biomechanics of pathologic fractures. Clin Orthop Relat Res. 1982;169:109-114.
64 Healey JH. Metastatic cancer to the bone. In: In, DeVita VT, Hellman S, Rosenberg SA. Cancer: Principles and practice of oncology. ed 5. Philadelphia: Lippincott-Raven; 1997:2570-2583.
65 Hipp JA, Katz G, Hayes WC. Local demineralization as a model for bone strength reductions in lytic transcortical metastatic lesions. Invest Radiol. 1991;26:934-938.
66 Fidler M. Prophylactic internal fixation of secondary neoplastic deposits in long bones. Br Med J. 1973;1:341-343.
67 Reference deleted in proofs.
68 Keene JS, Sellinger DS, McBeath AA, et al. Metastatic breast cancer in the femur. A search for the lesion at risk of fracture. Clin Orthop Relat Res. 1986;203:282-288.
69 Mirels H. Metastatic disease in long bones. A proposed scoring system for diagnosing impending pathologic fractures. Clin Orthop Relat Res. 1989;249:256-264.
70 Hipp JA, Springfield DS, Hayes WC. Predicting pathologic fracture risk in the management of metastatic bone defects. Clin Orthop Relat Res. 1995;312:120-135.
71 Harrington KD. Management of lower extremity metastases. In: In, Harrington KD. Orthopaedic management of metastatic bone disease. St. Louis, C.V: Mosby; 1988:141-214.
72 Harrington KD. Metastatic disease of the spine. In: In, Harrington KD. Orthopaedic management of metastatic bone disease. St. Louis, C.V: Mosby; 1988:309-383.
73 Wenger M. Vertebroplasty for metastasis. Med Oncol. 2003;20:203-209.
74 Hoskin PJ. Scientific and clinical aspects of radiotherapy in the relief of bone pain. Cancer Surv. 1988;7:69-86.
75 Blitzer PH. Reanalysis of the RTOG study of the palliation of symptomatic osseous metastasis. Cancer. 1985;55:1468-1472.
76 Orton CG, Ellis F. A simplification in the use of the NSD concept in practical radiotherapy. Br J Radiol. 1973;46:529-537.
77 Price P, Hoskin PJ, Easton D, et al. Prospective randomised trial of single and multifraction radiotherapy schedules in the treatment of painful bony metastases. Radiother Oncol. 1986;6:247-255.
78 Hoskin PJ, Price P, Easton D, et al. A prospective randomised trial of 4 Gy or 8 Gy single doses in the treatment of metastatic bone pain. Radiother Oncol. 1992;23:74-78.
79 Nielsen OS, Bentzen SM, Sandberg E, et al. Randomized trial of single dose versus fractionated palliative radiotherapy of bone metastases. Radiother Oncol. 1998;47:233-240.
80 Wu JS, Wong R, Johnston M, et al. Meta-analysis of dose-fractionation radiotherapy trials for the palliation of painful bone metastases. Int J Radiat Oncol Biol Phys. 2003;55:594-605.
81 van der Linden YM, Lok JJ, Steenland E, et al. Single fraction radiotherapy is efficacious. A further analysis of the Dutch Bone Metastasis Study controlling for the influence of retreatment. Int J Radiat Oncol Biol Phys. 2004;59:528-537.
82 Poulter CA, Cosmatos D, Rubin P, et al. A report of RTOG 8206. A phase III study of whether the addition of single dose hemibody irradiation to standard fractionated local field irradiation is more effective than local field irradiation alone in the treatment of symptomatic osseous metastases. Int J Radiat Oncol Biol Phys. 1992;23:207-214.
83 Chang EL, Shiu AS, Mendel E, et al. Phase I/II study of stereotactic body radiotherapy for spinal metastasis and its pattern of failure. J Neurosurg Spine. 2007;7:151-160.
84 Gerszten PC, Burton SA, Ozhasoglu C, et al. Radiosurgery for spinal metastases. Clinical experience in 500 cases from a single institution. Spine. 2007;32:193-199.
85 Ryu S, Rock J, Rosenblum M, et al. Patterns of failure after single-dose radiosurgery for spinal metastasis. J Neurosurg. 2004;101(Suppl 3):402-405.
86 Yamada Y, Bilsky MH, Lovelock DM, et al. High-dose, single-fraction image-guided intensity-modulated radiotherapy for metastatic spinal lesions. Int J Radiat Oncol Biol Phys. 2008;71:484-490.
87 Yamada Y, Lovelock DM, Yenice KM, et al. Multifractionated image-guided and stereotactic intensity-modulated radiotherapy of paraspinal tumors. A preliminary report. Int J Radiat Oncol Biol Phys. 2005;62:53-61.
88 Gibbs IC, Kamnerdsupaphon P, Ryu MR, et al. Image-guided robotic radiosurgery for spinal metastases. Radiother Oncol. 2007;82:185-190.
89 Ryu S, Jin R, Jin JY, et al. Pain control by image-guided radiosurgery for solitary spinal metastasis. J Pain Symptom Manage. 2008;35:292-298.
90 Ryu S, Jin JY, Jin R, et al. Partial volume tolerance of the spinal cord and complications of single-dose radiosurgery. Cancer. 2007;109:628-636.
91 Amdur RJ, Bennett J, Olivier K, et al. A prospective, phase II study demonstrating the potential value and limitation of radiosurgery for spine metastases. Am J Clin Oncol. June 12, 2009. [Epub ahead of print.]
92 Ryu S, Fang YF, Rock J, et al. Image-guided and intensity-modulated radiosurgery for patients with spinal metastasis. Cancer. 2003;97:2013-2018.
93 Sahgal A, Ma L, Gibbs I, et al. Spinal cord tolerance for stereotactic body radiotherapy. Int J Radiat Oncol Biol Phys. 2010;77:548-553.
94 Pecher C. Biological investigation with radioactive calcium and strontium. Preliminary report on the use of radioactive strontium in the treatment of metastatic bone cancer. Univ Calif Publ Pharmacology. 1942;11:117-149.
95 Silberstein EB. The treatment of painful osseous metastases with phosphorus-32-labeled phosphates. Semin Oncol. 1993;20:10-21.
96 Blake GM, Zivanovic MA, McEwan AJ, et al. Sr-89 therapy. Strontium kinetics in disseminated carcinoma of the prostate. Eur J Nucl Med. 1986;12:447-454.
97 Breen SL, Powe JE, Porter AT. Dose estimation in strontium-89 radiotherapy of metastatic prostatic carcinoma. J Nucl Med. 1992;33:1316-1323.
98 Ben Josef E, Lucas DR, Vasan S, et al. Selective accumulation of strontium-89 in metastatic deposits in bone: radio-histological correlation. Nucl Med Commun. 1995;16:457-463.
99 Blake GM, Zivanovic MA, Blaquiere RM, et al. Strontium-89 therapy. Measurement of absorbed dose to skeletal metastases. J Nucl Med. 1988;29:549-557.
100 Ben Josef E, Maughan RL, Vasan S, et al. A direct measurement of strontium-89 activity in bone metastases. Nucl Med Commun. 1995;16:452-456.
101 Tennvall J, Darte L, Lundgren R, et al. Palliation of multiple bone metastases from prostatic carcinoma with strontium-89. Acta Oncol. 1988;27:365-369.
102 Silberstein EB, Williams C. Strontium-89 therapy for the pain of osseous metastases. J Nucl Med. 1985;26:345-348.
103 Laing AH, Ackery DM, Bayly RJ, et al. Strontium-89 chloride for pain palliation in prostatic skeletal malignancy. Br J Radiol. 1991;64:816-822.
104 Robinson RG, Spicer JA, Preston DF, et al. Treatment of metastatic bone pain with strontium-89. Int J Rad Appl Instrum B. 1987;14:219-222.
105 Correns HJ, Mebel M, Buchali K, et al. 89Strontium therapy of bone metastases of carcinoma of the prostatic gland. Eur J Nucl Med. 1979;4:33-35.
106 Firusian N, Mellin P, Schmidt CG. Results of 89strontium therapy in patients with carcinoma of the prostate and incurable pain from bone metastases. A preliminary report. J Urol. 1976;116:764-768.
107 Porter AT, McEwan AJ, Powe JE, et al. Results of a randomized phase-III trial to evaluate the efficacy of strontium-89 adjuvant to local field external beam irradiation in the management of endocrine resistant metastatic prostate cancer. Int J Radiat Oncol Biol Phys. 1993;25:805-813.
108 Bayouth JE, Macey DJ, Kasi LP, et al. Dosimetry and toxicity of samarium-153-EDTMP administered for bone pain due to skeletal metastases. J Nucl Med. 1994;35:63-69.
109 Eary JF, Collins C, Stabin M, et al. Samarium-153-EDTMP biodistribution and dosimetry estimation. J Nucl Med. 1993;34:1031-1036.
110 Collins C, Eary JF, Donaldson G, et al. Samarium-153-EDTMP in bone metastases of hormone refractory prostate carcinoma. A phase I/II trial. J Nucl Med. 1993;34:1839-1844.
111 Maxon HR3, Schroder LE, Thomas SR, et al. Re-186(Sn) HEDP for treatment of painful osseous metastases. Initial clinical experience in 20 patients with hormone-resistant prostate cancer. Radiology. 1990;176:155-159.
112 Maxon HR3, Thomas SR, Hertzberg VS, et al. Rhenium-186 hydroxyethylidene diphophonate for the treatment of painful osseous metastases. Semin Nucl Med. 1992;22:33-40.
113 Maxon HR3, Schroder LE, Hertzberg VS, et al. Rhenium-186(Sn)HEDP for treatment of painful osseous metastases. Results of a double-blind crossover comparison with placebo. J Nucl Med. 1991;32:1877-1881.
114 Niibe Y, Kuranami M, Matsunaga K, et al. Value of high-dose radiation therapy for isolated osseous metastasis in breast cancer in terms of oligo-recurrence. Anticancer Res. 2008;28:3929-3931.
115 Turner S, Marosszeky B, Timms I, et al. Malignant spinal cord compression. A prospective evaluation. Int J Radiat Oncol Biol Phys. 1993;26:141-146.
116 Sun H, Nemecek AN. Optimal management of malignant epidural spinal cord compression. Hematol Oncol Clin North Am. 2010;24:537-551.
117 Gilbert RW, Kim JH, Posner JB. Epidural spinal cord compression from metastatic tumor: diagnosis and treatment. Ann Neurol. 1978;3:40-51.
118 Graus F, Krol G, Foley K. Early diagnosis of spinal epidural metastases. Correlation with clinical and radiological findings. Proc Am Soc Clin Oncol. 1985;4:269.
119 Han JS, Benson JE, Yoon YS. Magnetic resonance imaging in the spinal column and craniovertebral junction. Radiol Clin North Am. 1984;22:805-827.
120 Modic MT, Weinstein MA, Pavlicek W, et al. Nuclear magnetic resonance imaging of the spine. Radiology. 1983;148:757-762.
121 Paushter DM. MT—Magnetic resonance imaging of the spine. Appl Radiol. 1984;13:61-68.
122 Young RF, Post EM, King GA. Treatment of spinal epidural metastases. Randomized prospective comparison of laminectomy and radiotherapy. J Neurosurg. 1980;53:741-748.
123 Patchell RA, Tibbs PA, Regine WF, et al. Direct decompressive surgical resection in the treatment of spinal cord compression caused by metastatic cancer. A randomised trial. Lancet. 2005;366:643-648.
124 Zelefsky MJ, Scher HI, Krol G, et al. Spinal epidural tumor in patients with prostate cancer. Clinical and radiographic predictors of response to radiation therapy. Cancer. 1992;70:2319-2325.
125 Kennedy AS, Dezarn WA, McNeillie P, et al. Radioembolization for unresectable neuroendocrine hepatic metastases using resin 90Y-microspheres. Early results in 148 patients. Am J Clin Oncol. 2008;31:271-279.
126 Hwang TL, Close TP, Grego JM, et al. Predilection of brain metastasis in gray and white matter junction and vascular border zones. Cancer. 1996;77:1551-1555.
127 Delattre JY, Krol G, Thaler HT, et al. Distribution of brain metastases. Arch Neurol. 1988;45:741-744.
128 Patchell RA, Tibbs PA, Walsh JW, et al. A randomized trial of surgery in the treatment of single metastases to the brain. N Engl J Med. 1990;322:494-500.
129 Glantz MJ, Cole BF, Forsyth PA, et al. Practice parameter. Anticonvulsant prophylaxis in patients with newly diagnosed brain tumors. Report of the Quality Standards Subcommittee of the American Academy of Neurology. Neurology. 2000;54:1886-1893.
130 El Kamar FG, Posner JB. Brain metastases. Semin Neurol. 2004;24:347-362.
131 Gaspar L, Scott C, Rotman M, et al. Recursive partitioning analysis (RPA) of prognostic factors in three Radiation Therapy Oncology Group (RTOG) brain metastases trials. Int J Radiat Oncol Biol Phys. 1997;37:745-751.
132 Vecht CJ, Haaxma-Reiche H, Noordijk EM, et al. Treatment of single brain metastasis: radiotherapy alone or combined with neurosurgery? Ann Neurol. 1993;33:583-590.
133 Mintz AH, Kestle J, Rathbone MP, et al. A randomized trial to assess the efficacy of surgery in addition to radiotherapy in patients with a single cerebral metastasis. Cancer. 1996;78:1470-1476.
134 Kondziolka D, Patel A, Lunsford LD, et al. Stereotactic radiosurgery plus whole brain radiotherapy versus radiotherapy alone for patients with multiple brain metastases. Int J Radiat Oncol Biol Phys. 1999;45:427-434.
135 Shaw E, Scott C, Souhami L, et al. Single dose radiosurgical treatment of recurrent previously irradiated primary brain tumors and brain metastases. Final report of RTOG protocol 90-05. Int J Radiat Oncol Biol Phys. 2000;47:291-298.
136 Sanghavi SN, Miranpuri SS, Chappell R, et al. Radiosurgery for patients with brain metastases. A multi-institutional analysis, stratified by the RTOG recursive partitioning analysis method. Int J Radiat Oncol Biol Phys. 2001;51:426-434.
137 Sneed PK, Suh JH, Goetsch SJ, et al. A multi-institutional review of radiosurgery alone vs. radiosurgery with whole brain radiotherapy as the initial management of brain metastases. Int J Radiat Oncol Biol Phys. 2002;53:519-526.
138 Andrews DW, Scott CB, Sperduto PW, et al. Whole brain radiation therapy with or without stereotactic radiosurgery boost for patients with one to three brain metastases. Phase III results of the RTOG 9508 randomised trial. Lancet. 2004;363:1665-1672.
139 Kondziolka D, Martin JJ, Flickinger JC, et al. Long-term survivors after gamma knife radiosurgery for brain metastases. Cancer. 2005;104:2784-2791.
140 Mehta MP, Tsao MN, Whelan TJ, et al. The American Society for Therapeutic Radiology and Oncology (ASTRO) evidence-based review of the role of radiosurgery for brain metastases. Int J Radiat Oncol Biol Phys. 2005;63:37-46.
141 Schomas DA, Roeske JC, Macdonald RL, et al. Predictors of tumor control in patients treated with linac-based stereotactic radiosurgery for metastatic disease to the brain. Am J Clin Oncol. 2005;28:180-187.
142 Varlotto JM, Flickinger JC, Niranjan A, et al. The impact of whole-brain radiation therapy on the long-term control and morbidity of patients surviving more than one year after gamma knife radiosurgery for brain metastases. Int J Radiat Oncol Biol Phys. 2005;62:1125-1132.
143 Aoyama H, Shirato H, Tago M, et al. Stereotactic radiosurgery plus whole-brain radiation therapy vs stereotactic radiosurgery alone for treatment of brain metastases. A randomized controlled trial. JAMA. 2006;295:2483-2491.
144 Fuentes R, Bonfill X, Exposito J: Surgery versus radiosurgery for patients with a solitary brain metastasis from non-small cell lung cancer. Cochrane Database Syst Rev 1:CD004840, 2006.
145 Vogelbaum MA, Angelov L, Lee SY, et al. Local control of brain metastases by stereotactic radiosurgery in relation to dose to the tumor margin. J Neurosurg. 2006;104:907-912.
146 Auchter RM, Lamond JP, Alexander E, et al. A multiinstitutional outcome and prognostic factor analysis of radiosurgery for resectable single brain metastasis. Int J Radiat Oncol Biol Phys. 1996;35:27-35.
147 Shiau CY, Sneed PK, Shu HK, et al. Radiosurgery for brain metastases: relationship of dose and pattern of enhancement to local control. Int J Radiat Oncol Biol Phys. 1997;37:375-383.
148 Chidel MA, Suh JH, Reddy CA, et al. Application of recursive partitioning analysis and evaluation of the use of whole brain radiation among patients treated with stereotactic radiosurgery for newly diagnosed brain metastases. Int J Radiat Oncol Biol Phys. 2000;47:993-999.
149 Pirzkall A, Debus J, Lohr F, et al. Radiosurgery alone or in combination with whole-brain radiotherapy for brain metastases. J Clin Oncol. 1998;16:3563-3569.
150 Shehata MK, Young B, Reid B, et al. Stereotactic radiosurgery of 468 brain metastases < or =2 cm. Implications for SRS dose and whole brain radiation therapy. Int J Radiat Oncol Biol Phys. 2004;59:87-93.
151 Hasegawa T, Kondziolka D, Flickinger JC, et al. Brain metastases treated with radiosurgery alone. An alternative to whole brain radiotherapy? Neurosurgery. 2003;52:1318-1326.
152 Sneed PK, Lamborn KR, Forstner JM, et al. Radiosurgery for brain metastases. Is whole brain radiotherapy necessary? Int J Radiat Oncol Biol Phys. 1999;43:549-558.
153 Sawrie SM, Guthrie BL, Spencer SA, et al. Predictors of distant brain recurrence for patients with newly diagnosed brain metastases treated with stereotactic radiosurgery alone. Int J Radiat Oncol Biol Phys. 2008;70:181-186.
154 Muacevic A, Kreth FW, Horstmann GA, et al. Surgery and radiotherapy compared with gamma knife radiosurgery in the treatment of solitary cerebral metastases of small diameter. J Neurosurg. 1999;91:35-43.
155 Rades D, Bohlen G, Pluemer A, et al. Stereotactic radiosurgery alone versus resection plus whole-brain radiotherapy for 1 or 2 brain metastases in recursive partitioning analysis class 1 and 2 patients. Cancer. 2007;109:2515-2521.
156 O’Neill BP, Iturria NJ, Link MJ, et al. A comparison of surgical resection and stereotactic radiosurgery in the treatment of solitary brain metastases. Int J Radiat Oncol Biol Phys. 2003;55:1169-1176.
157 Schoggl A, Kitz K, Reddy M, et al. Defining the role of stereotactic radiosurgery versus microsurgery in the treatment of single brain metastases. Acta Neurochir (Wien). 2000;142:621-626.
158 Baumert BG, Rutten I, Dehing-Oberije C, et al. A pathology-based substrate for target definition in radiosurgery of brain metastases. Int J Radiat Oncol Biol Phys. 2006;66:187-194.
159 Chang EL, Wefel JS, Hess KR, et al. Neurocognition in patients with brain metastases treated with radiosurgery or radiosurgery plus whole-brain irradiation. A randomised controlled trial. Lancet Oncol. 2009;10:1037-1044.
160 Sawaya R. Considerations in the diagnosis and management of brain metastases. Oncology (Williston Park). 2001;15:1144-1148.
161 Wen PY, Loeffler JS. Management of brain metastases. Oncology (Williston Park). 1999;13:941-961.
162 DeAngelis LM, Delattre JY, Posner JB. Radiation-induced dementia in patients cured of brain metastases. Neurology. 1989;39:789-796.
163 Auperin A, Arriagada R, Pignon JP, et al. Prophylactic cranial irradiation for patients with small-cell lung cancer in complete remission. Prophylactic Cranial Irradiation Overview Collaborative Group. N Engl J Med. 1999;341:476-484.
164 Meyers CA, Smith JA, Bezjak A, et al. Neurocognitive function and progression in patients with brain metastases treated with whole-brain radiation and motexafin gadolinium. Results of a randomized phase III trial. J Clin Oncol. 2004;22:157-165.
165 Regine WF, Scott C, Murray K, et al. Neurocognitive outcome in brain metastases patients treated with accelerated-fractionation vs. accelerated-hyperfractionated radiotherapy. An analysis from Radiation Therapy Oncology Group Study 91-04. Int J Radiat Oncol Biol Phys. 2001;51:711-717.
166 Aoyama H, Tago M, Kato N, et al. Neurocognitive function of patients with brain metastasis who received either whole brain radiotherapy plus stereotactic radiosurgery or radiosurgery alone. Int J Radiat Oncol Biol Phys. 2007;68:1388-1395.
167 Hellman S, Weichselbaum RR. Oligometastases. J Clin Oncol. 1995;13:8-10.
168 Rubin P, Green J. Solitary metastases. Springfield, IL: Charles C Thomas; 1968.
169 Rehders A, Hosch SB, Scheunemann P, et al. Benefit of surgical treatment of lung metastasis in soft tissue sarcoma. Arch Surg. 2007;142:70-75.
170 Baron O, Amini M, Duveau D, et al. Surgical resection of pulmonary metastases from colorectal carcinoma. Five-year survival and main prognostic factors. Eur J Cardiothorac Surg. 1996;10:347-351.
171 Fernandez FG, Drebin JA, Linehan DC, et al. Five-year survival after resection of hepatic metastases from colorectal cancer in patients screened by positron emission tomography with F-18 fluorodeoxyglucose (FDG-PET). Ann Surg. 2004;240:438-447.
172 Tanabe K. Emerging therapies for metastatic carcinoma to the liver. Community Oncol. 2006;3:567-573.
173 Okunieff P. Oligometastases. Semin Radiat Oncol. 2006;16(2):65-136.
174 Okunieff P. Design of multi-institutional and cooperative group studies of SBRT. Acta Oncol. 2006;45:775-778.
175 Katz AW, Carey-Sampson M, Muhs AG, et al. Hypofractionated stereotactic body radiation therapy (SBRT) for limited hepatic metastases. Int J Radiat Oncol Biol Phys. 2007;67:793-798.
176 Kavanagh BD, Schefter TE, Cardenes HR, et al. Interim analysis of a prospective phase I/II trial of SBRT for liver metastases. Acta Oncol. 2006;45:848-855.
177 McKay A, Fradette K, Lipschitz J. Long-term outcomes following hepatic resection and radiofrequency ablation of colorectal liver metastases. HPB Surg. 2009. 346863
178 Poulou LS, Ziakas PD, Xila V, et al. Percutaneous radiofrequency ablation for unresectable colorectal liver metastases: time for shadows to disperse. Rev Recent Clin Trials. 2009;4:140-146.
179 Hollett MD, Jeffrey RBJr, Nino-Murcia M, et al. Dual-phase helical CT of the liver. Value of arterial phase scans in the detection of small (< or = 1.5 cm) malignant hepatic neoplasms. AJR Am J Roentgenol. 1995;164:879-884.
180 Jones EC, Chezmar JL, Nelson RC, et al. The frequency and significance of small (less than or equal to 15 mm) hepatic lesions detected by CT. AJR Am J Roentgenol. 1992;158:535-539.
181 Pwint TP, Midgley R, Kerr DJ. Regional hepatic chemotherapies in the treatment of colorectal cancer metastases to the liver. Semin Oncol. 2010;37:149-159.
182 Vogl TJ, Zangos S, Eichler K, et al. Colorectal liver metastases: regional chemotherapy via transarterial chemoembolization (TACE) and hepatic chemoperfusion. An update. Eur Radiol. 2007;17:1025-1034.
183 Martin RC, Robbins K, Tomalty D, et al. Transarterial chemoembolisation (TACE) using irinotecan-loaded beads for the treatment of unresectable metastases to the liver in patients with colorectal cancer. An interim report. World J Surg Oncol. 2009;7:80.
184 You YT, Changchien CR, Huang JS, et al. Combining systemic chemotherapy with chemoembolization in the treatment of unresectable hepatic metastases from colorectal cancer. Int J Colorectal Dis. 2006;21:33-37.
185 Giroux MF, Baum RA, Soulen MC. Chemoembolization of liver metastasis from breast carcinoma. J Vasc Interv Radiol. 2004;15:289-291.
186 Borgelt BB, Gelber R, Brady LW, et al. The palliation of hepatic metastases: results of the Radiation Therapy Oncology Group pilot study. Int J Radiat Oncol Biol Phys. 1981;7:587-591.
187 Russell AH, Clyde C, Wasserman TH, et al. Accelerated hyperfractionated hepatic irradiation in the management of patients with liver metastases. Results of the RTOG dose escalating protocol. Int J Radiat Oncol Biol Phys. 1993;27:117-123.
188 Mohiuddin M, Chen E, Ahmad N. Combined liver radiation and chemotherapy for palliation of hepatic metastases from colorectal cancer. J Clin Oncol. 1996;14:722-728.
189 Bydder S, Spry NA, Christie DR, et al. A prospective trial of short-fractionation radiotherapy for the palliation of liver metastases. Australas Radiol. 2003;47:284-288.
190 Vente MA, Wondergem M, van dT I, et al. Yttrium-90 microsphere radioembolization for the treatment of liver malignancies: a structured meta-analysis. Eur Radiol. 2009;19:951-959.
191 Sato KT, Lewandowski RJ, Mulcahy MF, et al. Unresectable chemorefractory liver metastases: radioembolization with 90Y microspheres—safety, efficacy, and survival. Radiology. 2008;247:507-515.
192 Lewandowski RJ, Thurston KG, Goin JE, et al. 90Y microsphere (TheraSphere) treatment for unresectable colorectal cancer metastases of the liver. Response to treatment at targeted doses of 135-150 Gy as measured by [18F]fluorodeoxyglucose positron emission tomography and computed tomographic imaging. J Vasc Interv Radiol. 2005;16:1641-1651.
193 Gray B, Van Hazel G, Hope M, et al. Randomised trial of SIR-Spheres plus chemotherapy vs. chemotherapy alone for treating patients with liver metastases from primary large bowel cancer. Ann Oncol. 2001;12:1711-1720.
194 Van Hazel G, Blackwell A, Anderson J, et al. Randomised phase 2 trial of SIR-Spheres plus fluorouracil/leucovorin chemotherapy versus fluorouracil/leucovorin chemotherapy alone in advanced colorectal cancer. J Surg Oncol. 2004;88:78-85.
195 Rhee TK, Lewandowski RJ, Liu DM, et al. 90Y Radioembolization for metastatic neuroendocrine liver tumors. Preliminary results from a multi-institutional experience. Ann Surg. 2008;247:1029-1035.
196 Kennedy A, Nag S, Salem R, et al. Recommendations for radioembolization of hepatic malignancies using yttrium-90 microsphere brachytherapy. A consensus panel report from the radioembolization brachytherapy oncology consortium. Int J Radiat Oncol Biol Phys. 2007;68:13-23.
197 Dawood O, Mahadevan A, Goodman KA. Stereotactic body radiation therapy for liver metastases. Eur J Cancer. 2009;45:2947-2959.
198 Lo SS, Fakiris AJ, Teh BS, et al. Stereotactic body radiation therapy for oligometastases. Expert Rev Anticancer Ther. 2009;9:621-635.
199 Lee MT, Kim JJ, Dinniwell R, et al. Phase I study of individualized stereotactic body radiotherapy of liver metastases. J Clin Oncol. 2009;27:1585-1591.
200 Dawson LA, Ten Haken RK. Partial volume tolerance of the liver to radiation. Semin Radiat Oncol. 2005;15:279-283.
201 Aljubran AH, Griffin A, Pintilie M, et al. Osteosarcoma in adolescents and adults. Survival analysis with and without lung metastases. Ann Oncol. 2009;20:1136-1141.
202 Horan TA, Santiago FF, Araujo LM. The benefit of pulmonary metastectomy for bone and soft tissue sarcomas. Int Surg. 2000;85:185-189.
203 Temeck BK, Wexler LH, Steinberg SM, et al. Metastasectomy for sarcomatous pediatric histologies. Results and prognostic factors. Ann Thorac Surg. 1995;59:1385-1389.
204 Dahabre J, Vasilaki M, Stathopoulos GP, et al. Surgical management in lung metastases from colorectal cancer. Anticancer Res. 2007;27:4387-4390.
205 Dalrymple-Hay MJ, Rome PD, Kennedy C, et al. Pulmonary metastatic melanoma—the survival benefit associated with positron emission tomography scanning. Eur J Cardiothorac Surg. 2002;21:611-614.
206 Tanaka F, Li M, Hanaoka N, et al. Surgery for pulmonary nodules in breast cancer patients. Ann Thorac Surg. 2005;79:1711-1714.
207 Ketchedjian A, Daly B, Luketich J, et al. Minimally invasive techniques for managing pulmonary metastases. Video-assisted thoracic surgery and radiofrequency ablation. Thorac Surg Clin. 2006;16:157-165.
208 Okunieff P, Petersen AL, Philip A, et al. Stereotactic body radiation therapy (SBRT) for lung metastases. Acta Oncol. 2006;45:808-817.
209 Chi A, Liao Z, Nguyen NP, et al. Systemic review of the patterns of failure following stereotactic body radiation therapy in early-stage non-small-cell lung cancer. Clinical implications. Radiother Oncol. 2010;94:1-11.
210 Joyner M, Salter BJ, Papanikolaou N, et al. Stereotactic body radiation therapy for centrally located lung lesions. Acta Oncol. 2006;45:802-807.
211 Rusthoven KE, Kavanagh BD, Burri SH, et al. Multi-institutional phase I/II trial of stereotactic body radiation therapy for lung metastases. J Clin Oncol. 2009;27:1579-1584.
212 Press MF, Jones LA, Godolphin W, et al. HER-2/neu oncogene amplification and expression in breast and ovarian cancers. Prog Clin Biol Res. 1990;354A:209-221.
213 Okawa T, Kita M, Goto M, et al. Randomized prospective clinical study of small, large and twice-a-day fraction radiotherapy for painful bone metastases. Radiother Oncol. 1988;13:99-104.
214 Madsen EL. Painful bone metastasis: efficacy of radiotherapy assessed by the patients. A randomized trial comparing 4 Gy × 6 versus 10 Gy × 2. Int J Radiat Oncol Biol Phys. 1983;9:1775-1779.
215 Steenland E, Leer JW, van Houwelingen H, et al. The effect of a single fraction compared to multiple fractions on painful bone metastases. A global analysis of the Dutch Bone Metastasis Study. Radiother Oncol. 1999;52:101-109.
216 Sze WM, Shelley MD, Held I, et al. Palliation of metastatic bone pain: single fraction versus multifraction radiotherapy—a systematic review of randomised trials. Clin Oncol (R Coll Radiol). 2003;15:345-352.
217 Fitzpatrick PJ. Wide-field irradiation of bone metastasis. In: In, Weiss L, Gilbert HA. Bone metastasis. Boston, G.K: Hall Medical Publishers; 1981:83-113.
218 Rowland CG, Bullimore JA, Smith PJ, et al. Half-body irradiation in the treatment of metastatic prostatic carcinoma. Br J Urol. 1981;53:628-629.
219 Qasim MM. Half body irradiation (HBI) in metastatic carcinomas. Clin Radiol. 1981;32:215-219.
220 Salazar OM, Rubin P, Hendrickson FR, et al. Single-dose half-body irradiation for palliation of multiple bone metastases from solid tumors. Final Radiation Therapy Oncology Group report. Cancer. 1986;58:29-36.
221 Wilkins MF, Keen CW. Hemi-body radiotherapy in the management of metastatic carcinoma. Clin Radiol. 1987;38:267-268.
222 Landaw SA. Acute leukemia in polycythemia vera. Semin Hematol. 1986;23:156-165.
223 Quilty PM, Kirk D, Bolger JJ, et al. A comparison of the palliative effects of strontium-89 and external beam radiotherapy in metastatic prostate cancer. Radiother Oncol. 1994;31:33-40.
224 Ahonen A, Joensuu H, Hiltunen J, et al. Samarium-153-EDTMP in bone metastases. J Nucl Biol Med. 1994;38:123-127.