22 Meningeal Tumors
Epidemiology
Meningiomas constitute approximately 20% of all primary intracranial tumors.1,2 Reported incidence rates are between 1 and 5 per 100,000,2–10 with the range perhaps reflecting differences in study design and population exposure to etiologic factors. The Central Brain Tumor Registry of the United States estimated approximately 8600 new cases of meningioma in the United States in 2002.2 Most meningiomas are grossly unifocal. In computed tomography (CT)-era series, multiple synchronous lesions are seen in 40% or fewer of cases,11–16 with the majority of authors reporting multiplicity rates between 5 and 9%.12–15 Intracranial sites for meningioma are listed in order of incidence in Table 22-1. Metastases are extremely rare.17,18 The female/male ratio is approximately 2 : 1 for all meningiomas,1,7,8 and 1 : 1 for anaplastic meningiomas.19 The incidence of benign meningioma increases with age, peaking in the seventh decade of life. The incidence of anaplastic meningioma rises to a plateau in the third decade, where it remains relatively constant thereafter.19 Patients with benign and anaplastic meningioma present at a mean age of 58 ± 15 years (standard error of the mean), and 57 ± 14 years, respectively.19 In the pediatric population, meningiomas are less common, constituting 1% to 4% of all intracranial tumors. There is no female preponderance as seen with adult meningiomas, and there is a common (24%) association with neurofibromatosis.20,21
Site | Incidence, % |
---|---|
Cerebral convexity | 34 |
Parasagittal | 22 |
Sphenoid ridge | 17 |
Lateral ventricle | 5 |
Cerebellar convexity | 5 |
Tentorium | 4 |
Tuberculum sellae/parasellar | 3 |
Olfactory groove | 3 |
Intraorbital | 2 |
Cerebellopontine angle | 2 |
Foramen magnum | 1 |
Clivus | 1 |
Other | 1 |
Data from Rohringer, et al: Incidence and clinicopathologic features of meningioma, J Neurosurg 71:665, 1989.
Ionizing Radiation
Several case reports have identified intracranial meningioma in patients with a history of previous scalp irradiation for tinea capitis,22–24 suggesting an etiologic role. From 1910 to 1959, an estimated 20,000 children in the United States and 200,000 worldwide received such treatments.25 Ron and associates26 compared the incidence of meningioma in a cohort of 10,834 children treated with ionizing radiation in Israel for tinea capitis with that of a nonirradiated control group. With an estimated 1.5 Gy delivered to the brain surface, the relative risk (RR) was 9.5 times that of the control group (95% confidence interval [CI], 3.5-25.7). The mean interval between irradiation and diagnosis with meningioma was 20 years. Rubinstein and associates27 retrospectively analyzed 201 patients with intracranial meningioma, 43 of whom had received childhood radiation for tinea capitis, and identified several distinctive clinical and histologic features in the irradiated subgroup. A statistically significant (P < .001) difference in location was seen favoring falx cerebri, parasagittal, or convexity regions in the irradiated group, corresponding to the site of scalp treatment. Presentation with seizures was more common in the irradiated group (P < .005), perhaps reflecting the predominantly supratentorial location of the tumors. Histologically, the radiation-associated meningiomas (RAMs) had a significantly higher incidence of (1) high cellularity, (2) pleomorphic nuclei, and (3) numerous giant cells (P < .001). Following resection, recurrence rates were 25% versus 11% (P < .05) in the RAM and non-RAM groups, respectively.27 In a comparison between 16 RAM and 17 non-RAM tumors, Rienstein et al28 found no significant differences in the number of genetic changes and the extent and frequency of chromosomes 1 and 22 losses, suggesting similar molecular pathways for tumorogenesis, regardless of radiation exposure (See “Neurofibromatosis and Genetics”). Of note, radiofrequency electromagnetic field exposure, such as cell phone use, has not been associated with meningioma.29
Female Sex Hormones
Several findings suggest a role of female sex hormones in the etiology and regulation of growth of meningiomas: (1) incidence in women is twice that in men;1,7,8 (2) relationship between pregnancy and increased rate of meningioma symptom progression30; (3) approximately three quarters of meningiomas are progesterone receptor (PR) positive;31–36 (4) meningioma is less common in women with history of premenopausal bilateral oophorectomy;37 and more common among postmenopausal women with ever use of hormone replacement therapy (RR = 1.7; 95% CI, 1.0-2.8) or long-term (≥10 years) use of long-acting hormonal contraceptives such as subdermal implants, injections, or hormonal intrauterine devices (RR = 2.7; 95% CI, 0.9-7.5).38 Of note, receptor-negative and estrogen receptor (ER)-positive tumors are associated with higher proliferative index, worse histopathology, and more chromosomal abnormalities than PR-positive meningioma.39
Trauma
Head trauma, hypothesized by some to promote tumorogenesis during the increased mitotic activity of the reparative process, has not been convincingly shown to cause meningioma. In a multicenter study of 330 meningioma cases matched to 1132 controls, Preston-Martin and colleagues40 found no statistically significant relationship between trauma and meningioma—results which refute the authors’ earlier observations41–43 in Los Angeles County. No increased incidence of meningioma was found in a Mayo Clinic review of 3587 residents of Olmstead County who sustained head trauma between 1935 and 1974 associated with loss of consciousness, amnesia, or skull fracture,44 and a case-control study from Schlehofer and colleagues45 actually found a history of head trauma protective, with an RR of 0.5.
Viral Infection
Viral antigen and DNA have been found in meningioma by several investigators.46,47 It has been hypothesized that infection with papovavirus may promote meningioma by causing the loss of a tumor suppressor gene on chromosome 22.48,49 However, the etiologic role of viral infection has not yet been examined epidemiologically.
Neurofibromatosis and Genetics
Losses on 22q are the most frequent genomic alterations observed in meningioma.50,51 Type 2 neurofibromatosis (bilateral acoustic neurofibromatosis, NF2), characterized cytogenetically by the loss of the NF2 gene from 22q12, is associated with an increased incidence of meningioma.52 Based on the most common chromosomal aberrations identified in the various grades of meningioma, a model for progression has been proposed by Weber et al50 that initiates the clonal expansion from normal meningeal cells to benign World Health Organization (WHO) Grade I meningioma with loss on 22q, and continues with cumulative genetic alterations on other chromosomes in higher grade tumors (loss on 1p, 6q, 10, 14q, 18q, and gain on 1q, 9q, 12q, 15q, 17q, and 20 in atypical WHO Grade II; followed by loss on 9p and amplification on 17q in anaplastic WHO Grade III). Quantitative measure of accumulation of chromosome change correlates highly with early tumor recurrence.53 Perhaps contradicting stepwise clonal evolution, Al-Mefty et al have demonstrated deletions of 22, 1p, and 14q ab initio in histologically lower grade tumors that later recur and progress to higher grade, suggesting that benign meningioma presenting with complex genetic alterations are potentially aggressive and require closer follow up.54
Breast Cancer
Both the risk of developing meningioma in patients who have had breast cancer and the risk of developing breast cancer in patients who have had meningioma are higher than in the general population.55 The association may be explained by a hormonal effect or possibly by a shared chromosome 22 abnormality.56–58
Pathology
Meningiomas are thought to arise from arachnoidal cap cells that are found on the outer surface of the arachnoidal membrane between the dura and pia mater. Cells of the arachnoidal membrane uniquely display both mesenchymal and epithelial characteristics, capable of fibro-lipo-chondro-osteoblastic differentiation as well as of well-formed desmosomal connections between tumor cells and other structural and histochemical features usually attributed to epithelial neoplasms. Though much meningioma literature is based on legacy classification systems, most neurooncology communities have now adopted the WHO classification.59
Conventional histologic subtypes, including meningothelial, fibrous, transitional, psammomatous, angiomatous, microcystic, secretory, lymphoplasmacyte-rich, and metaplastic, have no apparent prognostic importance.60 By contrast, four rare variants (each constituting <1% of all meningiomas) have characteristically aggressive behavior:
Clear cell, composed of sheets of clear, glycogen-rich, polygonal cells forming only a few vague whorls; often with extensive stromal and perivascular hyalinization; frequently deceptively benign appearance with few mitotic figures.61
Chordoid, mimicking chordoma with lobular low-power architecture and production of stroma mucosubstances, but with scant reactivity for chordoma-typical epithelial membrane antigen, cytokeratin, and S-100.62
Rhabdoid, usually seen in association with conventional histological subtypes, with increasing prominence with each recurrence; characterized by sheets of loosely cohesive cells with eccentric nuclei and hyaline paranuclear inclusions.63
Papillary, seen both as the predominant morphology and in association with one of the conventional histologic subtypes; characterized by a striking papillary pattern with foci of necrosis, numerous mitotic figures, and local invasiveness.64
Since the 1993 WHO revision,62 characteristically aggressive hemangiopericytomas and hemangioblastomas have been deemed nonmeningothelial in origin and are no longer classified as meningiomas.
2007 WHO Classification59
Grade I: does not fulfill criteria for grades II (atypical) or III (anaplastic).
Grade II (atypical): Any of the following criteria:
Grade III (anaplastic): Either of the following criteria:

Most meningiomas are grade I. From a series of 581 consecutively resected patients from the Mayo Clinic, 81% were grade I and 19% grade II (data modified to reflect 2007 WHO classification).65 Grade III meningiomas are rare.
Proliferation indices based on MIB-1 labeling of Ki-67 nuclear protein or PC10 labeling of proliferating cell nuclear antigen (PCNA) are powerful adjuncts that offer additional prognostic information. High indices reflect increased proliferative activity and recurrence potential.66–71 Identification and quantitative measure of accumulation of chromosome change correlate highly with early tumor recurrence.53,72
At the time of this chapter’s preparation, the 2007 WHO classification has not yet been examined for prognostic utility in new radiotherapy studies. However, meningioma grade as defined by similar legacy systems,62,73,74 has been established of clear prognostic importance, with atypia and anaplasia associated with more frequent and earlier recurrence.73–76 For example, postradiotherapy 5-year progression-free survival (PFS) rates of 94% versus 78% for 1993 WHO Grade I versus Grade II (P = .02),75 95% versus 0% for 1993 WHO Grade I versus Grade II/III (P < .01),76 and 89% versus 49% for University of California, San Francisco (UCSF) system benign versus malignant (P = .001)73 have been reported.
Clinical Presentation and Diagnostic Evaluation
Evaluation begins with a detailed history and physical examination. Presenting complaints and physical findings, listed in order of frequency, are found in Tables 22-2 and 22-3, respectively. Patients with visual complaints should be evaluated with formal visual field and acuity testing before surgery or radiation therapy. Patients with hearing loss should be tested with formal audiometry.
Complaints | Incidence, % |
---|---|
Headache | 37 |
Personality change/confusion | 22 |
Paresis | 19 |
Generalized seizures | 19 |
Visual impairment | 16 |
Focal seizures | 15 |
Ataxia | 15 |
Aphasia | 10 |
Decreasing level of consciousness | 7 |
Paresthesia | 6 |
Diplopia | 3 |
Vertigo | 1 |
Decreased hearing | 1 |
Data from Rohringer, et al: Incidence and clinicopathologic features of meningioma, J Neurosurg 71:665, 1989.
Finding | Incidence, % |
---|---|
Paresis | 30 |
Nonfocal examination | 26 |
Memory deficit | 15 |
Cranial nerve deficit other than CN II | 12 |
Visual field deficit | 10 |
Paresthesia | 9 |
Aphasia | 9 |
Papilledema | 8 |
Decreased visual acuity | 6 |
Altered level of consciousness | 5 |
Nystagmus | 3 |
Decreased hearing | 2 |
Data from Rohringer, et al: Incidence and clinicopathologic features of meningioma, J Neurosurg 71:665, 1989.
Pediatric patients with convexity or parasagittal meningiomas often present with seizures, focal deficits, or signs of increased intracranial pressure (ICP), and those with intraventricular lesions typically present with elevated ICP, hemiparesis, and hemianopia. In addition to these typical presenting signs and symptoms, increasing head circumference as a first sign of meningioma is also seen in children.77
Adult patients with spinal meningioma most often present with pain (72%), which usually antedates other symptoms by months to years, followed by sensory loss and weakness, followed by bowel and bladder dysfunction.78 Children with spinal meningioma, unlike adults, most often present with extremity weakness (57%), followed by back pain, bowel and bladder dysfunction, and extremity pain. In children, back and extremity pain may be expressed as increased irritability.79
CT and magnetic resonance imaging (MRI) with contrast yield information regarding tumor size, location, potential resectability, and proximity to critical neurologic structures. Meningiomas are generally well circumscribed and smooth in contour. Most are homogeneously high density on unenhanced CT scans, 25% to 33% are isodense, about 1% are hypodense, and a few demonstrate mixed attenuation. The majority demonstrate moderate to intense homogeneous enhancement following administration of contrast, with the degree of enhancement dependent on tumor vascularity and extracellular accumulation of the contrast material. Peritumoral edema is seen in 60% of cases, and associated bony changes (destruction or hyperostosis) in 15% to 20%.80 The degree of preoperative peritumoral has been reported to correlate positively with probability of brain invasion81 and postoperative neurologic deficit.82
On MRI, meningiomas are typically isointense with gray matter on both T1– and T2-weighted images, and intensely enhanced when contrast is used. A radiographic feature that is suggestive of, but not specific for, meningioma is linear meningeal thickening and enhancement adjacent to a peripherally located cranial mass—the so-called “dural tail sign.”80,83 This has been reported in 60% of meningiomas, but can also been seen in chloroma, lymphoma, and sarcoidosis. The enhancement of the meningioma dural tail usually represents reactive changes and does not necessarily indicate neoplastic involvement.84–86 Intratumoral inhomogeneities are often seen on MRI, associated with calcifications, central necrosis, pseudocysts, and vascular spaces. Intratumoral hemorrhage is unusual. Displacement of the gray-white interface, peritumoral edema, and adjacent encephalomalacic changes are better seen with MRI than CT, whereas osseous changes and calcification are better seen with CT than MRI.80,87
Meningiomas also show high expression of the somatostatin-receptor subtype 2, enabling visualization with [68Ga]-DOTATOC-PET with very high meningioma to background ratios.88 Milker-Zabel et al have demonstrated complementary spatial information in a comparison of planning tumor volume (PTV) obtained with CT-MRI versus CT-MRI-[68Ga]-DOTATOC-PET, with the later smaller than CT-MRI in 35%, similar in 26%, and larger in 39%.89
Standard Therapeutic Approaches
Benign meningiomas are well managed with total excision if achievable with minimal morbidity, resulting in 5-, 10-, and 15-year PFS rates of 93%, 80%, and 75%, and 5-, 10-, and 15-year cause-specific survival (CSS) rates of 95%, 93%, and 88%, respectively.90 Approximately one in three meningiomas is not fully resectable,91 however, because of tumor location, size, and proximity to adjacent critical central nervous system (CNS) and vascular structures. In the skull base, total resection is accomplished about half the time91,92 and is less likely in the setting of previous irradiation, vessel encasement, multiple fossa involvement, and cranial nerve palsies.92 Although subtotal resection is an appropriate goal when decompression is expected to result in amelioration of symptoms, it is inadequate as a sole modality, with inferior 5-, 10-, and 15-year PFS of approximately 50%, 40%, and 30%, and CSS of 80%, 66%, and 50%,90 respectively.
Fractionated radiotherapy93 or single fraction radiosurgery,94 as primary management without surgery or after subtotal resection of benign meningioma, yields long-term PFS superior to subtotal resection and comparable to total excision, with low morbidity.
Atypical meningioma has an intermediate prognosis, with a 5-year PFS of 59% to 87%.65,95 Characteristics associated with higher likelihood of recurrence include subtotal resection,95,96 as well as brain invasion,65,95 high proliferative indices,97 numerous mitoses,98 and chromosomal change accumulation53,72,99 regardless of degree of resection. Adjuvant irradiation is recommended for subtotally resected or brain-invasive atypical meningioma, and should be considered for completely resected tumors with the above high-risk features (Fig. 22-1).100
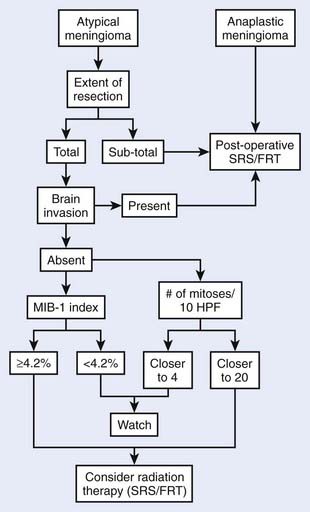
FIGURE 22-1 • The atypical and anaplastic meningioma treatment algorithm.
(From Modha A, Gutin PH: Diagnosis and treatment of atypical and anaplastic meningiomas: a review, Neurosurgery 57(3):538–550, 2005.)
Anaplastic meningiomas are likely to recur postoperatively, with a median PFS of 32 months and 5-year PFS rate of 29% to 44%.65,95 Adjuvant irradiation for anaplastic tumor is independently associated with an improved PFS rate95 and is recommended regardless of degree of resection.95,100
Fractionated Radiotherapy
Technique
Optimal radiation therapy requires meticulous attention to tumor volume as delineated by the neurosurgeon’s description as well as CT and MRI findings. MRI is superior to CT, with its exquisite resolution, absence of bone artifact, intense tumor contrast enhancement, and coronal and sagittal viewing advantages. In a comparison studied by Khoo and colleagues,87 however, MRI-defined meningioma volumes were larger but not inclusive of CT-defined volumes, suggesting complementary spatial information and a role for treatment planning with composite CT/MRI volumes. Milker-Zabel et al have also demonstrated complementary tumor imaging with CT/MRI and [68Ga]-DOTATOC-PET,89 suggesting composite use of all three modalities when available. Pre- and postoperative studies should be utilized in treatment planning when available. The PTV consists of the clinical tumor volume (CTV) plus a margin of 5 mm for conventional mask immobilization or 2 to 3 mm for stereotactic frame-based immobilization technique.75,101,102 For benign (WHO Grade I) meningioma, the CTV includes the composite gross tumor volume (GTV) in the setting of primary radiotherapy, and residual composite GTV in the adjuvant setting. After surgery, the CTV may be expanded beyond the remaining radiographic GTV to encompass microscopic residua based on the neurosurgeon’s description of intraoperative findings and an appreciation of the preoperative dural base. Because (1) the majority of benign meningioma recurrences following stereotactic fractionated radiotherapy75 are not marginal, and (2) microscopic involvement of the adjacent dura is typically confined to the first millimeter or two from the gross tumor,84–86 routine inclusion of the entire dural tail in the CTV has not been demonstrated to be necessary. Atypical and anaplastic meningioma also rarely fail marginally when treated with a CTV 10 mm beyond GTV, operative bed, hyperostotic bone, and dural thickening.103 For anaplastic tumors the CTV also includes peritumoral edema if present,103 or a rim of adjacent normal brain parenchyma if not.
Five-mm set-up error precision is achieved by use of an immobilization system, consisting of a head support, a thermoplastic mask that is molded to the patient’s head, and a frame that locks the mask and head in a reproducible position. Fused CT/MRI computerized treatment planning should be used, with treatment-planning scans obtained with the patient in the treatment position, wearing the immobilization device with radio-opaque fiducials. The use of such careful tumor localization and immobilization techniques has been shown to have greater prognostic importance than tumor size or radiotherapy dose, and has been associated with a 5-year PFS for benign meningioma which is significantly better (98% vs 77%, P = .002) than that achieved without such techniques.73 With the addition of a stereotactic frame and localization system, set-up error is reduced to 2 mm, allowing for a tighter PTV and less normal tissue irradiation.75,101
Optic nerve sheath meningiomas require precision conformal therapy, such as described by Eng and colleagues.104 The patient is positioned supine with the head rotated laterally, so that the affected optic nerve is approximately perpendicular to the horizontal plane. The appropriate angle of lateral head rotation can be estimated from diagnostic CT scans. In the series from Eng’s group, this value ranged from 15 to 27 degrees. Neck flexion should establish an imaginary vertical line on the lateral simulation film from the center of the orbit to a point just superior to the pituitary fossa. In this position, the patient’s head is immobilized with a head support, thermoplastic mask, and locking frame as just described. The treatment-planning CT scan is then obtained with the patient in this position, wearing the immobilization mask. Three small half-beam blocked fields are set up isocentrically with axes lying in the horizontal plane. Treatment table rotation is used to produce a vertex field and two superior-lateral fields. The superior-lateral fields are angled to avoid the contralateral eye and optic nerve. The beams are split perpendicular to the vertical axis and the anterior half is blocked in order to prevent anterior divergence toward the globe. Differential field weighting is used to optimize the isodose distribution, and the prescribed isodose volume (with 5% or less heterogeneity) should encompass the PTV. Stereotactic technique is particularly appropriate in this setting in order to minimize set-up error and PTV and maximize organ-at-risk (OAR) sparing.105,106
For other intracranial sites, multiple static fields should be selected to minimize dose to the uninvolved CNS tissues (Figs. 22-2 and 22-3). Opposed lateral fields should be avoided in practically all cases to prevent unnecessarily irradiating lateral CNS structures such as the temporal lobes. Intensity-modulated radiotherapy (IMRT), consisting of objective weighting, inverse planning, and fluence modulation, yields target coverage, target conformity, and OAR sparing superior to conventional radiotherapy, particularly for complex tumor volumes, at the expense of larger volumes of normal tissue receiving a low dose (Fig. 22-4).107,108 Careful conformal technique should limit inhomogeneity of dose to less than 10% (ideally less than 5%). No portion of the optic nerve or chiasm should receive greater than 54 Gy at 1.8 Gy per fraction.109 A dose of 54 Gy at 1.8 Gy per fraction for 5 days a week is generally given to benign (WHO Grade I) meningiomas,73,93 and for atypical and anaplastic (WHO Grade II and III) lesions, the dose is increased to 59.4 to 61.2 Gy103,110 Dose-response relationships have been demonstrated,73,103,111,112 with 54 Gy and 60 Gy conventionally fractionated yielding a significantly better PFS than lower dose schedules for benign112 and atypical or anaplastic103 meningiomas, respectively. Aggressive fractionated radiotherapy beyond 60 Gy is more toxic and has not been demonstrated to improve local control.110,113 Anaplastic lesions may be considered for radiosurgery boost following external beam radiotherapy, if the tumor volume is well defined and small.114
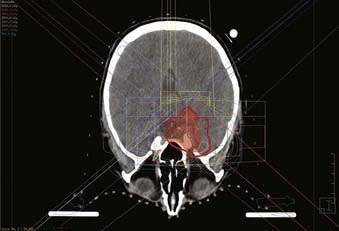
FIGURE 22-2 • Axial plane intensity-modulated radiation therapy isodose plan for right cavernous sinus meningioma.
Outcome
Fractionated radiotherapy as primary management without surgery75,93,115 or after subtotal resection of benign meningioma73,75,93 yields a 5-year PFS between 91% and 98%, which is superior to subtotal resection and comparable to total excision, and symptom improvement in 43% to 72% (Table 22-4). (Note that Table 22-4 excludes reports published before 1994, because those series did not use CT- and/or MRI-based targeting and dose planning, in contradistinction to all contemporary single- or multifraction radiation treatments of meningiomas.) Multivariate analyses demonstrate no relationship between PFS and degree of resection or tumor size.73,75,93,116
Mendenhall and colleagues93 treated 101 patients with skull base meningiomas between 1984 and 2001with fractionated radiotherapy alone (n = 66) or after subtotal resection (n = 35). The median dose was 54 Gy. After median follow-up of 5 years, 5-, 10-, and 15-year PFS rates were 95%, 92%, and 92%, respectively, and the corresponding CSS rates were 97%, 92%, and 92%, respectively, both independent of previous surgery.