Chapter 6 Mechanisms of Innate Immunity
• Innate immune responses do not depend on immune recognition by lymphocytes, but have co-evolved with and are functionally integrated with the adaptive elements of the immune system.
• The body’s responses to damage include inflammation, phagocytosis, and clearance of debris and pathogens, and remodeling and regeneration of tissues. Inflammation is a response that brings leukocytes and plasma molecules to sites of infection or tissue damage.
• The phased arrival of leukocytes in inflammation depends on chemokines and adhesion molecules expressed on the endothelium. Adhesion molecules fall into families that are structurally related. They include the cell adhesion molecules (CAMs) of the immunoglobulin supergene family (which interact with leukocyte integrins), and the selectins (which interact with carbohydrate ligands).
• Leukocyte migration to lymphoid tissues is also controlled by chemokines. Chemokines are a large group of signaling molecules that initiate chemotaxis and/or cellular activation. Most chemokines act on more than one receptor, and most receptors respond to more than one chemokine.
• Plasma enzyme systems modulate inflammation and tissue remodeling. The kinin system and mediators from mast cells including histamine contribute to the enhanced blood supply and increased vascular permeability at sites of inflammation.
• Pathogen-associated molecular patterns (PAMPs) or microbial-associated molecular patterns (MAMPs) are distinctive biological macromolecules that can be recognized by the innate immune system. Innate antimicrobial defenses include molecules of the collectin, ficolin, and pentraxin families, which can act as opsonins, either directly or by activating the complement system. Macrophages have cell-surface scavenger-receptors and lectin-like receptors, which allow them to directly bind to pathogens and cell debris.
• Toll like receptors (TLRs) are a family of receptors that recognize PAMPs from bacteria, viruses and fungi. They are present on many cell types, and can activate macrophages, using signaling systems that are closely related to those used by inflammatory cytokines TNFα and IL-1.
• Intracytoplasmic pattern recognition receptors (PRRS) recognize products of intracellular pathogens. Receptors of the Nod family recognize bacterial products, while the RLH receptors can recognize products of viral replication.
Innate immune responses
The adaptive immune responses depend on the recognition of antigen by lymphocytes, a cell type that has evolved relatively recently – lymphocytes are present in all vertebrates, but not invertebrates, although lymphocyte-like cells are present in closely related phyla, including the tunicates and echinoderms (Fig. 6.1).
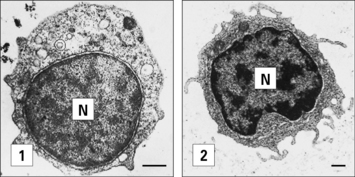
Fig. 6.1 Electron micrographs of lymphocyte-like cells
(Courtesy of Dr AF Rowley from Endeavour 1989:13;72–77. Copyright 1989 with permission from Elsevier.)
• displays the very primitive immune defense of phagocytosis; but also
• expresses MHC molecules and acts as an antigen-presenting cell, a function that makes sense only in relation to the evolution of T cells.
We can identify some of the ancient innate immune defense systems because related systems are seen in distant phyla. For example, the family of Toll-like receptors (TLRs, see Fig. 6.21) were first identified in insects. We can therefore infer that the distant ancestor of mammals and insects had a receptor molecule of this type that probably recognized microbial components.
Inflammation – a response to tissue damage
The body’s response to tissue damage depends on:
• killing of pathogens, neutralizing toxins, limiting pathogen spread;
• phagocytosis of debris, pathogens, and dead cells;
• proliferation and mobilization of fibroblasts or other tissue cells to contain an infection and/or repair damage;
• removal or dissolution of blood clots and remodeling of the components of the extracellular matrix;
• regeneration of cells of the tissue and re-establishing normal structure and function.
Inflammation brings leukocytes to sites of infection or tissue damage
Q. What three principal changes occur in the tissue during an acute inflammatory response?
A. An increased blood supply to the affected area, an increase in capillary permeability allowing larger serum molecules to enter the tissue and an increase in leukocyte migration into the tissue (see Chapter 1).
The cells seen in acute and chronic inflammation are quite different, and reflect the phased arrival of different populations of leukocytes into a site of infection (Fig. 6.2). Consequently:
• sites of acute inflammation tend to have higher numbers of neutrophils and activated helper T cells; whereas
• sites of chronic inflammation have a higher proportion of macrophages, cytotoxic T cells, and even B cells.
Cytokines control the movement of leukocytes into tissues
Tissue damage leads to the release of a number of inflammatory cytokines, either from:
The cytokines tumor necrosis factor-α (TNFα), IL-1 and interferon-γ (IFNγ) are particularly important in this respect. TNFα is produced primarily by macrophages and other mononuclear phagocytes and has many functions in the development of inflammation and the activation of other leukocytes (Fig. 6.3). Notably, TNFα induces the adhesion molecules and chemokines on the endothelium, which are required for the accumulation of leukocytes. TNFα and the related cytokines, the lymphotoxins, act on a family of receptors causing the activation of the transcription factor NF-κB (Fig. 6.4), which has been described as a master-switch of the immune system. NF-κB is, in fact, a group of related transcription factors, which can also be activated by Toll-like receptors and IL-1. The activation of vascular endothelium by TNFα or IL-1 causes chemokine production and adhesion molecules to be expressed on the endothelial surface.
Leukocytes migrate across the endothelium of microvessels
The routes that leukocytes take as they move around the body are determined by interactions between:
Leukocyte migration is controlled by signaling molecules, which are expressed on the surface of the endothelium, and occurs principally in venules (Fig. 6.5). There are three reasons for this:
• the signaling molecules and adhesion molecules that control migration are selectively expressed in venules;
• the hemodynamic shear force in the venules is relatively low, and this allows time for leukocytes to receive signals from the endothelium and allows adhesion molecules on the two cell types to interact effectively;
• the endothelial surface charge is lower in venules (Fig. 6.6).
Although the patterns of leukocyte migration are complex, the basic mechanism appears to be universal. The initial interactions are set out in a three-step model (Fig. 6.7):
• Step-1 leukocytes are slowed as they pass through a venule and roll on the surface of the endothelium before being halted – this is mediated primarily by adhesion molecules called selectins interacting with carbohydrates on glycoproteins;
• Step-2 the slowed leukocytes now have the opportunity to respond to signaling molecules held at the endothelial surface – particularly important is the large group of cytokines called chemokines, which activate particular populations of leukocytes expressing the appropriate chemokine receptors;
• Step-3 activation upregulates the affinity of the leukocytes’ integrins, which now engage the cellular adhesion molecules on the endothelium to cause firm adhesion and initiate a program of migration.
Transendothelial migration is an active process involving both leukocytes and endothelial cells (Fig. 6.8). Generally leukocytes migrate through the junctions between cells, but in specialized tissues such as the brain and thymus, where the endothelium is connected by continuous tight junctions, lymphocytes migrate across the endothelium in vacuoles, near the intercellular junctions, which do not break apart.
Selectins bind to carbohydrates to slow the circulating leukocytes
• E-selectin and P-selectin, which are expressed predominantly on endothelium and platelets; and
• L-selectin, which is expressed on some leukocytes (Fig. 6.9).
The carbohydrate ligands for the selectins may be associated with several different proteins:
• at sites of inflammation, E-selectin and P-selectin, which are induced on activated endothelium, bind to the sialyl Lewis-X carbohydrate associated with CD15, present on many leukocytes;
• some of the selectin ligands are selectively expressed on particular populations of leukocytes, for example the molecule PSGL-1 (P-selectin glycoprotein ligand) present on TH1 cells binds to E- and P-selectin, but a variant found on TH2 cells does not.
Chemokines and other chemotactic molecules trigger the tethered leukocytes
• one for their specific receptors; and
• a second for carbohydrate groups on proteoglycans (such as heparan sulfate), which allows them to attach to the luminal surface of endothelium (blood side), ready to trigger any tethered leukocytes (Fig. 6.10).
The chemokines may be produced by the endothelium itself. This depends on several factors including:
Chemokines receptors have promiscuous binding properties
• the receptors for the CXC chemokines are called CXCR1, CXCR2, and so on; while
• the receptors for the CC chemokines are called CCR1, CCR2, etc.
Originally most chemokines had a descriptive name and acronym such as macrophage chemotactic protein-1 (MCP-1). The current nomenclature describes them according to their type, hence MCP-1 is CCL2, meaning that it is a ligand for the CC family of chemokine receptors (Fig. 6.11).
The chemokine receptors are selectively expressed on particular populations of leukocytes (see Fig. 6.11) and this determines which cells can respond to signals coming from the tissues. The profile of chemokine receptors on a cell depends on its type and state of differentiation. For example:
• TH2 cells preferentially express CCR3; and
• TH1 cells preferentially express CCR5 and CXCR3. After activation in lymph nodes, the levels of CXCR3 on a T cell increase, so that it becomes more responsive for the IFNγ-inducible chemokines CXCL9, CXCL10, and CXCL11, which activate CXCR3. As a consequence, antigen-activated lymphocytes are more readily triggered to enter sites of inflammation where these chemokines are expressed.
Other molecules are also chemotactic for neutrophils and macrophages
Neutrophils and macrophages also have receptors for:
• C5a, which is a fragment of a complement component generated at sites of inflammation following complement activation; and
• LTB4 (leukotriene-B4), a product of arachidonic acid, which is generated at sites of inflammation, particularly by macrophages and mast cells.
• CXCL8 released by activated monocytes can induce neutrophil and basophil chemotaxis;
• similarly, macrophage activation leads to release of LTB4.
All of these chemotactic molecules act via 7tm receptors which activate trimeric G-proteins.
Integrins on the leukocytes bind to CAMs on the endothelium
Activation of leukocytes via their chemokine receptors initiates the next stage of migration.
In the third step of leukocyte migration (see Fig. 6.7), the leukocytes use their integrins to bind firmly to cell-adhesion molecules (CAMs) on the endothelium. Leukocyte activation promotes this step in three ways:
• it can cause integrins to be released from intracellular stores;
• it can cause clustering of integrins on the cell surface into high-avidity patches;
• most importantly, the cell activation induced by the chemokines causes the integrins to become associated with the cytoskeleton and can switch them into a high-affinity form (Fig. 6.12) – this is referred to as ‘inside-out signaling’ because activation inside the cell causes a change in the position and affinity of the extracellular portion of the integrin.
Many of the CAMs on the endothelium belong to the immunoglobulin superfamily. Some of them (e.g. ICAM-1 – intercellular adhesion molecule-1 and VCAM-1 – vascular cell adhesion molecule-1) are induced on endothelium at sites of inflammation, by inflammatory cytokines while others (e.g. ICAM-2) are constitutively expressed and not inducible (Fig. 6.13). Specific integrins bind to particular CAMs (Fig. 6.14), and since integrins vary between leukocytes and CAMs vary between endothelium in different tissues, the adhesion-step also affects which leukocytes will enter the tissue.
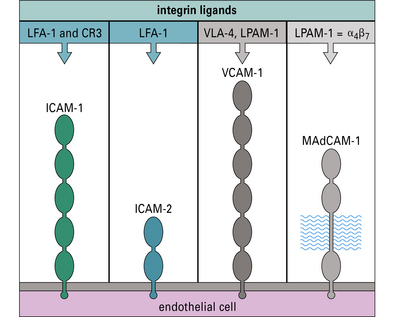
Fig. 6.14 Endothelial cell adhesion molecules
The molecules ICAM-1, ICAM-2, VCAM-1, and MAdCAM-1 are illustrated diagrammatically with their immunoglobulin-like domains. Their integrin ligands (see Fig. 6.w1) are listed above. MAdCAM-1 also has a heavily glycosylated segment, which binds L-selectin.
Integrins and CAMs – families of adhesion molecules
• the β1-integrins are involved in binding of cells to extracellular matrix;
• the β2-integrins are involved in leukocyte adhesion to endothelium or to other immune cells; and
• the β3-integrins (cytoadhesins) are involved in the interactions of platelets and neutrophils at inflammatory sites or sites of vascular damage.
However, there are several exceptions to this rule, and some α chains associate with more than one β chain (Fig. 6.w1).
• ICAM-1 and VCAM-1 are both induced by inflammatory cytokines;
• ICAM-2 is constitutively expressed at low levels on some endothelia and is downregulated by inflammatory cytokines.
MAdCAM-1 is a composite molecule that includes:
MAdCAM-1 was first identified on mucosal lymph node endothelium, but can also be induced at sites of chronic inflammation (Fig. 6.w2).
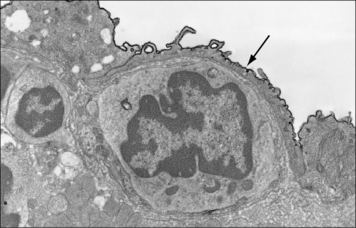
Fig. 6.w2 Mucosal addressin cell adhesion molecule of endothelium
(Reproduced from Immunology, O’Neill JK, Butter C 1991;72:520–525. Copyright 1991 with permission from Blackwell Publishing.)
Many of the integrins can bind to more than one ligand. For example:
• LFA-1 present on most lymphocytes binds to both intercellular CAM-1 (ICAM-1) and ICAM-2, which are expressed on endothelium;
• VLA-4 (α4β1-integrin) binds to vascular CAM-1 (VCAM-1) expressed on endothelium, or to fibronectin (an extracellular matrix component).
Leukocyte migration varies with the tissue and the inflammatory stimulus
• the state of activation of the lymphocytes or phagocytes – the expression of adhesion molecules and their functional affinity vary depending on the type of cell and whether it has been activated by antigen, cytokines, or cellular interactions. For example, activation of T cells induces both the chemokine receptor CXCR3, and the adhesion molecule LFA-1, which therefore promotes migration of activated cells into inflamed tissues.
• the types of adhesion molecule expressed by the vascular endothelium, which is related to its anatomical site and whether it has been activated by cytokines. For example: ICAM-1 is expressed at higher levels on brain endothelium than VCAM-1, whereas they are equally expressed on skin endothelium.
• the particular chemotactic molecules and cytokines present; receptors vary between leukocyte populations so that particular chemotactic agents act selectively.
Different chemokines cause different types of leukocyte to accumulate
In the second step of migration, neutrophils are triggered by chemokines such as CXCL8 synthesized by cells in the tissue, or by the endothelium itself. CXCL8 acts on two different chemokine receptors – CXCR1 and CXCR2 (see Fig. 6.11) – to initiate neutrophil migration.
• in the bronchi of individuals with asthma, CCL11 (eotaxin) is released, which causes the accumulation of eosinophils – CCL11 acts on CCR3, which is also present on TH2 cells and basophils, so by releasing one chemokine, the tissue can signal to three different kinds of cell to migrate into the tissue and this particular set of cells is characteristic of the cellular infiltrates in asthma;
• in sites of chronic inflammation, the chemokines CXCL10 and CCL2 are released by endothelium in response to IFNγ and TNFα – CXCL10 acts on activated TH1 cells (via CXCR3), while CCL2 acts on macrophages (via CCR5); consequently macrophages and TH1 cells tend to accumulate at sites of chronic inflammation.
Leukocyte migration to lymphoid tissues
Naive lymphocytes express L-selectin, which contributes to their attachment to carbohydrate ligands on HEVs in mucosal and peripheral lymph nodes. Once they have stopped on the HEV, migrating lymphocytes may use the integrin α4β7 (LPAM-1, see Fig. 6.14) to bind to MAdCAM on the HEV of mucosal lymph nodes or Peyer’s patches.
Chemokines are important in controlling cell traffic to lymphoid tissues
Once T cells have been activated they lose CXCR4 and CCR7, but gain new chemokine receptors (see Fig. 6.11), which allow them to respond to chemokines produced at sites of inflammation.
Naive B cells express CCR7 and CXCR5, a receptor for CXCL13, which is required for localization to lymphoid follicles within the lymph nodes. A subset of T cells, which are required to help B cell differentiation, also express CXCR5 causing them to colocalize with B cells in lymphoid follicles. Cells moving into lymphoid tissue therefore respond sequentially to signals on the endothelium and signals from the different areas within the tissue (Fig. 6.15).
Mediators of inflammation
• blood supply to the area increases;
• there is an increase in capillary permeability caused by retraction of the endothelial cells and increased vesicular transport across the endothelium – this permits larger molecules to traverse the endothelium than would ordinarily be capable of doing so, and so allows antibody and molecules of the plasma enzyme systems to reach the inflammatory site.
The kinin system generates powerful vasoactive mediators
Bradykinin is generated following the activation of Hageman factor (XII) of the blood clotting system, whereas tissue kallikrein is generated following activation of the plasmin system or by enzymes released from damaged tissues (Fig. 6.16).
The plasmin system is important in tissue remodeling and regeneration
The plasmin system can be activated by a soluble or a tissue-derived plasminogen activator, which leads to the enzymatic conversion of plasminogen into plasmin. Plasmin itself was originally identified by its ability to dissolve fibrin but it has several other activities, in particular it activates some matrix metalloproteases (MMPs), enzymes that are required for the breakdown and remodeling of collagen (Fig. 6.17). Additionally, it can promote angiogenesis (the formation of new blood vessels) by causing the release of cytokines that induce proliferation and migration of endothelial cells.
Mast cells, basophils and platelets release a variety of inflammatory mediators
Figure 6.18 lists the principal mediators of acute inflammation. The interaction of the immune system with complement and other inflammatory systems is shown in Figure 6.19.
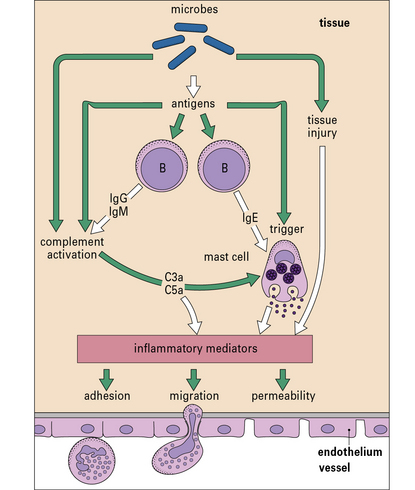
Fig. 6.19 The immune system in acute inflammation
The adaptive immune system modulates inflammatory processes via the complement system. Antigens (e.g. from microorganisms) stimulate B cells to produce antibodies including IgE, which binds to mast cells, while IgG and IgM activate complement. Complement can also be activated directly via the alternative pathway (see Fig. 4.4). When triggered by antigen, sensitized mast cells release their granule-associated mediators and eicosanoids (products of arachidonic acid metabolism, including prostaglandins and leukotrienes). In association with complement (which can also trigger mast cells via C3a and C5a), the mediators induce local inflammation, facilitating the arrival of leukocytes and more plasma enzyme system molecules.
Platelets may be activated by:
Activated platelets release mediators which are important in type II and type III hypersensitivity reactions (see Chapters 24 and 25).
Lymphocytes and monocytes release mediators that control the accumulation and activation of other cells
• activated macrophages release the chemokine CCL3 and leukotriene-B4, both of which are chemotactic and encourage further monocyte migration;
• lymphocytes can modulate later lymphocyte traffic by the release of chemokines and inflammatory cytokines, particularly IFNγ.
Chronic inflammation is characteristic of sites of persistent infection and occurs in autoimmune reactions where the antigen cannot ultimately be eradicated (see Chapter 20).
Pathogen-associated molecular patterns
There are three main types of PRR:
• secreted molecules, present in serum and body fluids;
• receptors, present on the cell surface and on endocytic vesicles; and
PRRs allow phagocytes to recognize pathogens
The binding of the pathogen to the phagocyte can be direct or indirect:
• direct recognition involves the surface receptors on the phagocyte directly recognizing surface molecules on the pathogen;
• indirect recognition involves the deposition of serum-derived molecules onto the pathogen surface and their subsequent binding to receptors on the phagocyte (i.e. the process of opsonization) (Fig. 6.20).
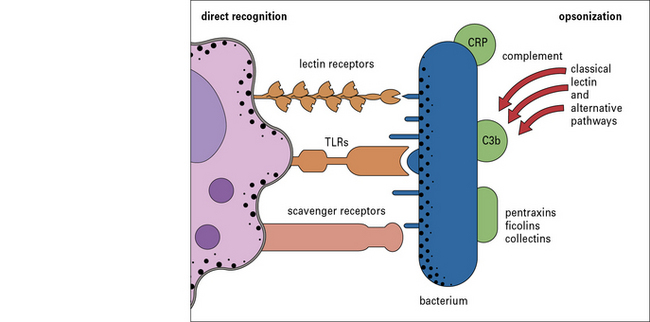
Fig. 6.20 The binding of pathogen to the macrophage can be direct or indirect
Macrophages can recognize PAMPs either directly or following opsonization with serum molecules.
Q. Give an example of an innate immune recognition system that allows macrophages to recognize and phagocytose bacteria
A. Complement C3b can become deposited on bacteria following activation of either the lectin pathway or the alternative pathway (see Fig. 4.4). The deposited C3b acts as an opsonin and is recognized by the receptors CR1, CR3, and CR4 on macrophages (see Fig. 4.12).
Soluble pattern recognition molecules
Pentraxins
The pentraxins are a family of molecules with a characteristic protein-fold, including C-reactive protein (CRP), serum amyloid-P (SAP), and pentraxin-3 (PTX3). In humans, CRP is the main acute-phase protein produced by liver hepatocytes in response to IL-6. It is a pentameric protein that binds to phosphorylcholine present on, for example, pneumococci and promotes their phagocytosis by binding to C1q and activating the complement classical pathway (see Fig. 4.4).
Collectins and ficolins opsonize pathogens and inhibit invasiveness
Collectins are a family of PRMs that bind to carbohyrates. Each member of the group has subunits formed of a triple-helical collagenous tail and a lectin head (Fig. 6.w3). A number of subunits may be linked together in the complete molecule and the overall structure of these molecules is similar to that of C1q (see Fig. 4.5). Although C1q is not itself a lectin, it probably evolved from this group as an Fc receptor, at the time that antibodies developed. In addition to Fc-binding, C1q recognizes pentraxins and some bacterial components. There are several cell receptors for C1q, so it can directly promote phagocytosis. Indeed, since much of the C1q in serum is not complexed with C1r and C1s, its direct action may be as important as its role in the classical pathway of complement.
Mannan-binding lectin (MBL), which activates the complement lectin pathway (see Fig. 4.4) is an acute phase protein, produced by the liver, which varies structurally between individuals, affecting their susceptibility to a number of infectious and autoimmune diseases.
Phagocytes have receptors that recognize pathogens directly
The scavenger receptors and carbohydrate receptors are primarily expressed on mononuclear phagocytes, and will be described more fully in Chapter 7.
Toll-like receptors activate phagocytes and inflammatory reactions
The family of TLRs include ten different receptors, in humans, many of which are capable of recognizing different microbial components (Fig. 6.21). All of the TLRs are present on phagocytic cells, and some are also expressed on dendritic cells, mast cells, and B cells. Indeed, most tissues of the body express at least one TLR.
Critical thinking: The role of adhesion molecules in T cell migration (see p. 434 for explanations)
Blocking antibody | Percentage of T cells migrating in 2 hours | |
---|---|---|
Unstimulated endothelium | IL-1-stimulated endothelium | |
none | 18 | 48 |
anti-ICAM-1 | 3 | 16 |
anti-VCAM-1 | 19 | 28 |
anti-αLβ2-integrin (LFA-1) | 2 | 14 |
anti-α4βrintegrin (VLA-4) | 17 | 32 |
1. Why does treatment of the endothelium with IL-1 cause an increase in the percentage of migrating cells in the absence of any blocking antibody?
2. Why does it require 24 hours of treatment with IL-1 to enhance the migration (1 hour of treatment does not produce this effect)?
3. Which adhesion molecules are important in mediating T cell migration across unstimulated endothelium?
4. Which adhesion molecules are important in mediating T cell migration across IL-1-activated endothelium?